1 Pharmacology of Botulinum Neurotoxins
Summary
Botulinum neurotoxin (BoNT) is the most potent toxin, which acts to block the release of the neurotransmitter acetyl choline as well as other neurotransmitters such as calcitonin gene-related peptide, substance P, and glutamate. BoNT has been approved as a safe and effective therapeutic option for the management of many motor, sensory, and autonomic conditions. This chapter will review the biochemistry and mechanism of action of the neurotoxins, the mechanism of action, the pharmacokinetics, and the multiple clinical applications possible with these neurotoxins.
1.1 Introduction
Botulinum neurotoxin (BoNT) has been approved as a safe and effective therapeutic option for several clinical conditions. The common basis underlying all of the conditions amenable to treatment with BoNT is overactivity of neurotransmitter or neuropeptide release. The clinical efficacy of BoNT is based on the localized and highly specific, but reversible, disruption of the exocytotic mechanism within neurons, resulting in temporary inhibition of the release of neurotransmitters after injection into specific muscles or other tissues.
1.2 Subtypes of Botulinum Neurotoxin
Botulinum neurotoxins are synthesized by a variety of clostridial species, most notably Clostridium botulinum, but also including C. baratii (BoNT-F) and C. butyricum (BoNT-E), and C. argentinese (BoNTG). 1 Recently, non-Clostridial bacteria, Weisella oryzae, was also found to produce BoNT-A; however, further investigations are needed to define the biological role of this novel bacterial toxin. 1
These bacteria synthesize several immunologically distinct neurotoxins classified as serotypes A through G with over 40 subtypes. A numerical notation has been introduced such that the subtypes are designated, BoNT-A1 and BoNT-A2 for example. 2 Epidemiologic studies and in vitro characterization show that only serotypes A, B, E, and F cause human botulism, whereas serotypes C and D are prevalent in bird and cattle botulisms. 3 Furthermore, three hybrid mosaic types have been described, including BoNT-CD, BoNT-DC, and BoNT-HA. 4 The latter was originally termed “BoNT-H” but was later renamed based on amino acid and genomic sequence analysis, this being the first new BoNT serotype to be described in over 40 years.
1.3 Biochemistry of Botulinum Neurotoxin: Toxin Structure
The biologic activity of the neurotoxin is contained within a 150-kd protein commonly referred to as the core neurotoxin. In nature, a single 150-kd neurotoxin is incorporated into a molecular complex that varies in size based on the number of associated nontoxic proteins, which are classified as hemagglutinins or nonhemagglutinins. 5 The largest neurotoxin complex is 900-kd, formed only by the A serotype. Type A contains seven subtypes termed A1-A7 based on sequencing previously been described. 6 It remains unclear how these amino acid differences affect the subtype’s biologic activity and only a few differences in characteristics have been elucidated. BoNT-A2 has been shown to enter cells faster than BoNT-A1, 7 to more potently inhibit the grip strength in rats after local administration, and to be more potent in the hemidiaphragm assay. 8 , 9
Serotypes A, B, C1, and hemagglutinin-positive D are in the form of 500-kd and 300-kd complexes, whereas serotypes E, F, and hemagglutinin-negative D form only 300-kd complexes. 10 The neurotoxin complex is known to stabilize and protect the core neurotoxin against thermal and pH stress in addition to shielding the neurotoxin from enzymatic degradation. 11 , 12 , 13
The core neurotoxin is synthesized as a 150-kd single-chain protein that must be cleaved or “nicked” by proteases to exert its activity. 14 Cleavage produces a di-chain molecule consisting of a 100-kd C-terminal heavy chain and a 50-kd N-terminal light chain metalloprotease that are held together by a disulfide bond. The C-terminal heavy chain can be divided into two domains: the N-terminal portion weighing 50 kDa is the translocation domain required for release into the cytosol and the C-terminal part is the receptor-binding domain that allows for the specific binding and endocytosis of BoNT into motor neurons (Fig. 1‑1). 4

The tertiary structure of the di-chain protein is conserved through all clostridial neurotoxins; however, there is considerable heterogeneity between the protein sequences of the different serotypes (reportedly up to 70%), accounting for their different neuronal affinities, antigenicity, and intracellular targets. 15
1.4 Mechanism of Action
In the treatment of skeletal muscle overactivity, BoNTs inhibit acetylcholine release at the neuromuscular junction, thereby reducing excessive muscle contractions. This inhibition of calcium-dependent vesicular neurotransmitter release occurs via a multiple-step process. In the first step, the neurotoxin must dissociate from its protective protein shield to allow binding of the free neurotoxin to the neuronal surface. The kinetics of dissociation in vivo are currently unknown, but the stability of the neurotoxin complex is known to be affected by basic pH and increasing ionic strength of the solute. 16 , 17
The binding of the free BoNT to the neuron is accomplished through the interaction of the heavy chain of the 150-kd neurotoxin with neuronal receptors that are located predominantly, but not exclusively, on cholinergic nerve terminals. For BoNT-A (the most commonly used in clinical practice), a two-receptor mechanism has been proposed: the neurotoxin first interacts with a cell-surface resident ganglioside that serves to retain the neurotoxin close to the plasma membrane, facilitating the binding of the neurotoxin with its cognate protein receptor. The protein receptor for type A, E, and F neurotoxins has been identified as SV2, a constituent protein of the synaptic vesicle. 18 , 19 , 20 , 21 The neurotoxin is only able to bind to SV2 when the protein is exposed on the cell surface during neurotransmitter release. Thus, the neurotoxin preferentially enters neurons that are actively secreting neurotransmitter or neuropeptides. 19 The receptor molecules for serotypes A and B are distinct, with the protein receptor for types B and G identified as another synaptic vesicle protein known as synaptotagmin. 20 Other serotypes may also bind to unique sites, but they have not been characterized at this time.
