Chapter 32 Applied Anatomy of the Thoracic and Lumbar Spine
Spine Alignment
The acquisition of upright posture and bipedal locomotion during human evolution imposed specific demands on the thoracic and lumbar spine. These demands are reflected in its overall structure (Fig. 32-1). Upright posture requires added load-bearing capacity; along the rostral-caudal axis, there is a substantial increase in the robustness not only of the vertebral bodies, but also of other stabilizing structures, such as the articular processes and spinous processes. Bipedalism requires spinal balance; this is reflected in normal spinal curvature. Unlike our quadruped ancestors, who possessed a single, broad spinal curvature, humans exhibit a combination of thoracic kyphosis and lumbar lordosis. These curves are formed by relatively small variations in intervertebral disc and vertebral body morphology. In the absence of spine deformity, the thoracic and lumbar curvatures are balanced, and the midportion of the C7 vertebral body sits atop the L5-S1 pivot point, located at the dorsal aspect of the L5-S1 intervertebral disc. With normal spinal balance, the degree of thoracic and lumbar curvature may vary considerably without adverse effect. However, deviations from normal sagittal alignment are mechanically unfavorable, imposing stress on axial musculature and resulting in pain and acceleration of the degenerative process.
Vertebrae and Ligaments
Vertebral Body
Sagittal vertebral morphology also changes along the rostral-caudal axis (Fig. 32-2). Thoracic vertebral bodies are wedge shaped, ventral height being shorter than dorsal height. This results in the normal thoracic kyphosis. In the lumbar spine, ventral and dorsal heights are generally comparable, and it is the disc shape, rather than vertebral body shape, that is the principal contributor to lumbar lordosis. At L4 and L5, some reverse wedging of the vertebral body may occur, with increased ventral height further contributing to the lower lumbar lordosis. The vertebral body gradually increases in cross-sectional area and height from the thoracic spine to the midlumbar spine (Figs. 32-3 and 32-4). From L2 to L5, vertebral height is usually stable and may decrease slightly. Changes in cross-sectional area are reflected in compression strength (Fig. 32-5).
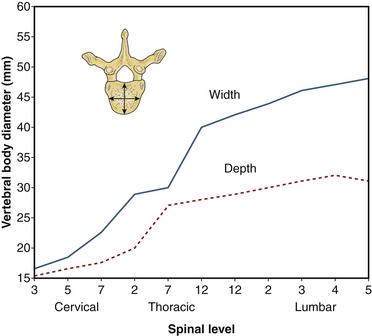
FIGURE 32-3 Vertebral body diameter by level.
(Benzel EC: Biomechanics of spine stabilization, New York, 2001, Thieme, p 1, reprinted with permission.)
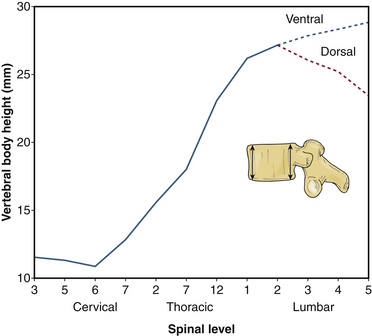
FIGURE 32-4 Vertebral body height by level.
(Benzel EC: Biomechanics of spine stabilization, New York, 2001, Thieme, p 1, reprinted with permission.)
Intervertebral Disc and Vertebral End Plate
The general function of the intervertebral disc is twofold: (1) It deforms to accommodate compressive loads, a role assumed by the nucleus pulposus, and (2) it resists tensile and torsional stresses, a role assumed by the anulus fibrosus (Fig. 32-6). At a microscopic level, the nucleus pulposus consists of a semifluid, gel-like substance embedded in a fine meshwork of fibrous strands. This structure results in a viscoelastic property that allows the disc to withstand and absorb axial stress. The anulus fibrosus has a lamellated boundary of intersecting fibrous strands. Anular fibers known as Sharpey fibers penetrate the dense cortical bone that makes up the outer ring of the vertebral end plate. The outermost fibers blend with overlying periosteum and longitudinal ligaments. Viewed in histologic cross section, the transition from nucleus pulposus to anulus fibrosus is a gradual one.
