Summary
The treatment of benign tumors of the skull base relies mainly on a surgical approach whose purpose is to obtain a maximal safe resection as well as, if possible, a complete resection. However, the proximity of functional neurovascular structures such as cranial nerves and the brainstem to skull-based tumors results in frequent inability to obtain a complete resection, leading to frequent local recurrences, significant neurologic disabilities, and decreased survival. Most benign skull-based tumors display a slow growth rate, which often results in intrinsic radioresistance and chemoresistance. Though biologically defined as “benign,” these tumors are ultimately complicated by clinical evolution that is far from “benign,” being mainly caused by the therapeutic limitations and high risk of recurrence already noted. Nevertheless, considerable progress has been achieved recently in understanding the underlying genetic, epigenetic, molecular, and cellular signaling mechanisms of these tumors, opening a pathway to potentially new therapeutic strategies. Nonetheless, the clinical development of targeted drugs for the different types of benign tumors of the skull base is at an early stage. Limitations such as the rarity of several of these tumors, the lack of preclinical animal model, and the lack of understanding of their clinical relevant biology remain to be addressed. The current review focuses on those benign skull-based tumors that exhibit the genetic and molecular understandings most promising for targeted therapies. The review provides an update on the current management, pathogenesis, and recent therapeutic advances of these tumors.
10 Management of Benign Skull Base Tumors in Neuro-oncology: Systemic Cytotoxic and Targeted Therapies
10.1 Introduction
Tumors of the skull base are categorized and defined as “benign” according to their pathologic and biologic behavior. Nevertheless, their anatomical location in proximity to critical neurovascular structures such as the cerebral vasculature, cranial nerves, and brainstem generally does not safely permit optimal surgical treatment. The absence of complete resection leads to high local recurrence rates, significant neurologic disabilities, compromised quality of life, and decreased overall survival. A comprehensive approach is thus necessary for management of benign skull base tumors, which often involves multiple therapeutic modalities. The slow growth rate that characterizes “benign tumors” is in large part responsible for their commonly seen intrinsic radioresistance and chemoresistance. This chapter provides an update on the pathogenesis and current neuro-oncology management of these tumors, including the related therapeutic advances from 2018 and earlier. Some benign tumors of the skull base, such as chondroma, paraganglioma, and pituitary adenoma, are not discussed, whether because they are discussed elsewhere or because there are insufficient data regarding active systemic cytotoxic or targeted treatment. The current review focuses on the benign skull-based tumors that are candidates for new systemic therapeutic approaches based on recently identified genetic and molecular targets. These tumors mainly comprise World Health Organization (WHO) grade I meningioma, chordoma, craniopharyngioma, and giant cell tumor of the bone.
10.2 WHO Grade I Meningioma
10.2.1 Introduction and Treatment
Benign or WHO grade I meningioma, when located in the skull base area, remains a challenge for complete and safe surgical resection; consequently, residual tumor remains postoperatively and there is often a significant risk of neurologic morbidity due to proximity to adjacent eloquent brain. The 2007 WHO classification, updated in 2016, did not change the grading of meningioma except to include existence of brain invasion with a mitotic count ≥ 4 that now categorizes WHO grade II or atypical meningioma.1 It was previously established that the prognosis and clinical evolution of grade I meningioma with brain invasion was like those characterized as grade II lesions.2
Skull base WHO grade I meningioma has a typically indolent onset, with minimal symptoms until tumors become large enough to compromise adjacent functional neurovascular structures. The slow onset results in a delay in diagnosis and presentation, often at an advanced stage.3 Depending on the location of the WHO grade I meningioma in the skull base, presenting deficits are usually referable to proximate cranial nerves.
Meningiomas of the skull base originate from the olfactory groove, sphenoid wing, suprasellar and parasellar regions, petroclival, cerebellopontine angle, and foramen magnum.4 Imaging is often characteristic, although radiographic findings may vary among patients. On CT, meningiomas are hyperdense and sometimes calcified. On MRI, meningiomas often are isointense with gray matter on all sequences, and contrast enhancement is intense and homogenous, although necrotic areas may be observed in large lesions.4
Meningiomas are highly vascularized tumors that have high relative cerebral blood volume (rCBV) values on MR perfusion. MR perfusion may assist in the differential diagnosis of skull-based lesions.5 , 6 , 7 Diffusion tensor imaging provides additional information about the tumor’s cellular content and may help differentiate among atypical, fibroblastic, and benign meningiomas.4 , 8 , 9 High alanine and low N-acetylaspartate peaks are observed on MR spectroscopy (MRS), but bone artifacts in the skull base often limit the use of MRS except for large lesions.10
The initial management of patients who have WHO grade I meningioma of the skull base usually consists of surgery, surgery plus radiation therapy (RT), RT alone, or observation.3 , 11 , 12 Observation is an option in asymptomatic and small lesions, those defined by National Comprehensive Cancer Network (NCCN) guidelines as having a diameter of less than 3 cm, but an observation-only approach is predicated in part on the tumor’s anatomical proximity to neurovascular structures. Surgery is recommended for symptomatic lesions whenever possible if it can be performed without sustaining functional morbidity. In instances of incomplete resection, postsurgical RT is often administered.3 , 11 , 12 Differing methods of RT may be used, including conformal external fractionated RT, stereotactic radiosurgery (SRS), stereotactic radiotherapy, intensity-modulated RT (IMRT), and volumetric-modulated arc RT.13 , 14 , 15 , 16 , 17 As of 2019, systemic treatment does not have an indication in the frontline treatment of meningioma. Particularly with skull-based WHO grade I meningioma, recurrence is frequent, and surgery or RT are most often deployed again as first salvage therapy. A variety of systemic therapies have been evaluated in surgical and RT refractory meningiomas, including chemotherapy, hormonal therapy, immunotherapy, and targeted therapies. These therapies are discussed hereafter. Regardless, most systemic treatments show modest activity. Recent identification of several molecular and druggable targets may allow development of more active systemic therapies in the future.
In all grades of meningioma, the prognosis is related to extent of resection (Simpson grade), patient characteristics such as gender (unfavorable for male), and tumor pathologic profile, such as WHO grade, Ki67/MIB-1 index, percentage of tumor cells in the S phase, p53 overexpression, telomerase activity, and telomerase reverse transcriptase (TERT) promoter mutation status (unfavorable when mutated).18 , 19 , 20
10.2.2 Pathogenesis, Genetics, Molecular, and Cellular Biology
Nearly 60% of WHO grade I meningiomas exhibit mutations of the NF2 gene on chromosome 22 (location: q12.2).21 , 22 , 23 , 24 The NF2 gene codes for the protein merlin, which belongs to the 4.1 family of structural proteins, and behaves as a tumor suppressor. Merlin is named for its similarity to moesin, ezrin and radixin-like protein. Variable levels of merlin loss are observed in different subtypes of grade I meningiomas (lower loss in the meningothelial type than in fibrous and other).25 Merlin localizes to the cell membrane and is involved in controlling cell–cell contact and cell motility.
The following proteins show some degree of interaction with merlin: CD44 and β1-integrin, βII-spectrin, paxillin, actin, and syntenin. These proteins are involved in cytoskeleton dynamics, sodium–hydrogen exchange regulatory factor, hepatocyte growth factor–receptor regulation, and endocytosis.26 Merlin downregulates the Yes-associated protein (YAP), a protein that controls cellular proliferation. NF2 mutations result in increased proliferation of arachnoid cap cells and meningioma development.27
Besides merlin loss, other protein 4.1 family members are also downregulated in grade I meningiomas. Up to 50% of WHO grade I meningiomas display a dual loss of protein 4.1B (DAL-1) and merlin expression.28 In up to 85% of sporadic meningioma, loss of TSLC-1 gene (tumor suppressor gene for lung cancer-1) is observed, triggering dysfunctional interactions between its protein and DAL-1.29 In normal conditions, the protein of the TSLC-1 gene (tumor suppressor gene for lung cancer-1) interacts with DAL-1, which is present in the plasma membrane, close to cell–cell contact points. Both proteins are spectrin–actin-binding proteins, and when they interact by direct binding, they influence cell motility through actin reorganization.30
The therapeutic targeting of TSLC-1 and DAL-1 might be part of a future strategy of anti-invasion and antimigration in meningiomas that exhibit intact expression of these two proteins.26 , 31 AKT1 mutation is found almost exclusively in skull-based grade I meningiomas and has become another potential therapeutic target since the development of AKT inhibitors.32 , 33
Multiple growth factors and their cognate receptors are observed in meningiomas and likely determine, at least in part, progression through overexpression and dysregulation. These include platelet-derived growth factor BB (PDGF-BB), epidermal growth factor receptor (EGFR), vascular endothelial growth factor (VEGF), transforming growth factor alpha and beta (TGF-alpha and -beta), SDF1 and receptor CXCR, bone morphogenic protein, insulin growth factor I and II (IGF), HER2, somatostatin, fibroblast growth factor, placental growth factor, and cyclo-oxygenase-2 Cox-2 (34–36). EGFR and platelet-derived growth factor receptor beta are both overexpressed in WHO grade I meningiomas.34
Meningiomas show alterations of chromosomes 1, 9, 10, 14, and 22, and their proliferation has been associated with mutations of genes in chromosomes 2, 4, 7, 8, 11, 12, and 16, leading to the activation of multiple signaling pathways. The most prominent ones are summarized hereafter.26 , 35 The Wnt/β catenin pathway is involved in grade I meningiomas, in which deletions of the tumor suppressor APC gene are observed.36 , 37 A third meningiomas of all grades display losses of E-cadherin function, leading to increased invasiveness and higher recurrence rates.37 The Notch pathway is an intracellular signaling pathway mediated by the transmembrane proteins Notch 1–4.38 , 39 Hes-1 is a protein that is a target of the Notch signaling pathway, and its expression is found in all grades of meningioma and related to an overexpression of the Jagged ligand, Notch 1, and Notch 2. TLE2/TLE3 are enhancers that increase the activity of the Split family (co-repressors) and that modulate Hes-1, a NOTCH signaling protein but appear to be upregulated in high-grade meningiomas only.39
The hedgehog (Hh)/patch (PTCH) pathway is involved in cell growth and is regulated by the (Smoothened) SMO gene. When Hh binds to PTCH, it activates SMO, leading to the activation of GLI transcription factors such as GLI1 (growth activator), GLI2 (growth activator), and GLI3 (growth repressor). The Hh pathway is also involved in angiogenesis, matrix remodeling, and stem cell homeostasis.26 A recent large series demonstrated that 28% of SMO mutations (L412F or W535L) and 15% of AKT1E17K mutations are seen in meningiomas of the olfactory groove.40 Among WHO grade I meningiomas, the group having SMO mutations had a significantly poorer prognosis. Meningiomas in the SMO-mutant group had an overall 36% recurrence rate, significantly higher than in the AKT1-mutant group (16%) and the “SMO and AKT1 wildtype” group (11%). All late recurrences, defined as 5 years after diagnosis, occurred in the SMO-mutant group. The authors suggest that the high frequency of SMO mutations in meningiomas arising from the anterior and medial skull base might be explained by the central role of the sonic hedgehog pathway in craniofacial development during embryogenesis. These results support the systematic determination of SMO mutations, both for prognosis and for potential inclusion of patients in future trials targeting this pathway using Hh inhibitors. The p53/pRB pathway controls the G1 to S phase cell cycle transition through the tumor suppressor gene pRB and is involved in meningioma progression and development of the anaplastic grade.26
The PI3K/AKT and mitogen-activated protein kinase (MAPK) are two pathways involved in grade II/III meningiomas only, in which high levels of phosphorylated Akt (PI3K/Akt) are found.21 , 41 , 42 , 43 , 44 Decreased levels of MAPK lead to higher recurrence rate. Other pathways, such as the phospholipase A2-arachadonic acid cyclo-oxygenase pathway, the PLC-gamma1-PKC pathway, and the transforming growth factor-beta (TGF-b)-SMAD signaling pathway, have all been related to meningioma pathogenesis.45 , 46 , 47 The levels of expression of cox-2 and of tumor necrosis factor–related apoptosis-inducing ligand receptor 4 (TRAIL-R4) seem correlated to the grade of meningioma.48 KIT expression is upregulated in 20.6% of meningiomas and is another potential druggable target.18 TERT promoter mutations, which are correlated to a decreased time to progression and a higher risk of recurrence, are present in only 1.7% of WHO grade I meningiomas.47
10.2.3 Systemic Therapies
An accurate assessment of the therapeutic efficacy in meningiomas has not been possible until recently, mainly because of the heterogeneity of response criteria used in different series. The Response Assessment in Neuro-Oncology (RANO) working group recently proposed use of the 6-month progression-free survival (PFS-6) as the standard in clinical trials in recurrent meningiomas.49 The weighted PFS-6 of WHO grade I meningioma determined by the RANO authors was 29% (95% confidence interval: 20.3–37.7%). The authors concluded that a new therapeutic compound should demonstrate a PFS-6 > 50% in WHO grade I meningioma if it is to be considered a therapy of interest.49 Nevertheless, there is still a lack of standardization regarding the optimal response criteria. Due to the complex shape of meningioma, the potential superiority of volumetric measures as compared with 2D McDonald criteria has been highlighted.50
Chemotherapy
Hydroxyurea (HU), temozolomide, and irinotecan have been evaluated in phase II trials, retrospective cohorts, and case studies in patients having recurrent WHO grade I meningiomas.20 , 51 , 52 , 53 , 54 , 55 , 56 , 57 , 58 , 59 , 60 , 61 , 62 The reported median PFS ranged from 4 to 90 months.54 , 55 , 58 , 59 PFS-6 was retrospectively assessed in one series of 60 patients and was only 10%.59 Using a combination of HU and fractionated conformal RT in 13 patients who had recurrent or progressive lesions, median PFS-12 was 84%, but the specific contribution of HU cannot be disentangled from the effects of radiotherapy.56 A phase II trial did not show any efficacy of temozolomide in 16 patients with a PFS-6 of 0%.60 Similarly, irinotecan did not display activity in a series of 16 patients with a reported PFS-6 of 6%.61 Trabectidin, a novel marine-derived antineoplastic agent, has shown a significant cytotoxic activity in grade II/III meningioma in preclinical studies.63 Synergy with HU, cisplatin, and doxorubicin was observed as well. These results led to the development of a phase II clinical trial in recurrent high-grade meningioma, but WHO grade I meningioma are currently excluded.