After binding, BoNTs are internalized into the neuron via receptor-mediated endocytosis. 22 The neurotoxin undergoes a conformational change inside the acidified endocytotic vesicles, allowing the translocation domain to interact with the membrane of the endocytotic vesicle, providing a portal to enable the light chain to traverse the wall of the endosome. 23 The low pH in the endocytotic vesicle also reduces the disulfide bond, which releases the light chain from the heavy chain, freeing it to enter the neuronal cytosol. 24 Once inside the cytosol, the light chain, which contains a zinc-dependent endopeptidase, disrupts one or more of the SNARE (soluble N-ethylmaleimide-fusion-protein attachment protein receptor complex) proteins necessary for vesicle docking and fusion, thereby reducing exocytotic neurotransmitter release (Fig. 1‑2). 25

Impairing the function of the SNARE complex causes inhibition of neurotransmission, leading to flaccid paralysis and potentially death. Each serotype cleaves a specific peptide bond on one or more of these proteins. Type A neurotoxin cleaves the membrane-associated SNARE protein SNAP-25 (synaptosomal-associated membrane protein weighing 25 kDa), whereas type B neurotoxin cleaves the vesicular-associated protein VAMP (synaptobrevin). It has been recently proposed that modulation of the interstitial zinc concentration might alter the clinical efficacy of BoNT activity; however, this concept remains unexplored currently. Of note, BoNT-HA cleaves VAMP-2, or synaptobrevin, which is identical to the behavior of BoNT-FA. 4
1.5 Pharmacokinetics of Botulinum Neurotoxin
The relative duration of inhibition of neurotransmitter release varies among serotypes, presumably based on the half-life of the light-chain 26 and the time it has taken for the neuron to restore intact SNARE proteins (Fig. 1‑3). In a preclinical model, the duration of effect was the longest for type A, followed by types C1, B, F, and E. 27 Following injection in human muscles, serotypes A and C1 appear to have the longest duration of effect. 28

The recovery of neuronal activity following BoNT-induced denervation has been examined in several preclinical models. 29 , 30 , 31 Early studies showed that axonal sprouts developed from the affected nerves, likely in response to growth factor secretion from the denervated muscle. These sprouts are active and produce temporary reinnervation during the early recovery phase. The exact duration of the pharmacologic action of BoNT in neurons is not known; however, during the later phase of recovery, vesicular release has been found to return at the original terminal, a process that is accompanied by retraction of the neuronal sprouts. This suggests a process of sprouting that is associated with reestablishment of activity over time in the originally affected nerve terminal.
The inhibition of neurotransmitter release by BoNTs is reversible, which has both benefits and limitations in clinical use. The benefits include the flexibility to inject a given muscle based on its activity level, which may fluctuate over time, the avoidance of permanent interventions such as surgery, and the resolution of any unintended effects of BoNT treatment. The primary limitation associated with the reversibility of the effects of BoNT is the need for repeated injections; however, repeat treatment can result in sustained efficacy. As most conditions treated with BoNT are chronic in nature, a longer duration of effect is a desirable property and was the rationale for the development of type A over other serotypes of BoNT for clinical use.