The bony vertebral end plate is a concave depression. At its central portion, the cancellous bone of the vertebral body is directly apposed to a cartilaginous plate, which fills the depression up to the level of the apophyseal ring, or marginal ring. The apophyseal ring is composed of cortical bone and is more resistant to compression failure than is the central end plate. Biomechanical studies have shown the strongest region of the lumbar end plate to be the dorsal, lateral aspect of the marginal ring, adjacent to the pedicle.1
Posterior Longitudinal Ligament
The posterior longitudinal ligament (PLL) also spans the full rostral-caudal axis of the spine but is less substantial than the ALL. It is located along the dorsal surface of the vertebral bodies, within the spinal canal (Fig. 32-7). At the midbody level, it is relatively narrow, but it widens considerably at the level of the disc before narrowing again as it transitions to the level below. It is adherent at the level of the end plate and anulus but elevated from the concave dorsal surface of the midvertebral body. Along the lateral margins of the PLL, there are often areas of adhesion with the underlying dura. Although the PLL’s contribution to spine stability is modest, it serves to direct disc herniations dorsolaterally, away from the central portion of the spinal canal.
Pedicle
Throughout the thoracic spine, the rostral surface of the pedicle is typically flush with the apophyseal ring of the superior end plate. In the upper and midthoracic regions, the caudal pedicle surface inserts at approximately the midplane of the vertebral body. The intervertebral foramen at these levels is formed almost exclusively by the inferior vertebral notch; the superior vertebral notch is small or nonexistent. By the lower thoracic spine, a relative increase in pedicle height has resulted in the caudal pedicle surface inserting somewhat lower, in plane with the lower one third of the vertebral body. In the lumbar spine, the pedicle is positioned progressively lower on the vertebral body and the superior vertebral notch is more pronounced.
Transverse pedicle width, rather than pedicle height, is the dimension that limits the size of a pedicle screw that can be placed at a given level. In the thoracic spine, pedicle height is commonly double that of pedicle width. Pedicle width is smallest in the region of T4-6, but increases only minimally until one arrives at the thoracolumbar junction. A graphical depiction of changes in these two dimensions across the neuraxis is shown in Figures 32-8 and 32-9.
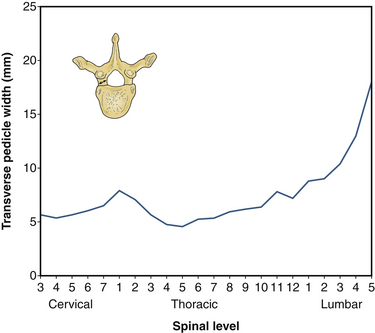
FIGURE 32-8 Transverse pedicle width by level.
(Benzel EC: Biomechanics of spine stabilization, New York, 2001, Thieme, p 6, reprinted with permission.)
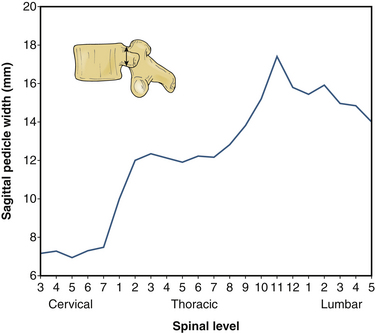
FIGURE 32-9 Sagittal pedicle width by level.
(Benzel EC: Biomechanics of spine stabilization, New York, 2001, Thieme, p 6, reprinted with permission.)
