10 Application of PET and SPECT in Pediatric Epilepsy Surgery
Epilepsy is the most common neurological disorder, with a prevalence of 1 to 2% and cumulative lifetime incidence exceeding 3%. Almost 25% of epileptic patients do not respond to multiple antiepileptic treatments and will have intractable (i.e., medically refractory) seizures. These patients can be helped by surgically removing the epileptogenic region of the cerebral cortex. However, to accomplish this, the epileptogenic region has to be precisely delineated before surgery. Indeed, the most important aspect of presurgical evaluation is to identify the discrete epileptogenic region that can be resected without causing an unacceptable loss of neurological function and that will lead to complete seizure control. Functional neuroimaging, such as positron emission tomography (PET) and single photon emission computed tomography (SPECT), combined with electroencephalography (EEG), can play a very important role by providing noninvasive presurgical localization of epileptogenic foci in the patient with no brain lesion on computed tomography (CT) or magnetic resonance imaging (MRI; i.e., nonlesional cases), with multiple structural lesions of which only one or two are epileptogenic, or in cases with discordant or inconclusive EEG findings. PET and SPECT can be very useful in cases by identifying the epileptogenic regions.
Rationale for PET and SPECT
SPECT and PET are imaging techniques that use radioisotopes or radiolabeled molecules (the term radiotracer will be used for both of them) to study the perfusion or function of an organ, even at the cellular or molecular level. With both methods, a very small amount of a selected radiotracer is injected into the patient. The radiotracer is selected on the basis of desired purpose, that is, which organs and what functions the physician is interested in exploring. The gamma rays emitted by the radiotracers are detected externally with the help of suitable detectors, and an image of the spatial distribution of these radiotracers is generated. Therefore, theoretically, any organ or its function can be studied, provided appropriate radiotracers are available. The reason for applying PET and SPECT in epilepsy is based on the fact that the metabolism (particularly of glucose), receptor density and neurotransmission, and cerebral blood flow in the epileptic region (as well as in the associated seizure propagation network) are altered and can be detected by these imaging techniques.
Principle and Techniques of PET and SPECT
PET
PET is an imaging technique used to noninvasively image and measure the function of various organs. In PET, positronemitting radionuclides, such as 18F, 11C, 15O, and 13N, are used to label various natural biological substrates and drugs or pharmaceuticals. These all contain hydrogen, carbon, oxygen, or nitrogen, which can be replaced with their radioactive positronemitting counterpart. The resulting radioactive substance, also known as a radiotracer or PET tracer, will have similar behavior and will follow the same physiological pathways by emitting paired high-energy (511 Kev) photons. These photons can be detected by external detectors, and the whole process can be traced or imaged. Any physiological or metabolic process, such as glucose metabolism, protein synthesis, enzymatic processes, or receptor–ligand interaction, can be studied using an appropriate radiotracer and various kinetic models.1
The most commonly used PET tracer in epilepsy is 2-deoxy-2[18F]fluoro-D-glucose (FDG; half-life: 110 minutes), which measures glucose metabolism. FDG is transported in tissue and phosphorylated to FDG-6-phosphate in the same manner as glucose. However, FDG-6-phosphate is not a substrate for the next step of glycolysis. Because it cannot immediately leave the cell, phosphorylated FDG gets trapped within the cell, and its location and quantity can be measured by PET. Under steady-state conditions, FDG uptake reflects the glucose metabolic rate. In the brain, this rate is highly related to the synaptic density and functional activity of the brain tissue.