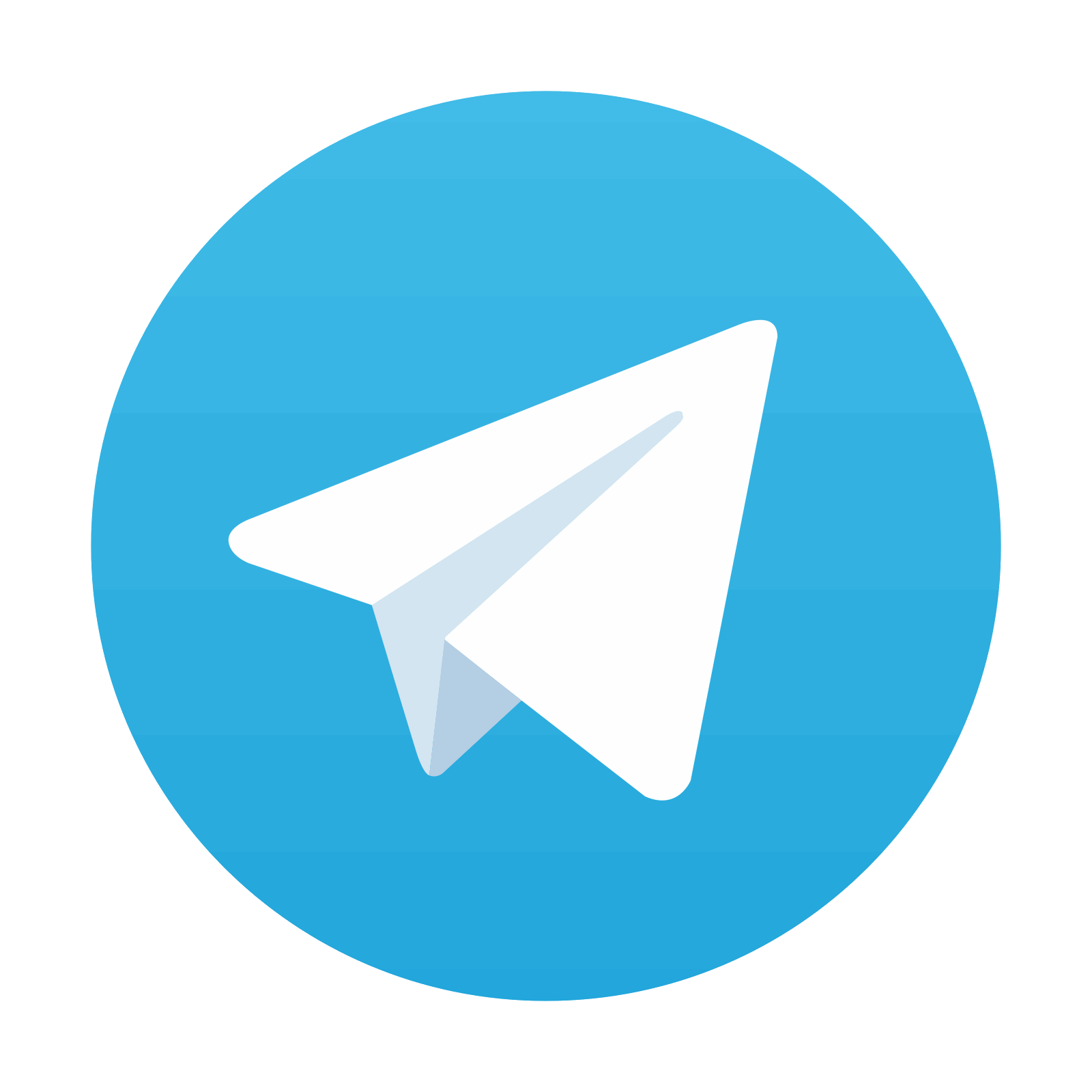
Stay updated, free articles. Join our Telegram channel

Full access? Get Clinical Tree
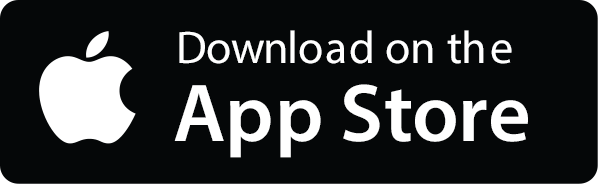
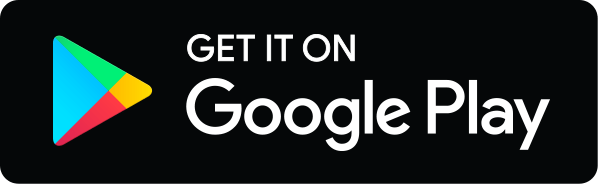