Hormonal Therapy
Thirty percent and 70% of meningiomas express receptors to estrogen and progesterone, respectively.45 , 64 , 65 , 66 , 67 Although PFS-6 is lacking in most published studies, the reported data fail to show any compelling efficacy of any antiestrogen or progesterone agents in recurrent and surgery-naive meningioma WHO grade I. Tamoxifen has been assessed in recurrent meningioma, including a single phase II trial, without significant effect.45 , 64 , 65 , 66 , 67 , 68 No radiographic responses were observed, although prolonged stabilization was seen in some studies.69 , 70 The progesterone-targeting agents megestrol acetate, medroxy-progeterone acetate, and mifepristone (RU-486) have been evaluated in grade I meningioma at recurrence or in meningiomas for which there was no prior surgery.71 , 72 , 73 , 74 , 75 Like antiestrogen-targeting agents, these agents achieved, at best, long-lasting disease stabilizations.71 , 74 Mifepristone was reported to have resulted in limited prolonged volumetric reductions in a few cases but had no significant impact when assessed in a randomized double-blind phase III study in grade I nonsurgical meningioma.75
Somatostatin Analogs
Ninety percent of meningiomas express somatostatin receptors, mostly of the sst2A subtype76 Several retrospective cohorts and phase II studies have assessed the effect of somatostatin analogs such as somatostatin, pasireotide, octreotide (Novartis), Sandostatin LAR (Novartis), pasireotide (Novartis), 90Y-DOTATOC (Abbott), 177Lu-DOTATOC (Novartis) on progressive or recurrent meningiomas of all grades.50 , 76 , 77 , 78 , 79 , 80 Some studies report global PFS-6 rates for tumors of all grades between 32 and 44%.50 , 76 A PFS-6 of 50% was observed in recurrent or progressive grade I meningiomas with pasireotide LAR, albeit without any objective radiographic response.50 The activity of these agents seems limited, notwithstanding a subpopulation of responders’ appearing to benefit from somatostatin-based therapy. Factors such as differing expression of somatostatin receptors (sst2 and 3) and level of octreotide uptake might be predictors of response and longer PFS and survival.50 , 76 , 77 , 78 , 80
Interferon Alpha
An encouraging PFS-6 rate of 54% was obtained in a phase II trial of patients who had refractory WHO grade I meningioma treated using interferon-alpha, an active agent in vitro.45 , 67 , 81 , 82 , 83 Although no objective radiological response was reported, 74% of patients were stabilized with a median PFS of 7 months and a median overall survival of 8 months (range 3–28 mo).81
Molecular Targeted Therapies
The lack of current effective treatment in recurrent or progressive WHO grade I meningioma is related in part to an insufficient understanding of the molecular pathogenesis of these tumors.26 Despite the identified overexpression of multiple growth factors, including platelet-derived growth factor (PDGF), Epidermal growth factor (EGF), vascular endothelial growth factor (VEGF), insulin-like growth factor (IGF), transforming growth factor-beta (TGF-β), and their receptor tyrosine kinases, and the dysregulation of their downstream signaling pathways, including the RAS/MAPK, PI3K/Akt, PLC-γ1-PKC, and TGF-β-SMAD pathways, their respective importance is poorly understood, and druggable targets need to be identified.26 Moreover, other approaches involving insulin-like growth factor receptor 2 (IGFR-2), histone deacetylase, NFκB, HSP90, JAK/STAT, immune checkpoint inhibitor, telomerase inhibitors, BRAF inhibitors, Src kinase, focal adhesion kinase (FAK), and hypoxia-inducible factor 1α might lead to potential new treatments.26 , 84 , 85
Sixty percent of meningiomas express EGFR.86 , 87 A phase II trial evaluating either gefitinib or erlotinib showed poor efficacy in recurrent meningioma WHO grade I patients who had a PFS-6 rate of 25%, 13 months of median overall survival, and 9 weeks median PFS.88 Most meningiomas of all grades express platelet-derived growth factor (PDGF)-b receptor and manifest an increased level of PDGF.89 , 90 In vitro, meningioma cell proliferation is inhibited by anti-PDGF antibodies.91 , 92 Imatinib, an inhibitor of PDGF receptor (PDGFR), was given as a single agent in a phase II trial.93 It showed limited efficacy, with a PFS-6 of 45% in recurrent and progressive WHO grade I meningioma. When combined with HU in the same setting, PFS-6 reached 87.5%.94 In a small randomized study of recurrent meningioma (all grades) that compared HU with HU + imatinib, PFS-9 (9-month PFS) was 0% and 75%, respectively.95
Meningiomas of all grades are highly vascularized and express VEGF and VEGF-R at variable levels related to grade (increased levels with higher grade).46 , 96 , 97 , 98 , 99 , 100 This pathway is involved in tumor growth vis-à-vis angiogenesis as well as in induction of peritumoral edema.45 , 66 , 83 , 101 Sunitinib (SU011248), a small molecule inhibitor that targets VEGF, PDGF, c-KIT, FLT, RET, macrophage colony–stimulating factor (CSF-1R), and valatinib (PTK787/ZK22584), a VEGFR 1–3 inhibitor, was ineffective in recurrent grade I meningiomas.102 Bevacizumab, a monoclonal antibody targeting VEGF, has been evaluated mostly in the retrospective setting and is often coadministered with either temozolomide or etoposide.99 , 100 , 103 , 104 In a phase II trial, bevacizumab was given to patients who had recurrent meningioma who were not otherwise considered candidates for local treatment. Results were encouraging in meningioma WHO grade I: PFS-6 of 87%, median PFS of 22.5 months, median overall survival of 35.6 months, and 100% tumor stabilization.105 Additionally, bevacizumab appears to have a mitigating effect on peritumoral edema and meningioma growth rate.106
Preclinical data show inhibiting activity of temsirolimus, an mTOR inhibitor, in meningioma in which the pathway is activated. Furthermore, a synergistic effect was seen when octreotide was added to everolimus.107 , 108 Consequently, this combination is being pursued in all grades of recurrent meningioma patients in a clinical trial with PFS-6 as the primary objective (CEVOREM/NCT02333565).
The therapeutic potential of TRAIL agonists has been evaluated in meningioma cell lines.108 TRAIL-induced apoptosis was seen in 29.7% of cell lines, but native TRAIL receptor expression was not predictive of TRAIL agonist sensitivity. The coadministration of bortezomib, which induces NOXA expression and downregulation of c-FLIP, may increase sensitivity to TRAIL-induced apoptosis.109 FAK inhibitors that target NF2 and merlin loss seen in up to 60% of sporadic meningiomas represent another trial in progress in patients who have recurrent meningiomas.110 , 111
The FAK inhibitor GSK2256098 and vismodegib, a hedgehog pathway inhibitor, are being studied in progressive meningiomas with SMO/AKT/NF2 mutations. A histone deacetylase inhibitor and its impact on suppression of p-AKT are also being explored.18 , 112
Some of the numerous potential targets may preferentially be expressed in high-grade rather than low-grade meningiomas. For example, programmed death-1 ligand (PD-L1) is frequently expressed in higher-grade tumors, indicating that it may play a biological role in the aggressive phenotype of meningiomas and may be targetable by PD-1 and PDL-1.113
Other approaches, such as gene silencing, supplementation of lost genetic factors, regulation of YAP levels in NF2 mutated tumors, and addressing of deficient intrinsic and extrinsic pathways, may improve management of recurrent and progressive meningiomas.26
Insufficient understandings of the evolution of grade I meningiomas and the lack of a preclinical reliable animal model are significant barriers to the development of novel active therapies. The RANO criteria will help standardize results of future trials in recurrent meningioma.
10.3 Chordomas
10.3.1 Introduction to Chordomas and Treatment Overview
Chordomas of the skull base are rare, slow-growing, locally aggressive, and destructive bony tumors derived from embryonic remnants of the notochord. Chordomas located in the skull base represent a third of all chordomas and are most frequently located in the spheno-occipital region.114 Headaches and intermittent diplopia are the predominant clinical features at onset, followed by additional cranial nerves palsies that differ according to the topography of the chordoma (e.g., supra- or infraclival). Delays in diagnosis are frequently caused by nonspecific symptoms such as nasal congestion or dysphagia that are related to cranial nerve involvement but not always initially identified as being so.
CT imaging is the best technique for assessing bone destruction; it may show characteristic ring-forming calcifications.115 MRI better shows the extent of tumor, including soft tissue components and dural extension.116 Chordomas on MRI are hypo- or isointense on T1-weighted and hyperintense on T2-weighted MRI sequences. Contrast enhancement is heterogeneous, with a lobulated “honeycomb” appearance, reflecting the liquid and gelatinous mucoid components and necrotic-hemorrhagic areas.117 A fusion of CT and MRI ideally provides the most accurate assessment of the anatomical relation between soft tissue tumor components surrounding normal soft tissue and bone.
Though chordomas are classified as benign tumors, their prognosis is more akin to that of a malignant lesion, with high local recurrence rates, including systemic metastases (40% incidence overall, 12.5% from skull base chordoma), largely responsible for tumor-related lethality.118 , 119 , 120 , 121 , 122 The median survival rate is approximately 6.29 years with 5-year OS and PFS rates of 78.4 and 50.8%, respectively.114 , 121 , 123
Histologically, chordomas, like the notochord, are composed of large vacuolated physaliphorous cells surrounded by an extracellular myxoid matrix.118 , 124 Chordomas comprise three subgroups: conventional chordomas, chondroid chordomas, and dedifferentiated chordomas (i.e., those with sarcomatous transformation). Conventional chordomas, which comprise chordomatous features only, are most frequent, followed by chondroid chordomas, which comprise additional chondromatous features. Both entities share the same prognosis, unlike dedifferentiated or sarcomatous chordomas, which behave in a more malignant manner.114 , 125 Most chordomas stain positive for cytokeratin, and 80% are epithelial membrane antigen–positive. A limited number in addition are S100 protein–positive.126 , 127 , 128 Routine immunohistochemical staining for brachyury, a key transcription factor in notochord development that is overexpressed by chordomas, is a sensitive and specific diagnostic tool that also rules out cartilaginous tumors.129 , 130 , 131 , 132 , 133
The treatment of chordoma is continually evolving owing to continued progress in understanding underlying biology. Chordomas are radioresistant to standard radiation protocols and chemoresistant to classic cytotoxic therapies, in part as a result of their slow-growing kinetics. Standard radiation to the skull base is also limited by the radiosensitivity of the brainstem and other proximate intracranial neurovascular structures that increase the risk of radiation-related injury.134 , 135
For localized disease, consensus guidelines published in 2015 recommend a complete surgical resection with tumor-free margins.136 Classic open skull base approaches and less invasive endoscopic approaches are used to maximize the resection.121 , 122 , 137 , 138 , 139 , 140 , 141 , 142 When residual disease is remaining, often having a relationship to adjacent neurovascular structures, radiotherapy is recommended.143 , 144 , 145 , 146 Definitive (proton beam) RT is the preferred alternative in instances in which resection is not feasible. Recent advances in heavy particle RT, such as proton and carbon ion beam, are promising in the adjuvant setting of skull base chordomas.147 , 148 , 149 Adjuvant proton therapy, when added to maximal safe resection in skull base chordomas, led to 5-year local control rates of 75.8% and improved survival.147 , 148 Adjuvant carbon ion radiation provided similar local 5-year control rates of 70.0%.149 Image-guided IMRT (IG-IMRT) is also under evaluation for skull base chordoma.150 Cytotoxic chemotherapy or other systemic therapies have no proven efficacy and are thus not recommended in any line of treatment according to current guidelines.136 , 151 Nevertheless, several molecular therapies targeting essential pathways in chordomas are currently under evaluation and are discussed hereafter.
10.3.2 Pathogenesis, Genetics, and Molecular and Cellular Pathways
Advances in the molecular understanding of chordomas have led to the identification of promising prognostic markers and targetable pathways. These include genetic and epigenetic alterations that involve brachyury, downstream pathways, and receptor tyrosine kinases (RTKs). The brachyury or T gene located on chromosome 6q27 encodes a transcription factor essential for the development of the notochord.152 Normally silenced, brachyury is reexpressed at high levels in chordoma cells. Several lines of evidence also suggest a causative role of brachyury overexpression in chordoma formation. Further brachyury might be involved in the metastatic process by facilitating the epithelial–mesenchymal transition.153 , 154 , 155 , 156 As already mentioned, the expression of brachyury is very helpful for pathologic diagnosis, but its level does not seem correlated with prognosis.157
Other genetic alterations in chordomas include loss of p16, PTEN, CDKN2a/CDKN2b, and PDCD4.158 , 159 , 160 , 161 , 162 , 163 , 164 , 165 , 166 , 167 , 168 Losses on chromosome 1p and the FHIT gene and gains on 1q and 2p have been detected in skull base chordomas.152 , 160 Loss of chromosome 1p36, 9p loss of heterozygosity, and an elevated Ki67 proliferative index are associated with shorter OS in skull base chordomas.152 , 161 Additionally, loss of chromosome 1q, gain of 2p, and aberrant brachyury copy number are associated with recurrence.
Many alterations in the signaling pathways and growth factors are involved in chordoma biology as well. Both the PI3K/AKT/TSC1/TSC2/mTOR and STAT3 pathways are activated.162 STAT3 level of expression has reported prognostic value. Changes in EGFR signaling, activation of IGF1R, and loss of MTAP have also been identified.118 , 163 , 164
Protein tyrosine kinases (PTKs) mediate phosphorylation of selected tyrosine residues, resulting in functional activation of many proteins and thus playing a crucial role in cancer development. RTKs are specialized transmembrane PTKs that mediate signaling via sampling of the external environment. RTKs are composed of extracellular domains that bind cognate environmental ligands and of an intracellular domain that mediates the signaling event via dimerization and binding to other signaling molecules. EGFR overexpression is observed in 69 to 79.6% of chordomas and is related to tumor aggressiveness.144 , 163 , 165 , 166 , 167 PDGFR expression is also observed in chordoma. Although PDGFR promotes chordoma cell proliferation through activation of the PI3K/AKT, RAS/ERK, and STAT pathways, PDGFRβ is observed in the stromal component of the tumor and is likely involved in microenvironmental regulation.144 , 165 , 168 , 169 , 170 , 171
Increased expression of angiogenesis-related factors such as VEGF, hypoxia-inducible factor-1α (HIF-1α) and matrix metalloproteinase (MMP)-2 and -9 has been identified in chordoma tissue and cell lines.172 , 173 MMP-9 expression is correlated with higher rates of local recurrence and poor prognosis.173
Epigenetic alterations, especially modifications of the expression of microRNA (miRNA) have been identified in chordomas, and may be therapeutically targetable as illustrated in vitro.174 , 175 , 176 , 177 , 178 , 179 For example, the administration of nonselective histone deacetylase inhibitors significantly triggers apoptosis in chordomas cells.174 Similarly, the blockade of the constitutive downregulation of microRNA-1 in chordomas inhibits cellular proliferation, migratory and invasive properties, and the expression of the oncoprotein SLUG as well.176 , 177 The restoration of two constitutively downregulated miRNAs targeting EGFR, MET, and Bcl-xL block cell proliferation.175
10.3.3 Systemic Therapies
As already mentioned, cytotoxic chemotherapy has not proven efficacious and thus is not recommended in any line of treatment of chordomas. Brachyury based on its determinant role in notochord development and selective reexpression in chordoma makes this an attractive and potential target.145 This is illustrated in vitro by the differentiation and senescence of chordoma cells, triggered by silencing the brachyury gene.180 Currently no treatment directly targets brachyury. An alternative approach would be instead to target downstream or interacting signaling pathways such as FGFR/MEK/ERK signal-transduction and EGFR signaling pathways.181 , 182 Immunotherapy may be promising, as illustrated by the partial responses observed in advanced chordoma patients treated with an immune-stimulating therapeutic cancer vaccine designed to elicit brachyury-specific T-cell responses.183
Multiple PTKs are posited to be involved in chordoma development and progression, some of which are functionally redundant. Therapeutic strategies comprise simultaneous inhibition of different PTKs or blocking of downstream signaling pathways. In chordomas, the PI3K/AKT/mTOR pathway is activated and PTEN is inhibited.162 , 168 , 169 , 184 It was hypothesized that therapeutic activity might be seen with inhibition of signaling mediated by EGFR and PDGFR, both of which converge on the PI3K/AKT/mTOR pathway. Some data suggest a potential therapeutic role for combined AKT and mTOR inhibitors, such as rapamycin.162
Preclinical use of the combination of a mTORC1 (rapamycin), mTOR (MLN0128), and PI3K/AKT/mTOR (PI-103) inhibitors presaged clinical activity seen with both mTOR and PDGFR as single agents in recurrent chordoma. The combined clinical efficacy of the combination of an mTOR inhibitor and imatinib in imatinib-resistant patients who had chordomas illustrates another possible strategy.184 , 185 , 186 , 187 Improved activity-based molecular profiling of a patient’s tumor and identification of specific aberrant signaling pathways is likely the most effective approach, as illustrated by the spectacular efficacy of rapamycin on tumors having aberrant mTOR-pathway signaling.188 , 189
PDGFR inhibition with imatinib mesylate (alone or combined with sirolimus) has shown some antitumoral activity in case series and one phase II trial in chordoma patients, including several who had skull base chordomas.170 , 185 , 190 , 191 An overall clinical benefit of 64% and stabilization rate of 70% were observed in advanced chordoma patients during the phase II trial.190 Sorafenib, a dual inhibitor targeting PDGF and VEGF pathways, has also been prospectively assessed in a phase II trial of patients who had advanced and metastatic chordomas.192 The response rate was low (3.7%), although the 9-month PFS was 73% and the 12-month OS 86.5%. The modest efficacy of PDGFR inhibition probably reflects the limitation of drug delivery into the chordoma and thus drug exposure.144 , 145 , 169 , 192 EGFR inhibition has also shown responses and clinical improvement in a few case series and in a single phase II trial in patients who had recurrent chordoma, including skull base tumors.145 , 193 , 194 , 195 , 196 , 197 Anti-EGFR monoclonal antibodies such as cetuximab, and EGFR tyrosine kinase inhibitors such as gefitinib, erlotinib, and lapatinib, have been studied.145 , 193 , 194 , 195 , 196 , 197 Median PFS was 8 months in a phase II trial of lapatinib, suggesting the need for a larger trial to establish the role of this strategy.193
Inhibition of proangiogenic growth factors such as VEGF is another option for recurrent chordomas. In a small clinical series including patients who had skull base tumors, the combined use of erlotinib and bevacizumab, a humanized anti-VEGF antibody, led to disease stabilization.198 Small numbers of patients have been treated with the VEGF inhibitors pazopanib or sunitinib.120 One partial response was achieved with sunitinib, and 50% of patients benefited clinically from pazopanib, exhibiting 14- to 15-month disease stabilization. These data need further confirmation to confirm the place of anti-VEGF therapy in chordomas. Other potential therapeutic targets in chordomas, such as the inhibition of STAT3 with SD-1029, or the selective de novo purine synthesis inhibitor in MTAP-deficient chordomas, have shown promise in preclinical studies.164 , 199 , 200
Although epidemiologic data show an improvement over time in the management of chordomas, with improved survival and local control, this effect is related mostly to earlier diagnosis made possible by improved imaging techniques, to improved surgical resection using endoscopic endonasal approaches, and to recent developments in radiation techniques.118 , 121 , 136 , 138 , 139 The place of systemic therapies remains suboptimal and is confined to the recurrent setting when no other options are available. Multiple targeted therapies have been tried with modest results. These approaches need further development based on an improved understanding of the underlying molecular pathways relevant to chordoma and on the increasing use of molecular characterization of chordomas that match the molecular target with a targeted therapy.