For the same reason that pedicle width constrains screw placement more than pedicle height does, transverse pedicle angle is a more relevant anatomic variable than is sagittal angle in the placement of thoracic pedicle screws. If a screw’s medial-lateral trajectory differs from that of the pedicle by even a relatively small amount, medial or lateral breach may result. Transverse pedicle angle declines fairly steadily as one proceeds down the thoracic spine until a nearly “straight-ahead” trajectory is encountered in the lower thoracic vertebrae; it then increases steeply across the lumbar levels, such that the L5 pedicle has a transverse angle of 25 to 30 degrees (Fig. 32-10).
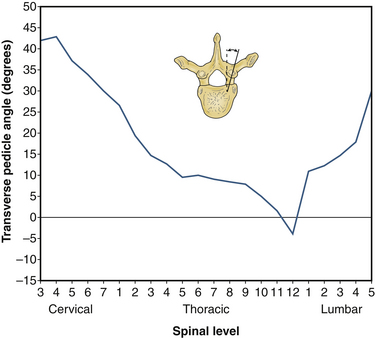
FIGURE 32-10 Transverse pedicle angle by level.
(Benzel EC: Biomechanics of spine stabilization, New York, 2001, Thieme, p 6, reprinted with permission.)
Transpedicular instrumentation in the thoracic spine is more challenging than that in the lumbar spine because of the presence of the adjacent spinal cord, the smaller pedicle diameter, and the relative proximity to neural structures (see Figs. 32-8, 32-9, and 32-14). In the lumbar spine, there is approximately 1.5 mm of epidural space between the medial pedicle wall and the thecal sac.2 In the thoracic spine, the medial pedicle border is contiguous with the edge of the thecal sac.3 In the lumbar spine, the distance from the upper edge of the pedicle to the nerve root above is approximately 5 mm, and the distance from the lower edge of the pedicle to the nerve root below, approximately 1.5 mm.2 In the thoracic spine, the distance from the upper edge of the pedicle to the nerve root above is approximately 2 to 4 mm and the distance from the lower edge of the thoracic pedicle to the nerve root below is approximately 2 to 3 mm.3
Facet and Pars Interarticularis
The facet joint, or zygapophyseal joint, is formed by an inferior articulating process emanating from the level above and a superior articulating process emanating from the level below. The facet joint is a synovial joint, possessing a true joint capsule. This capsule has two layers: an outer layer, composed of parallel bundles of collagenous fibers, and an inner elastic layer, similar in composition to the ligamentum flavum.4 Gliding articulation at the facet joint is limited by the capsular fibers, which are relatively lax in the cervical region but increase in tautness along the rostral caudal axis.
The facet joint functions primarily as a motion-limiting structure; only in extension does it function in an axial load-bearing capacity. The way in which a facet joint constrains motion depends on the alignment of its articulating surfaces (see Fig. 32-2). Lumbar facets occupy a plane that is intermediate between the sagittal and coronal planes. In the lumbar spine, there is a progression from relatively sagittal (25 degrees from sagittal) at L1-2 to relatively coronal (50 degrees from sagittal) at L5-S1. To the surgeon approaching the dorsal lumbar spine, the entry into the facet joint will be found progressively more laterally as one descends toward the lumbosacral junction. This more coronal orientation at the lumbosacral junction is an important element in preventing spondylolisthesis. Thoracic facets have an alignment that is oblique to all three cardinal planes; the articulating surface faces dorsally and slightly superolaterally. Thus, the superior articulating process is positioned relatively medial to the inferior articulating process; this is in contrast to the lumbar spine, where the superior articulating process occupies a lateral position. The transition from thoracic facet orientation to upper lumbar orientation occurs abruptly at the thoracolumbar junction.
Transverse Process
The transverse processes of the thoracic spine and the lumbar spine are relatively thin, consisting of both cortical and cancellous bone. In the thoracic spine, the transverse process projects dorsolaterally and articulates at its tip with the like-numbered rib. In the lumbar spine, the transverse processes project straight laterally and are easily fractured during wide dorsal exposure for dorsolateral fusion. The transverse processes of T11 and T12 are hypoplastic relative to their neighbors and do not articulate with their corresponding ribs. Commonly, the T11 transverse process is a dorsolaterally directed bony stump. In lieu of a T12 transverse process, there are only three small tubercles: a superior tubercle, which is equivalent to the lumbar mammillary process; an inferior tubercle, which is equivalent to the lumbar accessory process; and a lateral tubercle, which represents a very small equivalent of a transverse process. Variation in transverse process morphology can be seen in Figure 32-2.