Because brain glucose metabolism undergoes age-related changes, particularly in the early childhood, practitioners should be aware of this pattern while interpreting pediatric FDG PET scans. Metabolic rates of glucose in cerebral cortex at the time of birth are usually approximately 30% less than those of adult values. They reach adult values by the second year of life, exceeding them by the third year, and reach the peak values (almost double the adult values) by 3 to 4 years of age, when a plateau is reached. This plateau persists until approximately 10 years of age, then gradually the glucose metabolic rates decline to reach adult values by the age of approximately 16 to 18 years. The glucose metabolism pattern and visual appearance on PET scans also changes with age. In the newborn, glucose metabolic activity is most prominent in primary sensory and motor cortex, thalamus, brain stem and cerebellar vermis, cingulate cortex, amygdala, and hippocampus. Between ages 2 and 4 months, glucose use increases in parietal, temporal, and primary visual cortex (medial occipital or calcarine cortex), as well as in the basal ganglia and cerebellar hemispheres. Between 6 and 8 months of age, glucose use increases in the lateral and inferior prefrontal regions, with medial and dorsal frontal cortex becoming active between 8 and 12 months of age. The adult pattern is seen by 1 year of age.2,3
Interictal FDG PET typically shows reduced radiotracer uptake (hypometabolism) in the epileptogenic region ( Fig. 10.1 ). The hypometabolism can result from a variety of mechanisms, including neuronal loss, diaschisis, or reduction in synaptic density. It appears that cortical hypometabolism may be associated with duration, frequency, and severity of the seizures, hypometabolism is usually found in only one fourth of children with new onset epilepsy compared with 80 to 85% of adults with intractable seizures.4 Persistent or increased seizure frequency may lead to enlargement of the hypometabolic area, whereas seizure control may be associated with decrease in the size of hypometabolic cortex or even its resolution.5 The time interval between PET acquisition and the most recent seizure also may affect the extent and severity of the cortical abnormality, with shorter duration having a positive effect on the extent and severity of the hypometabolism.6
During the ictal phase, metabolism increases many fold in the epileptic region; however, because FDG PET shows cumulative FDG uptake over a period of 30 to 45 minutes, the final images can be variable and complex, depending on the exact nature of the underlying pathology, seizure duration, seizure evolution, and net summation effects of ictal, postictal, and interictal metabolism. Therefore, ictal FDG PET is not very reliable and is often difficult to interpret, and EEG monitoring should be performed during the FDG uptake period to rule out any clinical or subclinical seizure. Because ictal FDG PET is virtually not done, in all the subsequent discussion, we use the term FDG PET in place of interictal FDG PET.
Because FDG PET usually shows a larger area of hypometabolism extending beyond the epileptogenic region, it cannot be reliably used to precisely determine the surgical margin. However, it can be used for lateralization and general localization of the seizure focus. Further, this information can help in making an a priori hypothesis about subsequent subdural electrode placement, which may be very useful, particularly in cases of normal MRI ( Fig. 10.2A ). However, use of more specific tracers, as discussed here, can help in providing a more precise delineation of the epileptogenic tissue; this becomes particularly important in a developing pediatric brain and when the epileptic focus potentially involves eloquent brain regions (primary motor, speech, or visual areas).
Other PET tracers with the potential for detecting epileptic brain regions include 11C-flumazenil (FMZ), which binds to α subunits of the GABAA-benzodiazepine receptor and 11C-alphamethyl-L-tryptophan (AMT), which measures tryptophan metabolism. FMZ binding or FMZ VD (volume of distribution) is high at 2 years of age and then decreases exponentially with age until adult values are reached at approximately age 20 years. The order of brain region (from highest to lowest FMZ binding) at 2 years of age is as follows: primary visual cortex, superior frontal cortex, medial temporal cortex, temporal lobe, prefrontal cortex, cerebellum, basal ganglia, and thalamus. FMZ binding in medial temporal lobe is robust enough for its good visualization (compared with FDG PET, in which the medial temporal region, particularly hippocampus, is often not well visualized). This is one of the main reasons for an important role of FMZ PET in epileptic patients in whom medial temporal lobe abnormalities are suspected. AMT is an analog of tryptophan, the precursor for serotonin synthesis, but, unlike tryptophan, AMT is not incorporated into protein in significant amounts. AMT is converted to α-methyl-serotonin (AM-5HT) by tryptophan hydroxylase, and it accumulates in neurons and nerve terminals along with the releasable pool of serotonin. After intravenous injection, AMT accumulates in the brain for the first 20 minutes (less than 2% of the injected dose is present in the brain at peak values), after which a plateau is reached and maintained for up to 60 minutes, with no right–left asymmetry in normal subjects.