10.4 Craniopharyngioma
10.4.1 Introduction to Craniopharyngiomas and Treatment Overview
Craniopharyngiomas (CPs) are rare slow-growing solid or mixed solid–cystic tumors that arise from remnants of Rathke’s pouch found along the midline from the nasopharynx to the diencephalon. These epithelial tumors commonly occur close to the optic chiasm in the suprasellar area but may also be observed within the sella, the third ventricle, and the optic system.201 , 202 , 203 , 204 , 205 CP often decrease life span and can consequently be considered low-grade malignancies. These tumors show no gender predilection and have a bimodal occurrence between 5 and 14 years in children and 50 and 75 years in adults.206
Clinical presentation is variable, depending on the tumor location, and may consist of visual acuity and field disturbances, a wide range of endocrinopathies, headaches caused by compression or hydrocephalus or meningeal spread, and depression. Growth retardation in children and sexual dysfunction in adults are the most frequent endocrine manifestations. Diabetes insipidus is also frequent. Endocrine and visual assessments, including visual field testing, are part of the systematic presurgical and postsurgical usual and customary evaluations. Neuroimaging most often shows a calcified cystic mass in the parasellar region, but both characteristics may be lacking in up to 25% of cases. Based on histological patterns, adamantinomatous and papillary CP are separate entities in the WHO classification.207 Both subtypes seem to share the same evolution in terms of treatment efficacy and survival.208 , 209
Surgery is the primary and major component of initial treatment. Surgery provides a diagnosis as well as relief of symptoms. Maximal safe resection is advocated, but cyst aspiration followed by partial resection is an alternative in some cases considering that the expected benefits of surgery should be balanced with potential surgical morbidities.
RT is indicated after partial resections and at recurrence.210 Stereotactic RT and RSR, IMRT, and proton beam RT are most often employed.210 , 211 , 212 , 213 , 214 , 215 , 216
Compressive cysts need to be addressed when causing hydrocephalus or visual or hypothalamic disturbances. Percutaneous aspiration or aspiration via an Ommaya reservoir, intracavitary irradiation with stereotactic administered radioisotopes, and intracavitary chemotherapy may be used for this purpose.217 , 218 , 219 , 220 , 221 , 222 , 223 , 224 , 225 , 226 , 227 , 228 , 229 Surgery and RT may result in a wide range of endocrine, visual, neurological, and cerebrovascular side effects that may appear early or late, depending on clinical context. These treatment-related deficits include panhypopituitarism and related complications such as obesity, diabetes insipidus, sleep and temperature regulation disorders, visual field impairment, cognitive and behavioral disorders, arterial stenosis and ischemic strokes, cerebral cavernomas and aneurysm, moyamoya syndrome, meningioma, and high-grade glioma.230 , 231 , 232 , 233
10.4.2 Pathogenesis, Genetics, Molecular and Cellular Overview
The two histologic subtypes of CP show distinct molecular genetics. Activation of the Wnt signaling pathway via activation of mutations in the gene encoding β-catenin (CTNNB1) are reported in 96% of adamantinomatous CP (ACP), a subtype of CP seen mostly in the pediatric population.234 , 235 , 236 , 237 , 238 , 239 This driver mutation leads to accumulation of the protein β-catenin, which plays a role in cell signaling and cell adhesion. The Wnt signaling pathway promotes cell proliferation and differentiation. When the Wnt pathway is activated, β-catenin localizes to the cytoplasm and nucleus and can be detected through standard immunochemistry and used as a marker of CTNNB1 mutation status.235 , 236 , 239 , 240
In one study using whole-exome sequencing, mutations of BRAF V600E oncogene were reported in 95% of papillary CP (PCP), which are commonly observed in the adult population and are not found in ACP.239 In another study using targeted Sanger sequencing, the BRAF V600E mutation was shown in 81% of PCP and in 12.5% of ACP displaying the CTNNB1 mutation as well. These data suggest that these mutations are not mutually exclusive but rather tend to be predominant in one specific subtype of CP.240 Immunohistochemistry provides the same sensitivity for detection of the BRAF V600E mutation as sequencing and is much more widely available.235 , 236 , 239 , 240 Circulating BRAF V600E DNA has been detected in blood during treatment with a BRAF inhibitor, indicating that “liquid biopsy” may become a tool in the diagnosis and response to treatment of BRAF V600E mutated CP.235 The activating mutation BRAF may serve as a potential therapeutic target.
10.4.3 Systemic Therapies
Intracavitary Chemotherapy
An alternative approach to managing cystic CP is administration of intracystic bleomycin by way of an implanted catheter and reservoir such as an Ommaya device.228 Experience is more limited than with intracavitary irradiation, but in one series of 17 children, intracystic bleomycin was well tolerated, with five complete remissions and a median progression-free interval of 1.8 years.229 This approach may have a role in delaying RT or aggressive surgery.
Biological Therapy
The intracystic administration of IFN-α via an intracystic catheter connected to an Ommaya reservoir was assessed in more than 75 patients.241 , 242 , 243 Three million units of IFN-α were administered three times per week. One cycle was defined as 4 weeks of therapy and a total of 36 million units. Patients received from one to nine cycles. In all studies, tolerance to therapy was good, no drug interruption occurred, and significant clinical and radiologic responses were observed in the majority of patients.
Targeted Therapies
The use of BRAF inhibitors in BRAF-mutated PCP may become a new therapeutic option, as suggested by dramatic isolated responses.244 , 245 , 246 Nevertheless, when vemurafenib was used, regrowth was observed as soon as the targeted therapy was interrupted.244 Combination of a BRAF inhibitor (dabrafenib) and a MEK inhibitor (trametinib) is the new standard treatment in BRAF-mutated melanoma, providing optimal inhibition of the MAPK kinase (MEK) and RAS pathways with a significantly reduced risk of death compared with that seen for use of vemurafenib alone.247 This approach combining dabrafenib and trametinib has recently been tried successfully in patients who have BRAF-mutated CP and will be further studied in a National Cancer Institute–sponsored multicenter clinical trial.235 , 245 , 246 , 248
Future trials are needed to confirm the value of targeted therapies in CPs with respect both to time of implementation (neoadjuvant to facilitate resection, adjuvant after incomplete resection or at relapse) and to overall activity in the multidisciplinary approach of these tumors.249
10.5 Giant Cell Tumor of the Bone
10.5.1 Introduction to Giant Cell Tumor of the Bone and Treatment Overview
Giant cell tumor of the bone (GCTB) is a rare, benign, slow-growing but locally destructive intraosseous and osteolytic tumor of skeletally mature young adults in their second to fourth decade, predominant in females and Asians. There is an increased incidence of GCTB of the skull in Paget’s disease. Although GCTB is considered a benign lesion, its evolution is unpredictable. Neurologic deficits often result from local invasive and compressive effects of the tumor. Prognosis is impacted by recurrence, malignant transformation, and the rare (< 5%) occurrence of metastases, predominantly to lungs. The local recurrence rate is high in cases of incomplete surgery, mostly within 2 years of surgery. The recommended follow-up period is 5 years only, because recurrences after that period are quite rare. Clinically, patients present with multiple cranial nerves palsies and headaches. Minimal work-up should include serum calcium and phosphorous, serum parathyroid hormone, bone scan, chest X-ray, contrast brain CT, and MRI. Additionally, some investigators advise performance of a chest CT, at least at time of recurrence, because lung metastases are most common at recurrence but on occasion may be inaugural.
A lytic lesion resulting from an intratumoral hemorrhage is evocative but not pathognomonic of the diagnosis by either skull film or CT scan.250 , 251 CT imaging is more accurate for assessment of bone architecture and MRI for the surrounding neurovascular structures. Either may show a hypervascularized and cystic lesion.252 , 253 The Campanacci classification categorizes GCTB into three grades (I, II, III), based on clinical and radiological features, but does not correlate with pathology, nor is it prognostic.254
GCTB consist of three cell-types: neoplastic giant cell tumor stromal cells, which have high proliferative activity; recruited mononuclear histiocytic cells; and multinuclear giant cells. The histologic heterogeneity of GCTB increases the difficulty of diagnosis, which is compounded by the limited sampling associated with core or fine-needle biopsies. It can be challenging to identify the atypical neoplastic stromal cells among the reactive giant cells and thus to distinguish between benign and malignant GCTB.255 , 256
Until now, surgery has been the mainstay of local treatment, achieving generally good local control rates when resection is complete. Recent advances in the understanding of the molecular pathogenesis of GCTB, and improvements in RT, have led to new and better-tolerated therapeutic options. Nevertheless, to preserve the adjacent neurovascular structures and minimize surgery-related injury, resection is often incomplete and adjuvant therapy thus required. To decrease the risk of post-RT malignant transformation, the NCCN has recommended RT as the last adjuvant option notwithstanding the established radiosensitivity of GCTB and its excellent local control rate. Stereotactic radiosurgery and IMRT have been recently used as adjuvant therapies but require further validation.251 , 257 , 258 Denosumab, a monoclonal antibody targeting the receptor activator of nuclear factor-κB ligand (RANKL), was approved by the FDA in 2013 for the treatment of GCTB.255 , 256 Other systemic therapies are discussed hereafter.259 , 260
10.5.2 Pathogenesis, Genetics, and Molecular and Cellular Pathways
The proliferative fraction of GCTB is thought to be derived from the stromal compartment, but the absence of cytologic aspects of malignancy in stromal cells, combined with the lack of clonal cytogenetic structural aberrations in GCTB in most studies, suggests that these cells may be reactive and not neoplastic.261 , 262 , 263 , 264 However, neoplastic stromal cells and mesenchymal stem cells share similar differentiation characteristics. When present, the overexpression of p53 and centrosome amplification are correlated with a high risk of recurrence, and the overexpression of p53 is also associated with an increased risk of metastases. Centrosome amplification is more frequent in recurrent and malignant GCTB.265 , 266 Fifty-four percent of GCTBs have 20q11 amplifications.267 Genetic instability is also suggested by the presence of a driver mutation in H3F3A, exclusively in the stromal cells in > 90% of GCTB.268 Furthermore, telomere dysregulation is present in up to 70% of GCTB, with a telomere protective-capping mechanism permitting telomere length maintenance.269 , 270 , 271 Telomere maintenance markers such as human telomerase reverse transcriptase and promyelocytic leukemia body-related antigens are expressed by GCTB.271 , 272 , 273 A telomeric fusion in chromosomes 11p, 13p, 15p, 18p, 19p, and 21p is present in more than 80% of GCTB, predominantly in grade III tumors.274
The multinucleated giant cells in GCTB result from the recruitment of CD68-positive monocytes attracted by SDF-1 and MCP-1, which are produced by stromal cells, and by the VEGF present in the stromal environment, because these monocytes express VEGFR1 (Flt1) among other macrophage markers.250 At a molecular level, RANKL (receptor activator of nuclear factor kappa B [NF-kB] ligand) plays a major functional role in the molecular pathogenesis of GCTB. The osteoblast-like mononuclear stromal cells display a high rate of expression of RANKL.255 , 262 , 275 , 276 , 277 , 278
Receptor activator of nuclear factor-kappa beta (RANK) ligand (RANKL) interactions and macrophage colony–stimulating factor (M-CSF) stimulate recruitment of osteoclastic cells from normal mononuclear preosteoclast cells that become multinucleated osteoclast-like giant cells.279 , 280 , 281 , 282 , 283 Through a cathepsin K- and MMP-13–mediated process, these giant cells cause the characteristic bone destruction observed in these lesions.284 , 285 , 286 Runx2 may be a driver in cytokine-mediated MMP-13 expression in GCTB stromal cells.287 CCAAT/enhancer binding protein beta(C/EBPbeta), by activating RANKL promoter, seems to be a significant factor in GCTB pathophysiology.288 Given the predominant role of RANKL in the genesis of these multinucleated giant cells, the inhibition of RANKL signaling by the use of the targeted antibody denosumab has proven highly effective.