Rib Articulations of the Thoracic Spine
The relationship of each rib to its corresponding vertebral body changes along the rostral-caudal axis of the thoracic spine (see Fig. 32-1). The 1st rib articulates exclusively with T1 via a single, complete facet located at the lateral aspect of the vertebral body. The 2nd through 9th ribs articulate with their like-numbered vertebrae, as well as the vertebra below, via paired demifacets. These demifacets are also located on the lateral aspect of the vertebral body but in a progressively dorsal position. By the lower thoracic spine (T10-12), two important changes have occurred, First, the pattern of articulation has returned to that of a single, complete, unpaired facet; second, the gradual dorsal movement of the articulation has resulted in the joint being located on the lateral surface of the pedicle, not the vertebral body. However, the size of the rib head and its joint capsule are such that ventrolateral access to the disc space or neural foramen inevitably requires removal of some or all of the adjacent rib head, regardless of level (Fig. 32-11). Because the parietal pleura obscures much of the relevant anatomy, palpation of the rib head is an important orienting maneuver during transthoracic approaches to the spine; often the only recognizable structure that is visible through the pleura is the sympathetic chain, coursing over the rib heads (Fig. 32-12).
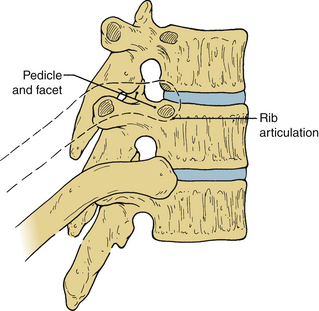
FIGURE 32-11 Rib removal is required for access to the thoracic neural foramen and intervertebral disc.
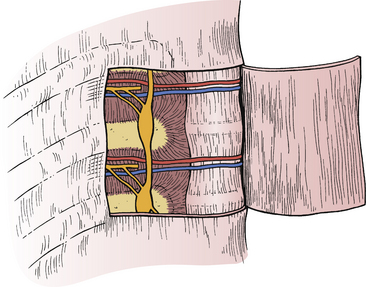
FIGURE 32-12 Lateral view of thoracic spine with segmental vessels and sympathetic chain located deep to the parietal pleura.
Lamina and Spinous Process
In general, the laminae are arranged in a shingle-like array, in which the lamina above overlaps the one below (see Fig. 32-1). During laminectomy, the deeper position of the more rostral portion of the lamina is readily appreciated. In the thoracic spine and the lumbar spine, the pars interarticularis consists of the lateralmost portion of the lamina. This is the portion of lamina that resides between, and functionally connects, the superior articulating process and the inferior articulating process. The pars interarticularis is important for spine stability; without it, the stabilizing effect of the facet joint below is lost.