SPECT
In SPECT, rotating γ cameras (detectors) are used to image the distribution of the injected radiotracer in the organ of interest. For this purpose, 1 to 3 detectors and only γ ray emitting radioisotopes are used. For the purpose of brain and particularly for epilepsy, SPECT is mostly used to study brain perfusion, with hexamethyl propylene amine oxime (HMPAO) and ethylene cysteine dimer (ECD) labeled with 99mTc being the most common radiotracers used for this purpose. HMPAO readily crosses the blood–brain barrier and approximately 80% is extracted by brain during the first pass. Once inside the neurons and glial cells, HMPAO gets trapped because it is oxidized by glutathione into a nondiffusible compound. A total of 4 to 7% of the injected activity is trapped within the brain, reaching its peak in 1 to 2 minutes. ECD is another lipophilic compound that, like HMPAO, gets trapped inside neurons because of its transformation into a hydrophilic compound that cannot diffuse back. Its first pass extraction is 60 to 70%, with a maximum of 6 to 7% injected activity accumulating in the brain 1 to 2 minutes after the injection. The brain can be imaged subsequently, and the resulting image provides a snapshot of perfusion immediately after the injection. This is the basis for applying brain SPECT in epilepsy, because the ictal and interictal phases are usually associated with increased or decreased blood flow, respectively, in the epileptic foci. However, during seizure, the cerebral blood flow changes rapidly with time, depending on the seizure type and its mode of propagation. Therefore, early radiotracer injection is imperative to catch the blood flow changes in the epileptic zone, during seizure. Similarly, knowledge of the exact time of injection (because it takes approximately 20 to 30 seconds for the radiotracer to reach the brain from an arm vein) and seizure duration is very important for the correct interpretation of the SPECT images. Delayed injection of radiotracer may show a variable pattern of blood flow changes associated with seizure evolution in the epileptic zone and mode and pattern of seizure propagation, depending on the time of injection. Also, because seizure propagation is usually from the temporal to the frontal lobe or from posterior (parietooccipital lobes) to anterior cortical regions (temporal and frontal lobe),7–10 interpretation of ictal SPECT can be very challenging if the exact timing of radiotracer injection and seizure onset is not known, unless injection is synchronized with video-EEG monitoring.
During the ictal phase, blood flow in the epileptic region can increase up to 300%, which can be seen as an area of hyperperfusion in ictal SPECT.11 A true ictal SPECT (tracer injected immediately after the onset of the seizure and SPECT images showing perfusion at that point of time) shows an area of hyperperfusion in the epileptogenic region, surrounded by an area of hypoperfusion, which becomes more prominent at the end of the ictal phase. This surrounding area of hypoperfusion may be caused by steal syndrome (shift of blood flow to the seizure focus) or this area may function as an inhibitory zone trying to limit the seizure spread.12 Interictal SPECT (tracer is injected when patient is not having any clinical or subclinical seizure and SPECT images show the baseline perfusion pattern) shows hypoperfusion or normal perfusion in the epileptogenic region. Even when present, hypoperfusion may be very mild and sometimes difficult to distinguish from the surrounding normal brain on visual examination. The main role of interictal SPECT currently is to assist in the evaluation of ictal SPECT, visually or quantitatively, using statistical parametric mapping (SPM) or SISCOM (subtractionictal SPECT coregistered to MRI, i.e., the interictal SPECT images are subtracted from the ictal images and the results are displayed on coregistered MR images), by providing a baseline blood flow. Use of these registration techniques can increase the sensitivity and specificity of ictal SPECT. Studies have shown that SPM can increase the sensitivity of SPECT scan over visual analysis.13–15 However, lack of age-matched control subjects can make its use difficult, particularly in children younger than 6 years of age.16 SISCOM, conversely, appears to be very useful in children. The probability of localizing an ictal onset zone has been reported higher with SISCOM, compared with ictal EEG and MRI.17,18 Use of SISCOM can help in revisiting and detecting subtle changes in MRI, which were initially reported normal.19 Studies have shown that the area of the resected SISCOM abnormality is associated with the surgical outcome; the larger the area of resected SISCOM abnormality is, better the outcome will be.17,20
The major limitation of SPECT in the pediatric population is that it is difficult to acquire good interictal or ictal brain SPECT because children may have very frequent and short-lasting seizures (such as infantile spasms or myoclonic epilepsy). Another limitation is the poor spatial resolution (10–15 mm) of SPECT, compared with FDG PET (~5–6mm), which becomes even more crucial in small pediatric brains.