The CD33 + phenotype of the giant cells in GCTB may also in the future be targetable using an anti-CD33 antibody such as gemtuzumab.282 , 283 Some cells also are positive for PDGFA, C-kit, and EGFR, all of which can be therapeutically targeted using commercial drugs.289 , 290 The EGFR is expressed by neoplastic stromal cells and plays a role in their proliferation, in osteoclastogenesis, and likely in the progression of the disease.289
Less prominent RANKL-independent pathways are involved in GCTB osteoclastogenesis and may represent another druggable target.279 These RANKL-independent pathways include tumor necrosis factor-α, interleukin-6, tumor growth factor-β, a proliferation-inducing ligand, B-cell activating factor, nerve growth factor, insulin-like growth factor I (IGF-I), and IGF-II. Further understanding of GCTB molecular pathogenesis will further inform the development of novel molecular-based therapies.
10.5.3 Systemic Therapies
Chemotherapy
There is limited evidence regarding the benefit of chemotherapy in benign GCTB, and because more efficient and better-tolerated systemic alternatives exist, it is usually not prescribed in these tumors except in cases of malignant GCTB. Doxorubicin, cisplatin, methotrexate, ifosfamide, and cyclophosphamide have been used in advanced aggressive, unresectable tumors, although in nonrandomized studies only.261 , 291 , 292 , 293 , 294 , 295 , 296 , 297
Interferon Alpha
Limited retrospective data suggest activity of IFNα in the treatment of aggressive GCTB but at the cost of poor patient tolerance—a common side effect of systemic interferon-based therapy.296 , 297 , 298 , 299 Consequently, the benefits and risks of IFNα should be balanced in clinical situations in which there are no alternatives.
Biphosphonates
Biphosphonates, with their antiosteoclastic properties, have shown activity in vitro in GCTB.300 , 301 However, clinical data are scarce, with limited case reports, retrospective series, and one phase II trial having assessed zoledronic acid.302 , 303 , 304 , 305 Modest clinical benefit and long-term local disease control have been reported in the adjuvant setting but without any significant preventive effect on local recurrence.250 , 300 , 302 , 303 , 304 , 305 , 306
Little information regarding the utility of calcitonin and sunitinib has been reported, but they represent other potential treatment options for recurrent GCTB.250 , 307 , 308 Local control is occasionally seen with oral steroids in patients who have Paget’s disease and GCTB.309 , 310
Denosumab
Denosumab is a fully human monoclonal antibody against RANKL. Its clinical benefit has been shown in phase II trials involving recurrent or unresectable GCTB.260 , 311 , 312 Reported objective response rates of 86% are seen along with a reduced need for resective surgery. Long-lasting responses are seen in most patients (96% PFS at a median follow-up of 15 months), which combined with a favorable toxicity profile (1% incidence of osteonecrosis) make denosumab the therapy of first choice in patients who have GCTB (Fig. 10.1).251 , 312

Based on results in 305 patients from two phase II trials, denosumab has been approved for patients who have unresectable GCTB or in whom a surgery would result in significant functional morbidity. NCCN guidelines emphasize indication for use of densoumab in instances of unresectable tumor. However, there are no data regarding the optimal duration or dose schedule of denosumab. Additionally, the optimal timing for the use of denosumab is also not well defined. Denosumab use has been proposed in the neoadjuvant setting, when surgery is expected to be incomplete, and when disease is clinically aggressive or is associated with a low performance status (PS) and impaired quality of life.273 The goals are to improve the resectability of the tumor by reducing its size (i.e., downstage) and to further achieve improvement in performance status (PS) and quality of life (QoL). Potential benefits in the adjuvant setting after surgery, to reduce local recurrence, is also under evaluation.312
There are limited data on the long-term safety of denosumab, especially relating to the most serious treatment-related adverse event, mandibular osteonecrosis. New challenges come with new therapies, and response assessment in GCTB is no exception. Postdenosumab treatment pathology can be challenging to interpret in some cases.313 Most often seen is a significant reduction of giant cells and neoplastic stromal cells, with some degree of bone reformation. However, cellular atypia and some patterns of ossification may suggest osteosarcoma or an undifferentiated pleomorphic sarcoma.255 , 260 , 313 Correlation of posttreatment pathology with clinical and imaging data is often necessary to achieve a correct diagnosis.
Although the role of (18F-FDG) PET scanning is not clear at time of diagnosis in GCTB, FDG-PET does appear to be a sensitive marker of early response to denosumab, as changes in standardized uptake values reflect tumor metabolism and angiogenesis.314 , 315 , 316 , 317 Nevertheless, MRI should not be replaced by FDG-PET; rather, the latter provides correlative information that may augment MRI findings.
The introduction of denosumab has profoundly altered the management of GCTB of the skull base, providing a new treatment option that augments surgery. However, because many questions remain unanswered regarding the practical aspects and timing of denosumab treatment, further prospective randomized trials are needed.
10.6 Conclusion
“Benign” tumors of the skull base are characterized by a high local recurrence rate and attendant consequences, including diminished QOL and decreased survival. In some of these tumors, such as chordomas, improvements in global management have been observed over time with earlier diagnosis and better local control as a result of improved imaging and surgical techniques. Nevertheless, management of these tumors remains challenging, and despite the recent making of considerable progress in understandings of their genetics and molecular pathogenesis, many limitations remain with respect to current systemic therapies.
Because these tumors are rare, treatment of numbers of patients large enough to allow determination of the benefits of novel targeted therapies can be difficult. As a result, most studies of targeted agents have involved a single institution and comprised small numbers of patients. Furthermore, and as has become increasingly apparent in other tumor types, molecular characterization and matching of actionable targets with targeted therapies are essential to best determining the role of these new therapies. Studies such as these will require multi-institutional efforts and likely also industry sponsors to provide access to novel targeted therapies. The application of precision or personalized oncology based on the molecular profiling of aberrant pathways in individual patients holds great promise for advancing the treatment of patients who have skull-based benign tumors.
10.7 References
- 1 Louis DN, Perry A, Reifenberger G et al. The 2016 World Health Organization Classification of Tumors of the Central Nervous System: a summary.. Acta Neuropathol 2016; 131 (6) 803-820 PubMed 27157931
- 2 Perry A, Stafford SL, Scheithauer BW, Suman VJ, Lohse CM. Meningioma grading: an analysis of histologic parameters.. Am J Surg Pathol 1997; 21 (12) 1455-1465 PubMed 9414189
- 3 Black PM, Villavicencio AT, Rhouddou C, Loeffler JS. Aggressive surgery and focal radiation in the management of meningiomas of the skull base: preservation of function with maintenance of local control.. Acta Neurochir (Wien) 2001; 143 (6) 555-562 PubMed 11534672
- 4 Mathur A, Jain N, Kesavadas C, Thomas B, Kapilamoorthy TR. Imaging of skull base pathologies: role of advanced magnetic resonance imaging techniques.. Neuroradiol J 2015; 28 (4) 426-437 PubMed 26427895
- 5 Hakyemez B, Erdogan C, Bolca N, Yildirim N, Gokalp G, Parlak M. Evaluation of different cerebral mass lesions by perfusion-weighted MR imaging.. J Magn Reson Imaging 2006; 24 (4) 817-824 PubMed 16958061
- 6 Zimny A, Sasiadek M. Contribution of perfusion-weighted magnetic resonance imaging in the differentiation of meningiomas and other extra-axial tumors: case reports and literature review.. J Neurooncol 2011; 103 (3) 777-783 PubMed 21061142
- 7 Kremer S, Grand S, Rémy C et al. Contribution of dynamic contrast MR imaging to the differentiation between dural metastasis and meningioma.. Neuroradiology 2004; 46 (8) 642-648 PubMed 15232661
- 8 Zhang H, Rödiger LA, Shen T, Miao J, Oudkerk M. Preoperative subtyping of meningiomas by perfusion MR imaging.. Neuroradiology 2008; 50 (10) 835-840 PubMed 18542938
- 9 Jolapara M, Kesavadas C, Radhakrishnan VV et al. Role of diffusion tensor imaging in differentiating subtypes of meningiomas.. J Neuroradiol 2010; 37 (5) 277-283 PubMed 20381865
- 10 Cho YD, Choi GH, Lee SP, Kim JK. (1)H-MRS metabolic patterns for distinguishing between meningiomas and other brain tumors.. Magn Reson Imaging 2003; 21 (6) 663-672 PubMed 12915198
- 11 Mendenhall WM, Morris CG, Amdur RJ, Foote KD, Friedman WA. Radiotherapy alone or after subtotal resection for benign skull base meningiomas.. Cancer 2003; 98 (7) 1473-1482 PubMed 14508835
- 12 NCCN Clinical Practice Guidelines in Oncology [Internet]. Available from: http://www.nccn.org/professionals/physician_gls/f_guidelines.asp#cns
- 13 Milker-Zabel S, Zabel-du Bois A, Huber P, Schlegel W, Debus J. Intensity-modulated radiotherapy for complex-shaped meningioma of the skull base: long-term experience of a single institution.. Int J Radiat Oncol Biol Phys 2007; 68 (3) 858-863 PubMed 17379447
- 14 Minniti G, Amichetti M, Enrici RM. Radiotherapy and radiosurgery for benign skull base meningiomas.. Radiat Oncol 2009; 4: 42 PubMed 19828022
- 15 Lee JY, Niranjan A, McInerney J, Kondziolka D, Flickinger JC, Lunsford LD. Stereotactic radiosurgery providing long-term tumor control of cavernous sinus meningiomas.. J Neurosurg 2002; 97 (1) 65-72 PubMed 12134934
- 16 Spiegelmann R, Cohen ZR, Nissim O, Alezra D, Pfeffer R. Cavernous sinus meningiomas: a large LINAC radiosurgery series.. J Neurooncol 2010; 98 (2) 195-202 PubMed 20405308
- 17 Skeie BS, Enger PO, Skeie GO, Thorsen F, Pedersen PH. Gamma Knife surgery of meningiomas involving the cavernous sinus: long-term follow-up of 100 patients.. Neurosurgery 2010; 66 (4) 661-668, discussion 668–669 PubMed 20305491
- 18 Sahm F, Schrimpf D, Olar A et al. TERT promoter mutations and risk of recurrence in meningioma.. J Natl Cancer Inst 2015; 108 (5) djv377 PubMed 26668184
- 19 Yew A, Trang A, Nagasawa DT et al. Chromosomal alterations, prognostic factors, and targeted molecular therapies for malignant meningiomas.. J Clin Neurosci 2013; 20 (1) 17-22 PubMed 22743042
- 20 Le Rhun E, Taillibert S, Chamberlain MC. Systemic therapy for recurrent meningioma.. Expert Rev Neurother 2016; 16 (8) 889-901 PubMed 27123883
- 21 Newton HB. Molecular neuro-oncology and development of targeted therapeutic strategies for brain tumors. Part 2: PI3K/Akt/PTEN, mTOR, SHH/PTCH and angiogenesis.. Expert Rev Anticancer Ther 2004; 4 (1) 105-128 PubMed 14748662
- 22 Kim WY, Lee HY. Brain angiogenesis in developmental and pathological processes: mechanism and therapeutic intervention in brain tumors.. FEBS J 2009; 276 (17) 4653-4664 PubMed 19664069
- 23 Wellenreuther R, Kraus JA, Lenartz D et al. Analysis of the neurofibromatosis 2 gene reveals molecular variants of meningioma.. Am J Pathol 1995; 146 (4) 827-832 PubMed 7717450
- 24 Mawrin C, Perry A. Pathological classification and molecular genetics of meningiomas.. J Neurooncol 2010; 99 (3) 379-391 PubMed 20809251
- 25 Pavelin S, Bečić K, Forempoher G et al. The significance of immunohistochemical expression of merlin, Ki-67, and p53 in meningiomas.. Appl Immunohistochem Mol Morphol 2014; 22 (1) 46-49 PubMed 23455188
- 26 Miller Jr R, DeCandio ML, Dixon-Mah Y et al. Molecular targets and treatment of meningioma.. J Neurol Neurosurg 2014; 1 (1) 1000101 PubMed 25485306
- 27 Perry A, Gutmann DH, Reifenberger G. Molecular pathogenesis of meningiomas.. J Neurooncol 2004; 70 (2) 183-202 PubMed 15674477
- 28 Saraf S, McCarthy BJ, Villano JL. Update on meningiomas.. Oncologist 2011; 16 (11) 1604-1613 PubMed 22028341
- 29 Striedinger K, VandenBerg SR, Baia GS, McDermott MW, Gutmann DH, Lal A. The neurofibromatosis 2 tumor suppressor gene product, merlin, regulates human meningioma cell growth by signaling through YAP.. Neoplasia 2008; 10 (11) 1204-1212 PubMed 18953429
- 30 Wang H, Xu M, Cui X et al. Aberrant expression of the candidate tumor suppressor gene DAL-1 due to hypermethylation in gastric cancer.. Sci Rep 2016; 6: 21755 PubMed 26923709
- 31 Pervaiz S. Anti-cancer drugs of today and tomorrow: are we close to making the turn from treating to curing cancer?. Curr Pharm Des 2002; 8 (19) 1723-1734 PubMed 12171544