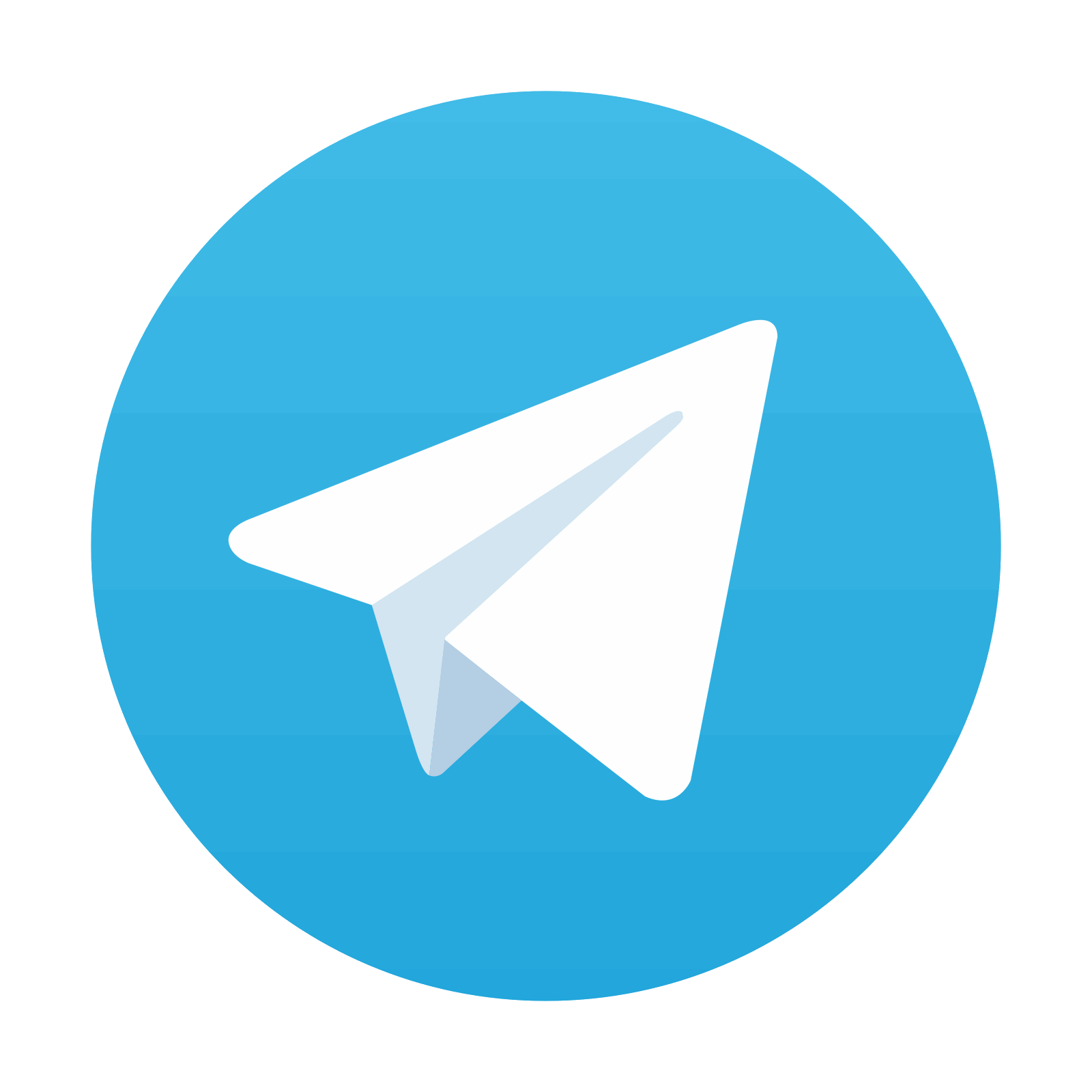
Stay updated, free articles. Join our Telegram channel

Full access? Get Clinical Tree
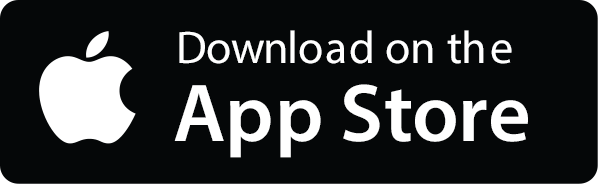
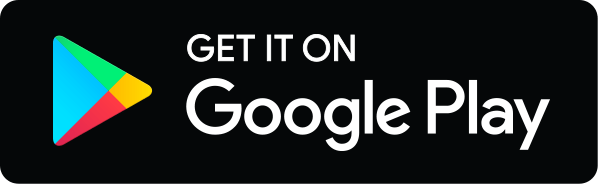