Role of PET and SPECT in Pediatric Epilepsy Surgery
The role of PET and SPECT can be summarized as follows:
Detection of epileptogenic cortex
Determination of dual pathology (i.e., medial temporal involvement)
Assessment for secondary epileptic focus
Evaluation of the functional status outside the epileptogenic zone
Evaluation of eloquent cortex
Postsurgical evaluation
Epileptogenic Region
Temporal Lobe Epilepsy
In temporal lobe epilepsy, interictal hypometabolic regions are not strictly confined to the presumed temporal epileptogenic zone or to the brain tissue showing pathological changes. They usually extend beyond temporal structures to ipsilateral parietal and frontal cortex as well as thalamus and also occasionally to the contralateral temporal lobe.21–24 Although this may represent the epileptic network involved in seizure propagation and may be related to behavioral and neuropsychological changes seen with chronic epilepsy, these extratemporal hypometabolic regions should be further investigated. FMZ PET is highly sensitive in temporal lobe epilepsy and shows decreased FMZ binding in the sclerotic hippocampus,22,25 and the reduction in FMZ binding is usually more than can be accounted for by the loss of hippocampal volume.26 Contrary to FDG PET, which usually shows extratemporal hypometabolism in parietal and frontal cortex in cases of temporal lobe epilepsy (probably associated with cognitive dysfunction or reflecting diaschisis), decreased FMZ binding usually represents neuronal loss or receptor changes related to epileptogenicity and, therefore, should be more closely scrutinized.22 The sensitivity of FMZ in detection of unilateral hippocampal sclerosis has been reported to be up to 100% with contralateral abnormalities in one third of patients.22,25 In MRI-negative patients, FMZ PET has been found to be abnormal in up to 85% patients with temporal lobe epilepsy.27–29 FMZ PET appears to be more sensitive than FDG PET in identifying an epileptogenic region and is associated with better surgical outcome, even when the MRI is normal. Use of SPM can further increase the accuracy of FMZ PET, with detection of subtle changes in FMZ binding, which is difficult to appreciate visually.25,30 These SPM studies sometimes found increased FMZ binding also,31–34 which, in some cases, indicated cortical developmental malformations.32 Use of SPM also revealed increased FMZ binding in the normal appearing temporal lobe white matter, which was found to be microdysgenesis on histopathological examination.35 This is an interesting finding, because these ectopic neuronal clusters may lead to epileptogenesis by providing an aberrant circuitry. Another PET tracer, AMT does not appear to be very useful in cases of medial temporal lobe epilepsy, particularly with hippocampal sclerosis.