- 32 Clark VE, Erson-Omay EZ, Serin A et al. Genomic analysis of non-NF2 meningiomas reveals mutations in TRAF7, KLF4, AKT1, and SMO.. Science 2013; 339 (6123) 1077-1080 PubMed 23348505
- 33 Sahm F, Bissel J, Koelsche C et al. AKT1E17K mutations cluster with meningothelial and transitional meningiomas and can be detected by SFRP1 immunohistochemistry.. Acta Neuropathol 2013; 126 (5) 757-762 PubMed 24096618
- 34 Dickinson PJ, Surace EI, Cambell M et al. Expression of the tumor suppressor genes NF2, 4.1B, and TSLC1 in canine meningiomas.. Vet Pathol 2009; 46 (5) 884-892 PubMed 19429976
- 35 Ragel BT, Jensen RL. Aberrant signaling pathways in meningiomas.. J Neurooncol 2010; 99 (3) 315-324 PubMed 20838852
- 36 Saydam O, Shen Y, Würdinger T et al. Downregulated microRNA-200a in meningiomas promotes tumor growth by reducing E-cadherin and activating the Wnt/beta-catenin signaling pathway.. Mol Cell Biol 2009; 29 (21) 5923-5940 PubMed 19703993
- 37 Zhou L, Ercolano E, Ammoun S, Schmid MC, Barczyk MA, Hanemann CO. Merlin-deficient human tumors show loss of contact inhibition and activation of Wnt/β-catenin signaling linked to the PDGFR/Src and Rac/PAK pathways.. Neoplasia 2011; 13 (12) 1101-1112 PubMed 22247700
- 38 Fernandez-Valle C, Tang Y, Ricard J et al. Paxillin binds schwannomin and regulates its density-dependent localization and effect on cell morphology.. Nat Genet 2002; 31 (4) 354-362 PubMed 12118253
- 39 Cuevas IC, Slocum AL, Jun P et al. Meningioma transcript profiles reveal deregulated Notch signaling pathway.. Cancer Res 2005; 65 (12) 5070-5075 PubMed 15958550
- 40 Boetto J, Bielle F, Sanson M, Peyre M, Kalamarides M. SMO mutation status defines a distinct and frequent molecular subgroup in olfactory groove meningiomas.. Neuro-oncol 2017; 19 (3) 345-351 PubMed 28082415
- 41 Santarius T, Kirsch M, Nikas DC, Imitola J, Black PM. Molecular analysis of alterations of the p18INK4c gene in human meningiomas.. Neuropathol Appl Neurobiol 2000; 26 (1) 67-75 PubMed 10736068
- 42 Cai SL, Tee AR, Short JD et al. Activity of TSC2 is inhibited by AKT-mediated phosphorylation and membrane partitioning.. J Cell Biol 2006; 173 (2) 279-289 PubMed 16636147
- 43 Vander Haar E, Lee SI, Bandhakavi S, Griffin TJ, Kim DH. Insulin signalling to mTOR mediated by the Akt/PKB substrate PRAS40.. Nat Cell Biol 2007; 9 (3) 316-323 PubMed 17277771
- 44 Xie J, Johnson RL, Zhang X et al. Mutations of the PATCHED gene in several types of sporadic extracutaneous tumors.. Cancer Res 1997; 57 (12) 2369-2372 PubMed 9192811
- 45 Chamberlain MC. The role of chemotherapy and targeted therapy in the treatment of intracranial meningioma.. Curr Opin Oncol 2012; 24 (6) 666-671 PubMed 22759739
- 46 Mawrin C, Chung C, Preusser M. Biology and clinical management challenges in meningioma. Am Soc Clin Oncol Educ Book ASCO Am Soc Clin Oncol Meet. 2015; 35:e106–e115
- 47 Preusser M, Berghoff AS, Hottinger AF. High-grade meningiomas: new avenues for drug treatment?. Curr Opin Neurol 2013; 26 (6) 708-715 PubMed 24184974
- 48 Kato Y, Nishihara H, Mohri H et al. Clinicopathological evaluation of cyclooxygenase-2 expression in meningioma: immunohistochemical analysis of 76 cases of low and high-grade meningioma.. Brain Tumor Pathol 2014; 31 (1) 23-30 PubMed 23250387
- 49 Kaley T, Barani I, Chamberlain M et al. Historical benchmarks for medical therapy trials in surgery- and radiation-refractory meningioma: a RANO review.. Neuro-oncol 2014; 16 (6) 829-840 PubMed 24500419
- 50 Norden AD, Ligon KL, Hammond SN et al. Phase II study of monthly pasireotide LAR (SOM230C) for recurrent or progressive meningioma.. Neurology 2015; 84 (3) 280-286 PubMed 25527270
- 51 Schrell UM, Rittig MG, Anders M et al. Hydroxyurea for treatment of unresectable and recurrent meningiomas. II. Decrease in the size of meningiomas in patients treated with hydroxyurea.. J Neurosurg 1997; 86 (5) 840-844 PubMed 9126900
- 52 Mason WP, Gentili F, Macdonald DR, Hariharan S, Cruz CR, Abrey LE. Stabilization of disease progression by hydroxyurea in patients with recurrent or unresectable meningioma.. J Neurosurg 2002; 97 (2) 341-346 PubMed 12186462
- 53 Rosenthal MA, Ashley DL, Cher L. Treatment of high risk or recurrent meningiomas with hydroxyurea.. J Clin Neurosci 2002; 9 (2) 156-158 PubMed 11922703
- 54 Newton HB, Scott SR, Volpi C. Hydroxyurea chemotherapy for meningiomas: enlarged cohort with extended follow-up.. Br J Neurosurg 2004; 18 (5) 495-499 PubMed 15799152
- 55 Loven D, Hardoff R, Sever ZB et al. Non-resectable slow-growing meningiomas treated by hydroxyurea.. J Neurooncol 2004; 67 (1–2) 221-226 PubMed 15072471
- 56 Hahn BM, Schrell UMH, Sauer R, Fahlbusch R, Ganslandt O, Grabenbauer GG. Prolonged oral hydroxyurea and concurrent 3D-conformal radiation in patients with progressive or recurrent meningioma: results of a pilot study.. J Neurooncol 2005; 74 (2) 157-165 PubMed 16193387
- 57 Weston GJ, Martin AJ, Mufti GJ, Strong AJ, Gleeson MJ. Hydroxyurea treatment of meningiomas: a pilot study.. Skull Base 2006; 16 (3) 157-160. 820 PubMed 17268588
- 58 Kim M-S, Yu D-W, Jung Y-J, Kim SW, Chang CH, Kim OL. Long-term follow-up result of hydroxyurea chemotherapy for recurrent meningiomas.. J Korean Neurosurg Soc 2012; 52 (6) 517-522 PubMed 23346322
- 59 Chamberlain MC, Johnston SK. Hydroxyurea for recurrent surgery and radiation refractory meningioma: a retrospective case series.. J Neurooncol 2011; 104 (3) 765-771 PubMed 21318318
- 60 Chamberlain MC, Tsao-Wei DD, Groshen S. Temozolomide for treatment-resistant recurrent meningioma.. Neurology 2004; 62 (7) 1210-1212. 835 PubMed 15079029
- 61 Chamberlain MC, Tsao-Wei DD, Groshen S. Salvage chemotherapy with CPT-11 for recurrent meningioma.. J Neurooncol 2006; 78 (3) 271-276 PubMed 16628476
- 62 Swinnen LJ, Renkin C, Rushing EJ et al. Proceedings SNO, annual meeting of the Society of Neuro-oncology, 18–21 Nov 2010, 830 Montreal, QC. Ongoing clinical trials. Abstract OT-08: iv70.. Neuro-oncol 2010; 12 (Suppl. suppl4) iv69-iv78 NOT_FOUND
- 63 Preusser M, Spiegl-Kreinecker S, Lötsch D et al. Trabectedin has promising antineoplastic activity in high-grade meningioma.. Cancer 2012; 118 (20) 5038-5049 PubMed 22392434
- 64 Chamberlain M. What constitutes activity of systemic therapy in recurrent meningioma?. Neurology 2015; 85: 1090 PubMed 26391415
- 65 Rockhill J, Mrugala M, Chamberlain MC. Intracranial meningiomas: an overview of diagnosis and treatment.. Neurosurg Focus 2007; 23 (4) E1 PubMed 17961033
- 66 Norden AD, Drappatz J, Wen PY. Advances in meningioma therapy.. Curr Neurol Neurosci Rep 2009; 9 (3) 231-240 PubMed 19348712
- 67 Sioka C, Kyritsis AP. Chemotherapy, hormonal therapy, and immunotherapy for recurrent meningiomas.. J Neurooncol 2009; 92 (1) 1-6 PubMed 19023520
- 68 Goldsmith B, McDermott MW. Meningioma.. Neurosurg Clin N Am 2006; 17 (2) 111-120, vi PubMed 16793503
- 69 Markwalder T-M, Seiler RW, Zava DT. Antiestrogenic therapy of meningiomas—a pilot study.. Surg Neurol 1985; 24 (3) 245-249 PubMed 4023903
- 70 Goodwin JW, Crowley J, Eyre HJ, Stafford B, Jaeckle KA, Townsend JJ. A phase II evaluation of tamoxifen in unresectable or refractory meningiomas: a Southwest Oncology Group study.. J Neurooncol 1993; 15 (1) 75-77 PubMed 8455065
- 71 Grunberg SM, Weiss MH. Lack of efficacy of megestrol acetate in the treatment of unresectable meningioma.. J Neurooncol 1990; 8 (1) 61-65 PubMed 2319292
- 72 Grunberg SM, Weiss MH, Spitz IM et al. Treatment of unresectable meningiomas with the antiprogesterone agent mifepristone.. J Neurosurg 1991; 74 (6) 861-866 PubMed 2033444
- 73 Grunberg SM, Weiss MH, Russell CA et al. Long-term administration of mifepristone (RU486): clinical tolerance during extended treatment of meningioma.. Cancer Invest 2006; 24 (8) 727-733 PubMed 17162554
- 74 Jääskeläinen J, Laasonen E, Kärkkäinen J, Haltia M, Troupp H. Hormone treatment of meningiomas: lack of response to medroxyprogesterone acetate (MPA). A pilot study of five cases.. Acta Neurochir (Wien) 1986; 80 (1–2) 35-41 PubMed 2939693
- 75 Touat M, Lombardi G, Farina P, Kalamarides M, Sanson M. Successful treatment of multiple intracranial meningiomas with the antiprogesterone receptor agent mifepristone (RU486).. Acta Neurochir (Wien) 2014; 156 (10) 1831-1835 PubMed 25078073
- 76 Chamberlain MC, Glantz MJ, Fadul CE. Recurrent meningioma: salvage therapy with long-acting somatostatin analogue.. Neurology 2007; 69 (10) 969-973 PubMed 17785665
- 77 Johnson DR, Kimmel DW, Burch PA et al. Phase II study of subcutaneous octreotide in adults with recurrent or progressive meningioma and meningeal hemangiopericytoma.. Neuro-oncol 2011; 13 (5) 530-535 PubMed 21558077
- 78 Simó M, Argyriou AA, Macià M et al. Recurrent high-grade meningioma: a phase II trial with somatostatin analogue therapy.. Cancer Chemother Pharmacol 2014; 73 (5) 919-923 PubMed 24619496
- 79 Gerster-Gilliéron K, Forrer F, Maecke H, Mueller-Brand J, Merlo A, Cordier D. 90Y-DOTATOC as therapeutic option for complex recurrent or progressive meningiomas.. J Nucl Med 2015; 56 (11) 1748-1751 PubMed 26294303
- 80 Marincek N, Radojewski P, Dumont RA et al. Somatostatin receptor-targeted radiopeptide therapy with 90Y-DOTATOC and 177Lu-DOTATOC in progressive meningioma: long-term results of a phase II clinical trial.. J Nucl Med 2015; 56 (2) 171-176 PubMed 25593116
- 81 Chamberlain MC, Glantz MJ. Interferon-alpha for recurrent World Health Organization grade 1 intracranial meningiomas.. Cancer 2008; 113 (8) 2146-2151 PubMed 18756531
- 82 Chamberlain MC. IFN-α for recurrent surgery- and radiation-refractory high-grade meningioma: a retrospective case series.. CNS Oncol 2013; 2 (3) 227-235 PubMed 25054463
- 83 Wen PY, Quant E, Drappatz J, Beroukhim R, Norden AD. Medical therapies for meningiomas.. J Neurooncol 2010; 99 (3) 365-378 PubMed 20820875
- 84 Mordechai O, Postovsky S, Vlodavsky E et al. Metastatic rhabdoid meningioma with BRAF V600E mutation and good response to personalized therapy: case report and review of the literature.. Pediatr Hematol Oncol 2015; 32 (3) 207-211 PubMed 25116269
- 85 Gelerstein E, Berger A, Jonas-Kimchi T et al. Regression of intracranial meningioma following treatment with nivolumab: case report and review of the literature.. J Clin Neurosci 2017; 37: 51-53 PubMed 28089420
- 86 Johnson M, Toms S. Mitogenic signal transduction pathways in meningiomas: novel targets for meningioma chemotherapy?. J Neuropathol Exp Neurol 2005; 64 (12) 1029-1036 PubMed 16319713
- 87 Simon M, Boström JP, Hartmann C. Molecular genetics of meningiomas: from basic research to potential clinical applications.. Neurosurgery 2007; 60 (5) 787-798 PubMed 17460514
- 88 Norden AD, Raizer JJ, Abrey LE et al. Phase II trials of erlotinib or gefitinib in patients with recurrent meningioma.. J Neurooncol 2010; 96 (2) 211-217 PubMed 19562255
- 89 Chamberlain MC. Is there effective systemic therapy for recurrent surgery- and radiation-refractory meningioma?. CNS Oncol 2013; 2 (1) 1-5 PubMed 25054350
- 90 Pietras K, Sjöblom T, Rubin K, Heldin CH, Ostman A. PDGF receptors as cancer drug targets.. Cancer Cell 2003; 3 (5) 439-443 PubMed 12781361
- 91 Johnson MD, Woodard A, Kim P, Frexes-Steed M. Evidence for mitogen-associated protein kinase activation and transduction of mitogenic signals by platelet-derived growth factor in human meningioma cells.. J Neurosurg 2001; 94 (2) 293-300 PubMed 11213968
- 92 Todo T, Adams EF, Fahlbusch R, Dingermann T, Werner H. Autocrine growth stimulation of human meningioma cells by platelet-derived growth factor.. J Neurosurg 1996; 84 (5) 852-858, discussion 858–859 PubMed 8622161
- 93 Wen PY, Yung WKA, Lamborn KR et al. Phase II study of imatinib mesylate for recurrent meningiomas (North American Brain Tumor Consortium study 01–08).. Neuro-oncol 2009; 11 (6) 853-860 PubMed 19293394
- 94 Reardon DA, Norden AD, Desjardins A et al. Phase II study of Gleevec® plus hydroxyurea (HU) in adults with progressive or recurrent meningioma.. J Neurooncol 2012; 106 (2) 409-415 PubMed 21938530
- 95 Mazza E, Brandes A, Zanon S et al. Hydroxyurea with or without imatinib in the treatment of recurrent or progressive meningiomas: a randomized phase II trial by Gruppo Italiano Cooperativo di Neuro-Oncologia (GICNO).. Cancer Chemother Pharmacol 2016; 77 (1) 115-120 PubMed 26659583
- 96 Nassehi D, Dyrbye H, Andresen M et al. Vascular endothelial growth factor A protein level and gene expression in intracranial meningiomas with brain edema.. APMIS 2011; 119 (12) 831-843 PubMed 22085359
- 97 Preusser M, Hassler M, Birner P et al. Microvascularization and expression of VEGF and its receptors in recurring meningiomas: pathobiological data in favor of anti-angiogenic therapy approaches.. Clin Neuropathol 2012; 31 (5) 352-360 PubMed 22541785
- 98 Baumgarten P, Brokinkel B, Zinke J et al. Expression of vascular endothelial growth factor (VEGF) and its receptors VEGFR1 and VEGFR2 in primary and recurrent WHO grade III meningiomas.. Histol Histopathol 2013; 28 (9) 1157-1166 PubMed 23475388
- 99 Lou E, Sumrall AL, Turner S et al. Bevacizumab therapy for adults with recurrent/progressive meningioma: a retrospective series.. J Neurooncol 2012; 109 (1) 63-70 PubMed 22535433
- 100 Nayak L, Iwamoto FM, Rudnick JD et al. Atypical and anaplastic meningiomas treated with bevacizumab.. J Neurooncol 2012; 109 (1) 187-193 PubMed 22544653
- 101 Kaley TJ, Wen P, Schiff D et al. Phase II trial of sunitinib for recurrent and progressive atypical and anaplastic meningioma.. Neuro-oncol 2015; 17 (1) 116-121 PubMed 25100872
- 102 Raizer JJ, Grimm SA, Rademaker A et al. A phase II trial of PTK787/ZK 222584 in recurrent or progressive radiation and surgery refractory meningiomas.. J Neurooncol 2014; 117 (1) 93-101 PubMed 24449400
- 103 Nunes FP, Merker VL, Jennings D et al. Bevacizumab treatment for meningiomas in NF2: a retrospective analysis of 15 patients.. PLoS One 2013; 8 (3) e59941 PubMed 23555840
- 104 Alanin MC, Klausen C, Caye-Thomasen P et al. Effect of bevacizumab on intracranial meningiomas in patients with neurofibromatosis type 2—a retrospective case series.. Int J Neurosci 2016; 126 (11) 1002-1006 PubMed 26365467
- 105 Grimm S, Kumthekar P, Chamberlain M et al. Phase II trial of bevacizumab in patients with surgery and radiation refractory progressive meningioma.. Neuro-Oncol 2015; 17 (Suppl 5) v130. (abstract MNGO-04) PubMed 26365467
- 106 Furtner J, Schöpf V, Seystahl K et al. Kinetics of tumor size and peritumoral brain edema before, during, and after systemic therapy in recurrent WHO grade II or III meningioma.. Neuro-oncol 2016; 18 (3) 401-407 PubMed 26354929
- 107 Pachow D, Andrae N, Kliese N et al. mTORC1 inhibitors suppress meningioma growth in mouse models.. Clin Cancer Res 2013; 19 (5) 1180-1189 PubMed 23406776
- 108 Graillon T, Defilles C, Mohamed A et al. Combined treatment by octreotide and everolimus: octreotide enhances inhibitory effect of everolimus in aggressive meningiomas.. J Neurooncol 2015; 124 (1) 33-43 PubMed 26015296
- 109 Koschny R, Boehm C, Sprick MR et al. Bortezomib sensitizes primary meningioma cells to TRAIL-induced apoptosis by enhancing formation of the death-inducing signaling complex.. J Neuropathol Exp Neurol 2014; 73 (11) 1034-1046 PubMed 25289891
- 110 Domingues P, González-Tablas M, Otero Á et al. Genetic/molecular alterations of meningiomas and the signaling pathways targeted.. Oncotarget 2015; 6 (13) 10671-10688 PubMed 25965831
- 111 Shah NR, Tancioni I, Ward KK et al. Analyses of merlin/NF2 connection to FAK inhibitor responsiveness in serous ovarian cancer.. Gynecol Oncol 2014; 134 (1) 104-111 PubMed 24786638
- 112 Du Z, Abedalthagafi M, Aizer AA et al. Increased expression of the immune modulatory molecule PD-L1 (CD274) in anaplastic meningioma.. Oncotarget 2015; 6 (7) 4704-4716 PubMed 25609200
- 113 Han SJ, Reis G, Kohanbash G et al. Expression and prognostic impact of immune modulatory molecule PD-L1 in meningioma.. J Neurooncol 2016; 130 (3) 543-552 PubMed 27624915
- 114 McMaster ML, Goldstein AM, Bromley CM, Ishibe N, Parry DM. Chordoma: incidence and survival patterns in the United States, 1973–1995.. Cancer Causes Control 2001; 12 (1) 1-11 PubMed 11227920
- 115 Rassekh CH, Nuss DW, Kapadia SB, Curtin HD, Weissman JL, Janecka IP. Chondrosarcoma of the nasal septum: skull base imaging and clinicopathologic correlation.. Otolaryngol Head Neck Surg 1996; 115 (1) 29-37 PubMed 8758626
- 116 Meyers SP, Hirsch Jr WL, Curtin HD, Barnes L, Sekhar LN, Sen C. Chondrosarcomas of the skull base: MR imaging features.. Radiology 1992; 184 (1) 103-108 PubMed 1609064
- 117 Doucet V, Peretti-Viton P, Figarella-Branger D, Manera L, Salamon G. MRI of intracranial chordomas. Extent of tumour and contrast enhancement: criteria for differential diagnosis.. Neuroradiology 1997; 39 (8) 571-576 PubMed 9272494
- 118 Youssef C, Aoun SG, Moreno JR, Bagley CA. Recent advances in understanding and managing chordomas.. F1000 Res 2016; 5: 2902 PubMed 28105324
- 119 Gulluoglu S, Turksoy O, Kuskucu A, Ture U, Bayrak OF. The molecular aspects of chordoma.. Neurosurg Rev 2016; 39 (2) 185-196 PubMed 26363792
- 120 Lipplaa A, Dijkstra S, Gelderblom H. Efficacy of pazopanib and sunitinib in advanced axial chordoma: a single reference centre case series.. Clin Sarcoma Res 2016; 6: 19 PubMed 27822356
- 121 Di Maio S, Temkin N, Ramanathan D, Sekhar LN. Current comprehensive management of cranial base chordomas: 10-year meta-analysis of observational studies.. J Neurosurg 2011; 115 (6) 1094-1105 PubMed 21819197
- 122 Yasuda M, Bresson D, Chibbaro S et al. Chordomas of the skull base and cervical spine: clinical outcomes associated with a multimodal surgical resection combined with proton-beam radiation in 40 patients.. Neurosurg Rev 2012; 35 (2) 171-182, discussion 182–183 PubMed 21863225
- 123 McMaster M. Update on the epidemiology of chordoma: SEER registry data 1973–2007. Poster presented at: Third International Chordoma Research Workshop, March 17–19, 2011; Bethesda, MD
- 124 Adson AW, Kernohan JW, Woltman HW. Cranial and cervical chordomas—a clinical and histologic study.. Arch Neurol Psychiatry 1935; 33 (2) 247-261 NOT_FOUND
- 125 Hruban RH, Traganos F, Reuter VE, Huvos AG. Chordomas with malignant spindle cell components: a DNA flow cytometric and immunohistochemical study with histogenetic implications.. Am J Pathol 1990; 137 (2) 435-447 PubMed 1696786
- 126 Mitchell A, Scheithauer BW, Unni KK, Forsyth PJ, Wold LE, McGivney DJ. Chordoma and chondroid neoplasms of the spheno-occiput: an immunohistochemical study of 41 cases with prognostic and nosologic implications.. Cancer 1993; 72 (10) 2943-2949 PubMed 7693324
- 127 Abenoza P, Sibley RK. Chordoma: an immunohistologic study.. Hum Pathol 1986; 17 (7) 744-747 PubMed 2424820
- 128 Meis JM, Giraldo AA. Chordoma: an immunohistochemical study of 20 cases.. Arch Pathol Lab Med 1988; 112 (5) 553-556 PubMed 2451900
- 129 Vujovic S, Henderson S, Presneau N et al. Brachyury, a crucial regulator of notochordal development, is a novel biomarker for chordomas.. J Pathol 2006; 209 (2) 157-165 PubMed 16538613
- 130 Song W, Gobe GC. Understanding molecular pathways and targets of brachyury in epithelial-mesenchymal transition (EMT) in human cancers.. Curr Cancer Drug Targets 2016; 16 (7) 586-593 PubMed 27018266
- 131 Tirabosco R, Mangham DC, Rosenberg AE et al. Brachyury expression in extra-axial skeletal and soft tissue chordomas: a marker that distinguishes chordoma from mixed tumor/myoepithelioma/parachordoma in soft tissue.. Am J Surg Pathol 2008; 32 (4) 572-580 PubMed 18301055
- 132 Oakley GJ, Fuhrer K, Seethala RR. Brachyury, SOX-9, and podoplanin, new markers in the skull base chordoma vs chondrosarcoma differential: a tissue microarray-based comparative analysis.. Mod Pathol 2008; 21 (12) 1461-1469 PubMed 18820665
- 133 Schwab JH, Boland PJ, Agaram NP et al. Chordoma and chondrosarcoma gene profile: implications for immunotherapy.. Cancer Immunol Immunother 2009; 58 (3) 339-349 PubMed 18641983
- 134 Terahara A, Niemierko A, Goitein M et al. Analysis of the relationship between tumor dose inhomogeneity and local control in patients with skull base chordoma.. Int J Radiat Oncol Biol Phys 1999; 45 (2) 351-358 PubMed 10487555
- 135 Catton C, O’Sullivan B, Bell R et al. Chordoma: long-term follow-up after radical photon irradiation.. Radiother Oncol 1996; 41 (1) 67-72 PubMed 8961370
- 136 Stacchiotti S, Sommer J, . Building a global consensus approach to chordoma: a position paper from the medical and patient community.. Lancet Oncol 2015; 16 (2) e71-e83 PubMed 25638683
- 137 Di Maio S, Rostomily R, Sekhar LN. Current surgical outcomes for cranial base chordomas: cohort study of 95 patients.. Neurosurgery 2012; 70 (6) 1355-1360, discussion 1360 PubMed 22157545
- 138 Chibbaro S, Cornelius JF, Froelich S et al. Endoscopic endonasal approach in the management of skull base chordomas—clinical experience on a large series, technique, outcome, and pitfalls.. Neurosurg Rev 2014; 37 (2) 217-224, discussion 224–225 PubMed 24249430
- 139 Fernandez-Miranda JC, Gardner PA, Snyderman CH et al. Clival chordomas: a pathological, surgical, and radiotherapeutic review.. Head Neck 2014; 36 (6) 892-906 PubMed 23804541
- 140 Zhang Q, Kong F, Yan B, Ni Z, Liu H. Endoscopic endonasal surgery for clival chordoma and chondrosarcoma.. ORL J Otorhinolaryngol Relat Spec 2008; 70 (2) 124-129 PubMed 18408411
- 141 Fatemi N, Dusick JR, Gorgulho AA et al. Endonasal microscopic removal of clival chordomas.. Surg Neurol 2008; 69 (4) 331-338 PubMed 18234296
- 142 Saito K, Toda M, Tomita T, Ogawa K, Yoshida K. Surgical results of an endoscopic endonasal approach for clival chordomas.. Acta Neurochir (Wien) 2012; 154 (5) 879-886 PubMed 22402876
- 143 Stacchiotti S, Gronchi A, Fossati P et al. Best practices for the management of local-regional recurrent chordoma: a position paper by the Chordoma Global Consensus Group.. Ann Oncol 2017; 28 (6) 1230-1242 PubMed 28184416
- 144 Di Maio S, Yip S, Al Zhrani GA et al. Novel targeted therapies in chordoma: an update.. Ther Clin Risk Manag 2015; 11: 873-883 PubMed 26097380
- 145 Di Maio S, Kong E, Yip S, Rostomily R. Converging paths to progress for skull base chordoma: review of current therapy and future molecular targets.. Surg Neurol Int 2013; 4: 72 PubMed 23776758
- 146 Tzortzidis F, Elahi F, Wright D, Natarajan SK, Sekhar LN. Patient outcome at long-term follow-up after aggressive microsurgical resection of cranial base chordomas.. Neurosurgery 2006; 59 (2) 230-237 PubMed 16883163
- 147 Weber DC, Malyapa R, Albertini F et al. Long term outcomes of patients with skull-base low-grade chondrosarcoma and chordoma patients treated with pencil beam scanning proton therapy.. Radiother Oncol 2016; 120 (1) 169-174 PubMed 27247057
- 148 Matloob SA, Nasir HA, Choi D. Proton beam therapy in the management of skull base chordomas: systematic review of indications, outcomes, and implications for neurosurgeons.. Br J Neurosurg 2016; 30 (4) 382-387 PubMed 27173123
- 149 Schulz-Ertner D, Karger CP, Feuerhake A et al. Effectiveness of carbon ion radiotherapy in the treatment of skull-base chordomas.. Int J Radiat Oncol Biol Phys 2007; 68 (2) 449-457 PubMed 17363188
- 150 Sahgal A, Chan MW, Atenafu EG et al. Image-guided, intensity-modulated radiation therapy (IG-IMRT) for skull base chordoma and chondrosarcoma: preliminary outcomes.. Neuro-oncol 2015; 17 (6) 889-894 PubMed 25543126
- 151 Colia V, Stacchiotti S. Medical treatment of advanced chordomas.. Eur J Cancer 2017; 83: 220-228 PubMed 28750274
- 152 Kitamura Y, Sasaki H, Kimura T et al. Molecular and clinical risk factors for recurrence of skull base chordomas: gain on chromosome 2p, expression of brachyury, and lack of irradiation negatively correlate with patient prognosis.. J Neuropathol Exp Neurol 2013; 72 (9) 816-823 PubMed 23965741
- 153 Miettinen M, Wang Z, Lasota J, Heery C, Schlom J, Palena C. Nuclear brachyury expression is consistent in chordoma, common in germ cell tumors and small cell carcinomas, and rare in other carcinomas and sarcomas: an immunohistochemical study of 5229 cases.. Am J Surg Pathol 2015; 39 (10) 1305-1312 PubMed 26099010
- 154 Palena C, Roselli M, Litzinger MT et al. Overexpression of the EMT driver brachyury in breast carcinomas: association with poor prognosis.. J Natl Cancer Inst 2014; 106 (5) 106 pii PubMed 24815864
- 155 Du R, Wu S, Lv X, Fang H, Wu S, Kang J. Overexpression of brachyury contributes to tumor metastasis by inducing epithelial-mesenchymal transition in hepatocellular carcinoma.. J Exp Clin Cancer Res 2014; 33: 105 PubMed 25499255
- 156 Yoshihama R, Yamaguchi K, Imajyo I et al. Expression levels of SOX2, KLF4 and brachyury transcription factors are associated with metastasis and poor prognosis in oral squamous cell carcinoma.. Oncol Lett 2016; 11 (2) 1435-1446 PubMed 26893757
- 157 Zhang L, Guo S, Schwab JH et al. Tissue microarray immunohistochemical detection of brachyury is not a prognostic indicator in chordoma.. PLoS One 2013; 8 (9) e75851 PubMed 24086644
- 158 Yang C, Hornicek FJ, Wood KB et al. Characterization and analysis of human chordoma cell lines.. Spine 2010; 35 (13) 1257-1264 PubMed 20461036
- 159 Rinner B, Froehlich EV, Buerger K et al. Establishment and detailed functional and molecular genetic characterisation of a novel sacral chordoma cell line, MUG-Chor1.. Int J Oncol 2012; 40 (2) 443-451 PubMed 22002331
- 160 Diaz RJ, Guduk M, Romagnuolo R et al. High-resolution whole-genome analysis of skull base chordomas implicates FHIT loss in chordoma pathogenesis.. Neoplasia 2012; 14 (9) 788-798 PubMed 23019410
- 161 Horbinski C, Oakley GJ, Cieply K et al. The prognostic value of Ki-67, p53, epidermal growth factor receptor, 1p36, 9p21, 10q23, and 17p13 in skull base chordomas.. Arch Pathol Lab Med 2010; 134 (8) 1170-1176 PubMed 20670138
- 162 Presneau N, Shalaby A, Idowu B et al. Potential therapeutic targets for chordoma: PI3K/AKT/TSC1/TSC2/mTOR pathway.. Br J Cancer 2009; 100 (9) 1406-1414 PubMed 19401700
- 163 Shalaby A, Presneau N, Ye H et al. The role of epidermal growth factor receptor in chordoma pathogenesis: a potential therapeutic target.. J Pathol 2011; 223 (3) 336-346 PubMed 21171079