In temporal lobe epilepsy, the pattern of perfusion on ictal SPECT depends on the origin of seizures. Although cases with medial temporal lesions usually show well-localized area of hyperperfusion involving ipsilateral medial and lateral temporal lobe, in cases of lateral temporal lesions, bilateral hyperperfusion is usually seen with higher increase in the ipsilateral side.36,37 This can be explained on the basis of different mechanisms of seizure propagation depending on the neuronal connectivity. The timing of tracer injection is also important in the case of temporal lobe epilepsy, because ictal, postictal, and peri-ictal SPECT scans have different perfusion patterns, which also depend on the area of temporal lobe involved. In the case of medial temporal lobe epilepsy, ictal scan (tracer injection within 20 seconds of the seizure onset) typically will show hyperperfusion of the entire medial temporal lobe along with surrounding hypoperfusion of orbital cortex or the entire frontal lobe. Peri-ictal scan (slightly delayed injection, 20–60 seconds after the seizure onset) will show hyperperfusion in lateral temporal cortex, orbital cortex, basal ganglia, or in contralateral temporal lobe, probably because of rapid seizure propagation. Postictal scan (injection within 4 minutes from the end of seizure) will show persistent hyperperfusion in medial temporal lobe with hypoperfusion in lateral temporal lobe gradually extending to surrounding hyperperfused areas. The medial temporal lobe remains isoperfused for another 10 to 15 minutes and then gradually becomes hypoperfused, resembling the interictal scan.
Although an extensive experience regarding SPECT is available for adults, not much data exist for the pediatric population. Overall, interictal SPECT has very low sensitivity (less than 50%) in the detection of epileptogenic regions in pediatric temporal lobe epilepsy, with false-positive or false-negative findings in 20 to 75% cases. However, ictal SPECT can correctly localize the epileptogenic foci in 70 to 90% of cases with unilateral temporal lobe epilepsy.38–41 As alluded to previously, various registration techniques, such as SPM or SISCOM, can further increase the sensitivity and specificity of ictal SPECT. In children, SISCOM was found to be helpful in identifying the epileptogenic region in up to 95% of cases.19 Compared with intracranial EEG findings, ictal SPECT was found to correctly localize the seizure onset zone in 80% of children with intractable epilepsy.42 False localization was caused by rapid seizure propagation or subclinical seizure onset. In addition, in the majority (70%) of children with favorable outcome of resective epilepsy surgery, the surgical margin coincided with the SPECT focus. Postictal SPECT is also more sensitive (70–90%) than interictal SPECT and can improve further with use of SISCOM.18,43 SISCOM can be particularly useful in cases of dysembryoplastic neuroepithelial tumor (DNET), which is more prevalent in the pediatric population. SISCOM can demonstrate some additional dysplastic areas around the DNET, and removal of these areas is essential for better surgical outcome. The use of SISCOM can increase the focus detection rate up to 93%, compared with 74% without it.1,19,44
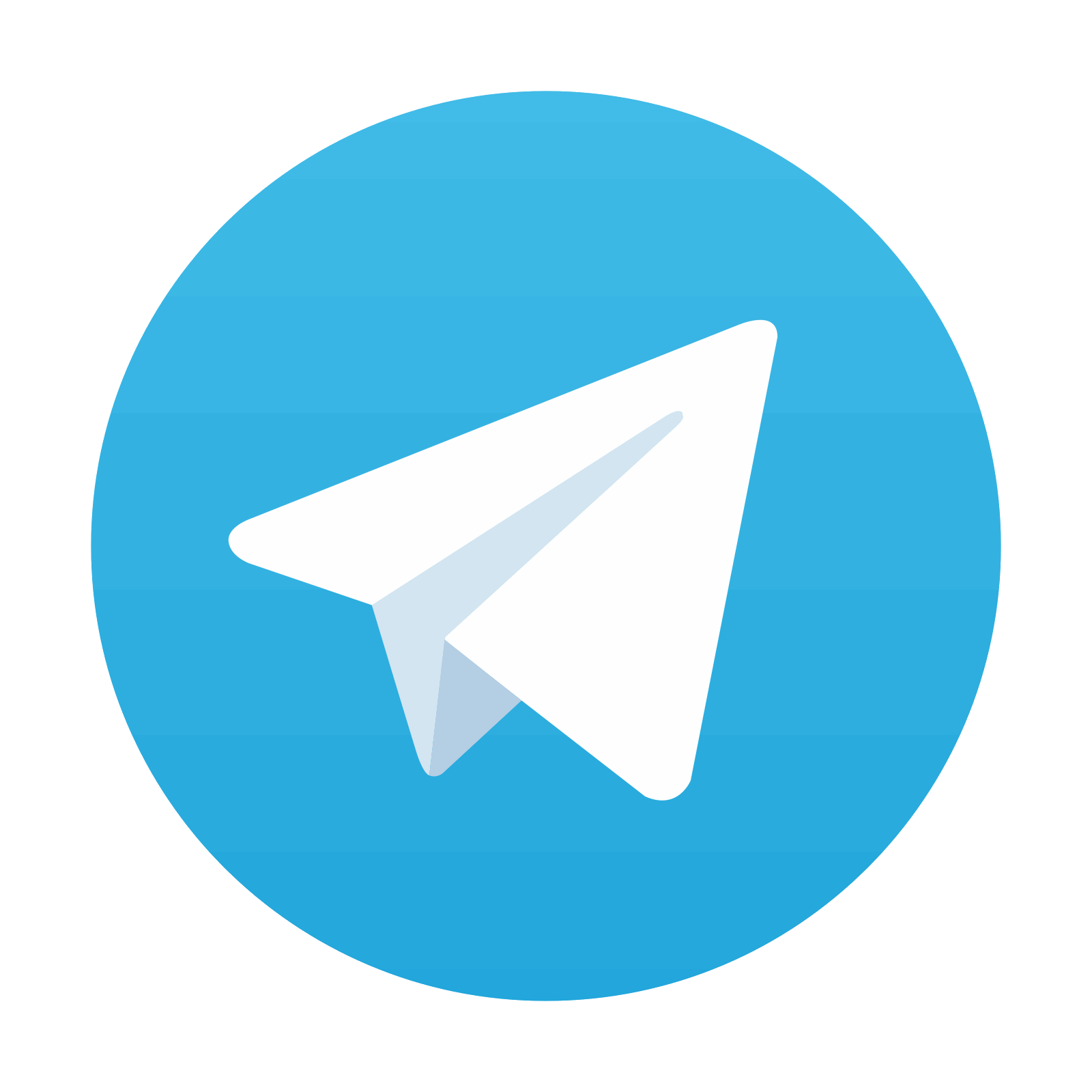
Stay updated, free articles. Join our Telegram channel

Full access? Get Clinical Tree
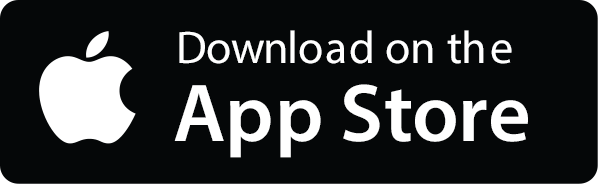
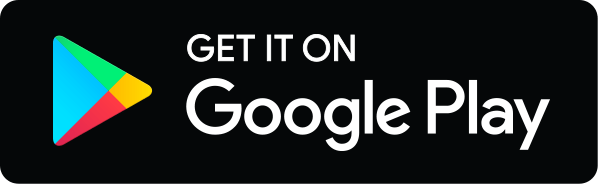