- 164 Sommer J, Itani DM, Homlar KC et al. Methylthioadenosine phosphorylase and activated insulin-like growth factor-1 receptor/insulin receptor: potential therapeutic targets in chordoma.. J Pathol 2010; 220 (5) 608-617 PubMed 20140939
- 165 Fasig JH, Dupont WD, LaFleur BJ, Olson SJ, Cates JM. Immunohistochemical analysis of receptor tyrosine kinase signal transduction activity in chordoma.. Neuropathol Appl Neurobiol 2008; 34 (1) 95-104 PubMed 17973908
- 166 Weinberger PM, Yu Z, Kowalski D et al. Differential expression of epidermal growth factor receptor, c-Met, and HER2/neu in chordoma compared with 17 other malignancies.. Arch Otolaryngol Head Neck Surg 2005; 131 (8) 707-711 PubMed 16103303
- 167 Ptaszyński K, Szumera-Ciećkiewicz A, Owczarek J et al. Epidermal growth factor receptor (EGFR) status in chordoma.. Pol J Pathol 2009; 60 (2) 81-87 PubMed 19886182
- 168 Tamborini E, Virdis E, Negri T et al. Analysis of receptor tyrosine kinases (RTKs) and downstream pathways in chordomas.. Neuro-oncol 2010; 12 (8) 776-789 PubMed 20164240
- 169 Tamborini E, Miselli F, Negri T et al. Molecular and biochemical analyses of platelet-derived growth factor receptor (PDGFR) B, PDGFRA, and KIT receptors in chordomas.. Clin Cancer Res 2006; 12 (23) 6920-6928 PubMed 17145809
- 170 Casali PG, Messina A, Stacchiotti S et al. Imatinib mesylate in chordoma.. Cancer 2004; 101 (9) 2086-2097 PubMed 15372471
- 171 Orzan F, Terreni MR, Longoni M et al. Expression study of the target receptor tyrosine kinase of Imatinib mesylate in skull base chordomas.. Oncol Rep 2007; 18 (1) 249-252 PubMed 17549375
- 172 Li X, Ji Z, Ma Y, Qiu X, Fan Q, Ma B. Expression of hypoxia-inducible factor-1α, vascular endothelial growth factor and matrix metalloproteinase-2 in sacral chordomas.. Oncol Lett 2012; 3 (6) 1268-1274 PubMed 22783431
- 173 Chen KW, Yang HL, Lu J et al. Expression of vascular endothelial growth factor and matrix metalloproteinase-9 in sacral chordoma.. J Neurooncol 2011; 101 (3) 357-363 PubMed 20549300
- 174 Scheipl S, Lohberger B, Rinner B et al. Histone deacetylase inhibitors as potential therapeutic approaches for chordoma: an immunohistochemical and functional analysis.. J Orthop Res 2013; 31 (12) 1999-2005 PubMed 23893747
- 175 Zhang Y, Schiff D, Park D, Abounader R. MicroRNA-608 and microRNA-34a regulate chordoma malignancy by targeting EGFR, Bcl-xL and MET.. PLoS One 2014; 9 (3) e91546 PubMed 24621885
- 176 Osaka E, Kelly AD, Spentzos D et al. MicroRNA-155 expression is independently predictive of outcome in chordoma.. Oncotarget 2015; 6 (11) 9125-9139 PubMed 25823817
- 177 Osaka E, Yang X, Shen JK et al. MicroRNA-1 (miR-1) inhibits chordoma cell migration and invasion by targeting slug.. J Orthop Res 2014; 32 (8) 1075-1082 PubMed 24760686
- 178 Duan Z, Shen J, Yang X et al. Prognostic significance of miRNA-1 (miR-1) expression in patients with chordoma.. J Orthop Res 2014; 32 (5) 695-701 PubMed 24501096
- 179 Duan Z, Choy E, Nielsen GP et al. Differential expression of microRNA (miRNA) in chordoma reveals a role for miRNA-1 in Met expression.. J Orthop Res 2010; 28 (6) 746-752 PubMed 20041488
- 180 Hsu W, Mohyeldin A, Shah SR et al. Generation of chordoma cell line JHC7 and the identification of brachyury as a novel molecular target.. J Neurosurg 2011; 115: 760-769 PubMed 20041488
- 181 Hu Y, Mintz A, Shah SR, Quinones-Hinojosa A, Hsu W. The FGFR/MEK/ERK/brachyury pathway is critical for chordoma cell growth and survival.. Carcinogenesis 2014; 35 (7) 1491-1499 PubMed 24445144
- 182 Nelson AC, Pillay N, Henderson S et al. An integrated functional genomics approach identifies the regulatory network directed by brachyury (T) in chordoma.. J Pathol 2012; 228 (3) 274-285 PubMed 22847733
- 183 Heery CS, Marte H, Madan J et al. NCI experience using yeast-brachyury vaccine (GI-6301) in patients (pts) with advanced chordoma.. J Clin Oncol 2014; 32: 3081-3081 NOT_FOUND
- 184 Schwab J, Antonescu C, Boland P et al. Combination of PI3K/mTOR inhibition demonstrates efficacy in human chordoma.. Anticancer Res 2009; 29 (6) 1867-1871 PubMed 19528441
- 185 Stacchiotti S, Marrari A, Tamborini E et al. Response to imatinib plus sirolimus in advanced chordoma.. Ann Oncol 2009; 20 (11) 1886-1894 PubMed 19570961
- 186 Han S, Polizzano C, Nielsen GP, Hornicek FJ, Rosenberg AE, Ramesh V. Aberrant hyperactivation of akt and mammalian target of rapamycin complex 1 signaling in sporadic chordomas.. Clin Cancer Res 2009; 15 (6) 1940-1946 PubMed 19276265
- 187 Davies JM, Robinson AE, Cowdrey C et al. Generation of a patient-derived chordoma xenograft and characterization of the phosphoproteome in a recurrent chordoma.. J Neurosurg 2014; 120 (2) 331-336 PubMed 24286145
- 188 Ricci-Vitiani L, Runci D, D’Alessandris QG et al. Chemotherapy of skull base chordoma tailored on responsiveness of patient-derived tumor cells to rapamycin.. Neoplasia 2013; 15 (7) 773-782 PubMed 23814489
- 189 Shrager J, Tenenbaum JM. Rapid learning for precision oncology.. Nat Rev Clin Oncol 2014; 11 (2) 109-118 PubMed 24445514
- 190 Stacchiotti S, Longhi A, Ferraresi V et al. Phase II study of imatinib in advanced chordoma.. J Clin Oncol 2012; 30 (9) 914-920 PubMed 22331945
- 191 Hindi N, Casali PG, Morosi C et al. Imatinib in advanced chordoma: a retrospective case series analysis.. Eur J Cancer 2015; 51 (17) 2609-2614 PubMed 26283036
- 192 Bompas E, Le Cesne A, Tresch-Bruneel E et al. Sorafenib in patients with locally advanced and metastatic chordomas: a phase II trial of the French Sarcoma Group (GSF/GETO).. Ann Oncol 2015; 26 (10) 2168-2173 PubMed 26202596
- 193 Stacchiotti S, Tamborini E, Lo Vullo S et al. Phase II study on lapatinib in advanced EGFR-positive chordoma.. Ann Oncol 2013; 24 (7) 1931-1936 PubMed 23559153
- 194 Hof H, Welzel T, Debus J. Effectiveness of cetuximab/gefitinib in the therapy of a sacral chordoma.. Onkologie 2006; 29 (12) 572-574 PubMed 17202828
- 195 Lindén O, Stenberg L, Kjellén E. Regression of cervical spinal cord compression in a patient with chordoma following treatment with cetuximab and gefitinib.. Acta Oncol 2009; 48 (1) 158-159 PubMed 18752082
- 196 Launay SG, Chetaille B, Medina F et al. Efficacy of epidermal growth factor receptor targeting in advanced chordoma: case report and literature review.. BMC Cancer 2011; 11: 423 PubMed 21970335
- 197 Singhal N, Kotasek D, Parnis FX. Response to erlotinib in a patient with treatment refractory chordoma.. Anticancer Drugs 2009; 20 (10) 953-955 PubMed 19730087
- 198 Asklund T, Danfors T, Henriksson R. PET response and tumor stabilization under erlotinib and bevacizumab treatment of an intracranial lesion non-invasively diagnosed as likely chordoma.. Clin Neuropathol 2011; 30 (5) 242-246 PubMed 21955928
- 199 Yang C, Schwab JH, Schoenfeld AJ et al. A novel target for treatment of chordoma: signal transducers and activators of transcription 3.. Mol Cancer Ther 2009; 8 (9) 2597-2605 PubMed 19723879
- 200 Lubin M, Lubin A. Selective killing of tumors deficient in methylthioadenosine phosphorylase: a novel strategy.. PLoS One 2009; 4 (5) e5735 PubMed 19478948
- 201 Laws Jr ER. Transsphenoidal microsurgery in the management of craniopharyngioma.. J Neurosurg 1980; 52 (5) 661-666 PubMed 7373393
- 202 Northfield DW. Rathke-pouch tumours.. Brain 1957; 80 (3) 293-312 PubMed 13471802
- 203 Rush JA, Younge BR, Campbell RJ, MacCarty CS. Optic glioma: long-term follow-up of 85 histopathologically verified cases.. Ophthalmology 1982; 89 (11) 1213-1219 PubMed 6818504
- 204 Bollati A, Giunta F, Lenzi A, Marini G. Third ventricle intrinsic craniopharingioma: case report.. J Neurosurg Sci 1974; 18 (3) 216-219 PubMed 4465421
- 205 Cashion EL, Young JM. Intraventricular craniopharyngioma: report of two cases.. J Neurosurg 1971; 34 (1) 84-87 PubMed 5539648
- 206 Bunin GR, Surawicz TS, Witman PA, Preston-Martin S, Davis F, Bruner JM. The descriptive epidemiology of craniopharyngioma.. J Neurosurg 1998; 89 (4) 547-551 PubMed 9761047
- 207 Louis DN, Ohgaki H, Wiestler OD, Cavenee WK, eds. Craniopharyngioma. In: World Health Organization Classification of Tumours of the Nervous System, Editorial and Consensus Conference Working Group, Lyon, France: IARC Press; 2007
- 208 Weiner HL, Wisoff JH, Rosenberg ME et al. Craniopharyngiomas: a clinicopathological analysis of factors predictive of recurrence and functional outcome.. Neurosurgery 1994; 35 (6) 1001-1010, discussion 1010–1011 PubMed 7885544
- 209 Crotty TB, Scheithauer BW, Young Jr WF et al. Papillary craniopharyngioma: a clinicopathological study of 48 cases.. J Neurosurg 1995; 83 (2) 206-214 PubMed 7616262
- 210 Minniti G, Esposito V, Amichetti M, Enrici RM. The role of fractionated radiotherapy and radiosurgery in the management of patients with craniopharyngioma.. Neurosurg Rev 2009; 32 (2) 125-132 PubMed 19165514
- 211 Combs SE, Thilmann C, Huber PE, Hoess A, Debus J, Schulz-Ertner D. Achievement of long-term local control in patients with craniopharyngiomas using high precision stereotactic radiotherapy.. Cancer 2007; 109 (11) 2308-2314 PubMed 17469176
- 212 Kobayashi T. Long-term results of Gamma Knife radiosurgery for 100 consecutive cases of craniopharyngioma and a treatment strategy.. Prog Neurol Surg 2009; 22: 63-76 PubMed 18948720
- 213 Niranjan A, Kano H, Mathieu D, Kondziolka D, Flickinger JC, Lunsford LD. Radiosurgery for craniopharyngioma.. Int J Radiat Oncol Biol Phys 2010; 78 (1) 64-71 PubMed 20005637
- 214 Fitzek MM, Linggood RM, Adams J, Munzenrider JE. Combined proton and photon irradiation for craniopharyngioma: long-term results of the early cohort of patients treated at Harvard Cyclotron Laboratory and Massachusetts General Hospital.. Int J Radiat Oncol Biol Phys 2006; 64 (5) 1348-1354 PubMed 16580494
- 215 Luu QT, Loredo LN, Archambeau JO, Yonemoto LT, Slater JM, Slater JD. Fractionated proton radiation treatment for pediatric craniopharyngioma: preliminary report.. Cancer J 2006; 12 (2) 155-159 PubMed 16630407
- 216 Bishop AJ, Greenfield B, Mahajan A et al. Proton beam therapy versus conformal photon radiation therapy for childhood craniopharyngioma: multi-institutional analysis of outcomes, cyst dynamics, and toxicity.. Int J Radiat Oncol Biol Phys 2014; 90 (2) 354-361 PubMed 25052561
- 217 McMurry FG, Hardy Jr RW, Dohn DF, Sadar E, Gardner WJ. Long term results in the management of craniopharyngiomas.. Neurosurgery 1977; 1 (3) 238-241 PubMed 615967
- 218 Ignelzi RJ, Squire LR. Recovery from anterograde and retrograde amnesia after percutaneous drainage of a cystic craniopharyngioma.. J Neurol Neurosurg Psychiatry 1976; 39 (12) 1231-1235 PubMed 1011035
- 219 Gutin PH, Klemme WM, Lagger RL, MacKay AR, Pitts LH, Hosobuchi Y. Management of the unresectable cystic craniopharyngioma by aspiration through an Ommaya reservoir drainage system.. J Neurosurg 1980; 52 (1) 36-40 PubMed 7350278
- 220 Manaka S, Teramoto A, Takakura K. The efficacy of radiotherapy for craniopharyngioma.. J Neurosurg 1985; 62 (5) 648-656 PubMed 3989587
- 221 Huk WJ, Mahlstedt J. Intracystic radiotherapy (90Y) of craniopharyngiomas: CT-guided stereotaxic implantation of indwelling drainage system.. AJNR Am J Neuroradiol 1983; 4 (3) 803-806 PubMed 6410858
- 222 Julow J, Lányi F, Hajda M et al. The radiotherapy of cystic craniopharyngioma with intracystic installation of 90Y silicate colloid.. Acta Neurochir (Wien) 1985; 74 (3–4) 94-99 PubMed 3984798
- 223 Voges J, Sturm V, Lehrke R, Treuer H, Gauss C, Berthold F. Cystic craniopharyngioma: long-term results after intracavitary irradiation with stereotactically applied colloidal beta-emitting radioactive sources.. Neurosurgery 1997; 40 (2) 263-269, discussion 269–270 PubMed 9007857
- 224 Pollock BE, Lunsford LD, Kondziolka D, Levine G, Flickinger JC. Phosphorus-32 intracavitary irradiation of cystic craniopharyngiomas: current technique and long-term results.. Int J Radiat Oncol Biol Phys 1995; 33 (2) 437-446 PubMed 7673031
- 225 Backlund EO, Johansson L, Sarby B. Studies on craniopharyngiomas. II. Treatment by stereotaxis and radiosurgery.. Acta Chir Scand 1972; 138 (8) 749-759 PubMed 4565719
- 226 Van den Berge JH, Blaauw G, Breeman WA, Rahmy A, Wijngaarde R. Intracavitary brachytherapy of cystic craniopharyngiomas.. J Neurosurg 1992; 77 (4) 545-550 PubMed 1527612
- 227 Barriger RB, Chang A, Lo SS et al. Phosphorus-32 therapy for cystic craniopharyngiomas.. Radiother Oncol 2011; 98 (2) 207-212 PubMed 21269713
- 228 Zhang S, Fang Y, Cai BW, Xu JG, You C. Intracystic bleomycin for cystic craniopharyngiomas in children.. Cochrane Database Syst Rev 2016; 7: CD008890 PubMed 27416004
- 229 Hukin J, Steinbok P, Lafay-Cousin L et al. Intracystic bleomycin therapy for craniopharyngioma in children: the Canadian experience.. Cancer 2007; 109 (10) 2124-2131 PubMed 17407137
- 230 Olsson DS, Andersson E, Bryngelsson IL, Nilsson AG, Johannsson G. Excess mortality and morbidity in patients with craniopharyngioma, especially in patients with childhood onset: a population-based study in Sweden.. J Clin Endocrinol Metab 2015; 100 (2) 467-474 PubMed 25375987
- 231 Lo AC, Howard AF, Nichol A et al. Long-term outcomes and complications in patients with craniopharyngioma: the British Columbia Cancer Agency experience.. Int J Radiat Oncol Biol Phys 2014; 88 (5) 1011-1018 PubMed 24661653
- 232 Liu AK, Bagrosky B, Fenton LZ et al. Vascular abnormalities in pediatric craniopharyngioma patients treated with radiation therapy.. Pediatr Blood Cancer 2009; 52 (2) 227-230 PubMed 18937328
- 233 Enchev Y, Ferdinandov D, Kounin G, Encheva E, Bussarsky V. Radiation-induced gliomas following radiotherapy for craniopharyngiomas: a case report and review of the literature.. Clin Neurol Neurosurg 2009; 111 (7) 591-596 PubMed 19447544
- 234 Oikonomou E, Barreto DC, Soares B, De Marco L, Buchfelder M, Adams EF. Beta-catenin mutations in craniopharyngiomas and pituitary adenomas.. J Neurooncol 2005; 73 (3) 205-209 PubMed 15980970
- 235 Martinez-Gutierrez JC, D’Andrea MR, Cahill DP, Santagata S, Barker II FG, Brastianos PK. Diagnosis and management of craniopharyngiomas in the era of genomics and targeted therapy.. Neurosurg Focus 2016; 41 (6) E2 PubMed 27903124
- 236 Kato K, Nakatani Y, Kanno H et al. Possible linkage between specific histological structures and aberrant reactivation of the Wnt pathway in adamantinomatous craniopharyngioma.. J Pathol 2004; 203 (3) 814-821 PubMed 15221941
- 237 Sekine S, Shibata T, Kokubu A et al. Craniopharyngiomas of adamantinomatous type harbor beta-catenin gene mutations.. Am J Pathol 2002; 161 (6) 1997-2001 PubMed 12466115
- 238 Buslei R, Nolde M, Hofmann B et al. Common mutations of beta-catenin in adamantinomatous craniopharyngiomas but not in other tumours originating from the sellar region.. Acta Neuropathol 2005; 109 (6) 589-597 PubMed 15891929
- 239 Brastianos PK, Taylor-Weiner A, Manley PE et al. Exome sequencing identifies BRAF mutations in papillary craniopharyngiomas.. Nat Genet 2014; 46 (2) 161-165 PubMed 24413733
- 240 Larkin SJ, Preda V, Karavitaki N, Grossman A, Ansorge O. BRAF V600E mutations are characteristic for papillary craniopharyngioma and may coexist with CTNNB1-mutated adamantinomatous craniopharyngioma.. Acta Neuropathol 2014; 127 (6) 927-929 PubMed 24715106
- 241 Cavalheiro S, Di Rocco C, Valenzuela S et al. Craniopharyngiomas: intratumoral chemotherapy with interferon-alpha: a multicenter preliminary study with 60 cases.. Neurosurg Focus 2010; 28 (4) E12 PubMed 20367356
- 242 Cavalheiro S, Dastoli PA, Silva NS, Toledo S, Lederman H, da Silva MC. Use of interferon alpha in intratumoral chemotherapy for cystic craniopharyngioma.. Childs Nerv Syst 2005; 21 (8–9) 719-724 PubMed 16133276
- 243 Dastoli PA, Nicácio JM, Silva NS et al. Cystic craniopharyngioma: intratumoral chemotherapy with alpha interferon.. Arq Neuropsiquiatr 2011; 69 (1) 50-55 PubMed 21359423
- 244 Aylwin SJ, Bodi I, Beaney R. Pronounced response of papillary craniopharyngioma to treatment with vemurafenib, a BRAF inhibitor.. Pituitary 2016; 19 (5) 544-546 PubMed 26115708
- 245 Brastianos PK, Shankar GM, Gill CM et al. Dramatic response of BRAF V600E mutant papillary craniopharyngioma to targeted therapy.. J Natl Cancer Inst 2015; 108 (2) djv310 PubMed 26498373
- 246 Roque A, Odia Y. BRAF-V600E mutant papillary craniopharyngioma dramatically responds to combination BRAF and MEK inhibitors.. CNS Oncol 2017; 6 (2) 95-99 PubMed 28425764
- 247 Robert C, Karaszewska B, Schachter J et al. Improved overall survival in melanoma with combined dabrafenib and trametinib.. N Engl J Med 2015; 372 (1) 30-39 PubMed 25399551
- 248 Brastianos PK, Santagata S. Endocrine tumors: BRAF V600E mutations in papillary craniopharyngioma.. Eur J Endocrinol 2016; 174 (4) R139-R144 PubMed 26563980
- 249 Tritos NA. Is there a role for targeted medical therapies in patients with craniopharyngiomas?. Future Oncol 2015; 11 (24) 3221-3223 PubMed 26562628
- 250 Singh AS, Chawla NS, Chawla SP. Giant-cell tumor of bone: treatment options and role of denosumab.. Biologics 2015; 9: 69-74 PubMed 26203221
- 251 Guajardo JH, de la Peña C, Gonzalez MF et al. Giant cell tumour of the skull base treated with surgery, stereotactic radiosurgery and denosumab: case report and review of literature.. Open Access Library Journal. 2017; 4: e3571 NOT_FOUND;INVALID_JOURNAL
- 252 Murphey MD, Nomikos GC, Flemming DJ, Gannon FH, Temple HT, Kransdorf MJ. From the archives of AFIP. Imaging of giant cell tumor and giant cell reparative granuloma of bone: radiologic-pathologic correlation.. Radiographics 2001; 21 (5) 1283-1309 PubMed 11553835
- 253 Kwon JW, Chung HW, Cho EY et al. MRI findings of giant cell tumors of the spine.. AJR Am J Roentgenol 2007; 189 (1) 246-250 PubMed 17579178
- 254 Campanacci M, Baldini N, Boriani S, Sudanese A. Giant-cell tumor of bone.. J Bone Joint Surg Am 1987; 69 (1) 106-114 PubMed 3805057
- 255 Thomas DM. RANKL, denosumab, and giant cell tumor of bone.. Curr Opin Oncol 2012; 24 (4) 397-403 PubMed 22581354
- 256 Lacey DL, Boyle WJ, Simonet WS et al. Bench to bedside: elucidation of the OPG-RANK-RANKL pathway and the development of denosumab.. Nat Rev Drug Discov 2012; 11 (5) 401-419 PubMed 22543469
- 257 Roeder F, Timke C, Zwicker F, et al. Intensity modulated radiotherapy (IMRT) in benign giant cell tumors—a single institution case series and a short review of the literature. Thieke C, Bischof M, Debus J, Huber PE
- 258 Kim IY, Jung S, Jung TY et al. Gamma Knife radiosurgery for giant cell tumor of the petrous bone.. Clin Neurol Neurosurg 2012; 114 (2) 185-189 PubMed 22079411
- 259 Colia V, Provenzano S, Hindi N, Casali PG, Stacchiotti S. Systemic therapy for selected skull base sarcomas: chondrosarcoma, chordoma, giant cell tumour and solitary fibrous tumour/hemangiopericytoma.. Rep Pract Oncol Radiother 2016; 21 (4) 361-369 PubMed 27330421
- 260 Thomas D, Henshaw R, Skubitz K et al. Denosumab in patients with giant-cell tumour of bone: an open-label, phase 2 study.. Lancet Oncol 2010; 11 (3) 275-280 PubMed 20149736
- 261 Osaka S, Toriyama M, Taira K, Sano S, Saotome K. Analysis of giant cell tumor of bone with pulmonary metastases.. Clin Orthop Relat Res 1997; (335) 253-261 PubMed 9020226
- 262 Morgan T, Atkins GJ, Trivett MK et al. Molecular profiling of giant cell tumor of bone and the osteoclastic localization of ligand for receptor activator of nuclear factor kappaB.. Am J Pathol 2005; 167 (1) 117-128 PubMed 15972958
- 263 Schwartz HS, Jenkins RB, Dahl RJ, Dewald GW. Cytogenetic analyses on giant-cell tumors of bone.. Clin Orthop Relat Res 1989; (240) 250-260 PubMed 2917441
- 264 Haque AU, Moatasim A. Giant cell tumor of bone: a neoplasm or a reactive condition?. Int J Clin Exp Pathol 2008; 1 (6) 489-501 PubMed 18787633
- 265 Papanastassiou I, Ioannou M, Papagelopoulos PJ et al. P53 expression as a prognostic marker in giant cell tumor of bone: a pilot study.. Orthopedics 2010; 33 (5) 33 PubMed 20506947
- 266 Moskovszky L, Dezsö K, Athanasou N et al. Centrosome abnormalities in giant cell tumour of bone: possible association with chromosomal instability.. Mod Pathol 2010; 23 (3) 359-366 PubMed 20062006
- 267 Smith LT, Mayerson J, Nowak NJ et al. 20q11.1 amplification in giant-cell tumor of bone: array CGH, FISH, and association with outcome.. Genes Chromosomes Cancer 2006; 45 (10) 957-966 PubMed 16847944
- 268 Behjati S, Tarpey PS, Presneau N et al. Distinct H3F3A and H3F3B driver mutations define chondroblastoma and giant cell tumor of bone.. Nat Genet 2013; 45 (12) 1479-1482 PubMed 24162739
- 269 Rao UN, Goodman M, Chung WW, Swalski P, Pal R, Finkelstein S. Molecular analysis of primary and recurrent giant cell tumors of bone.. Cancer Genet Cytogenet 2005; 158 (2) 126-136 PubMed 15796959
- 270 Gorunova L, Vult von Steyern F, Storlazzi CT et al. Cytogenetic analysis of 101 giant cell tumors of bone: nonrandom patterns of telomeric associations and other structural aberrations.. Genes Chromosomes Cancer 2009; 48 (7) 583-602 PubMed 19396867
- 271 Forsyth RG, De Boeck G, Bekaert S et al. Telomere biology in giant cell tumour of bone.. J Pathol 2008; 214 (5) 555-563 PubMed 18278785
- 272 Schwartz HS, Dahir GA, Butler MG. Telomere reduction in giant cell tumor of bone and with aging.. Cancer Genet Cytogenet 1993; 71 (2) 132-138 PubMed 8281516
- 273 van der Heijden L, Dijkstra PDS, van de Sande MAJ et al. The clinical approach toward giant cell tumor of bone.. Oncologist 2014; 19 (5) 550-561 PubMed 24718514
- 274 Matsuo T, Hiyama E, Sugita T et al. Telomerase activity in giant cell tumors of bone.. Ann Surg Oncol 2007; 14 (10) 2896-2902 PubMed 17653593
- 275 Atkins GJ, Haynes DR, Graves SE et al. Expression of osteoclast differentiation signals by stromal elements of giant cell tumors.. J Bone Miner Res 2000; 15 (4) 640-649 PubMed 10780856
- 276 Skubitz KM, Cheng EY, Clohisy DR, Thompson RC, Skubitz AP. Gene expression in giant-cell tumors.. J Lab Clin Med 2004; 144 (4) 193-200 PubMed 15514587
- 277 Huang L, Xu J, Wood DJ, Zheng MH. Gene expression of osteoprotegerin ligand, osteoprotegerin, and receptor activator of NF-kappaB in giant cell tumor of bone: possible involvement in tumor cell-induced osteoclast-like cell formation.. Am J Pathol 2000; 156 (3) 761-767 PubMed 10702390
- 278 Roux S, Amazit L, Meduri G, Guiochon-Mantel A, Milgrom E, Mariette X. RANK (receptor activator of nuclear factor kappa B) and RANK ligand are expressed in giant cell tumors of bone.. Am J Clin Pathol 2002; 117 (2) 210-216 PubMed 11863217
- 279 Hemingway F, Taylor R, Knowles HJ, Athanasou NA. RANKL-independent human osteoclast formation with APRIL, BAFF, NGF, IGF I and IGF II.. Bone 2011; 48 (4) 938-944 PubMed 21193069
- 280 Liao TS, Yurgelun MB, Chang SS et al. Recruitment of osteoclast precursors by stromal cell derived factor-1 (SDF-1) in giant cell tumor of bone.. J Orthop Res 2005; 23 (1) 203-209 PubMed 15607894
- 281 Clézardin P. [The role of RANK/RANKL/osteoprotegerin (OPG) triad in cancer-induced bone diseases: physiopathology and clinical implications].. Bull Cancer 2011; 98 (7) 837-846 PubMed 21700551
- 282 Forsyth RG, De Boeck G, Baelde JJ et al. CD33 + CD14- phenotype is characteristic of multinuclear osteoclast-like cells in giant cell tumor of bone.. J Bone Miner Res 2009; 24 (1) 70-77 PubMed 18767926
- 283 Maggiani F, Forsyth R, Hogendoorn PC, Krenacs T, Athanasou NA. The immunophenotype of osteoclasts and macrophage polykaryons.. J Clin Pathol 2011; 64 (8) 701-705 PubMed 21561891
- 284 Lau YS, Sabokbar A, Gibbons CL, Giele H, Athanasou N. Phenotypic and molecular studies of giant-cell tumors of bone and soft tissue.. Hum Pathol 2005; 36 (9) 945-954 PubMed 16153456
- 285 Lindeman JH, Hanemaaijer R, Mulder A et al. Cathepsin K is the principal protease in giant cell tumor of bone.. Am J Pathol 2004; 165 (2) 593-600 PubMed 15277232
- 286 Mak IW, Seidlitz EP, Cowan RW et al. Evidence for the role of matrix metalloproteinase-13 in bone resorption by giant cell tumor of bone.. Hum Pathol 2010; 41 (9) 1320-1329 PubMed 20573369
- 287 Mak IW, Cowan RW, Popovic S, Colterjohn N, Singh G, Ghert M. Upregulation of MMP-13 via Runx2 in the stromal cell of giant cell tumor of bone.. Bone 2009; 45 (2) 377-386 PubMed 19422937
- 288 Ng PK, Tsui SK, Lau CP et al. CCAAT/enhancer binding protein beta is up-regulated in giant cell tumor of bone and regulates RANKL expression.. J Cell Biochem 2010; 110 (2) 438-446 PubMed 20225273
- 289 Balla P, Moskovszky L, Sapi Z et al. Epidermal growth factor receptor signalling contributes to osteoblastic stromal cell proliferation, osteoclastogenesis and disease progression in giant cell tumour of bone.. Histopathology 2011; 59 (3) 376-389 PubMed 22034878
- 290 Patibandla MR, Thotakura AK, Rao MN et al. Clival giant cell tumor—a rare case report and review of literature with respect to current line of management.. Asian J Neurosurg 2017; 12 (1) 78-81 PubMed 28413541
- 291 Dominkus M, Ruggieri P, Bertoni F et al. Histologically verified lung metastases in benign giant cell tumours—14 cases from a single institution.. Int Orthop 2006; 30 (6) 499-504 PubMed 16909252
- 292 Anract P, De Pinieux G, Cottias P, Pouillart P, Forest M, Tomeno B. Malignant giant-cell tumours of bone. Clinico-pathological types and prognosis: a review of 29 cases.. Int Orthop 1998; 22 (1) 19-26 PubMed 9549577
- 293 Bertoni F, Present D, Enneking WF. Giant-cell tumor of bone with pulmonary metastases.. J Bone Joint Surg Am 1985; 67 (6) 890-900 PubMed 4019539
- 294 Faisham WI, Zulmi W, Halim AS, Biswal BM, Mutum SS, Ezane AM. Aggressive giant cell tumour of bone.. Singapore Med J 2006; 47 (8) 679-683 PubMed 16865207
- 295 Maloney WJ, Vaughan LM, Jones HH, Ross J, Nagel DA. Benign metastasizing giant-cell tumor of bone: report of three cases and review of the literature.. Clin Orthop Relat Res 1989; (243) 208-215 PubMed 2656024
- 296 Stewart DJ, Belanger R, Benjamin RS. Prolonged disease-free survival following surgical debulking and high-dose cisplatin/doxorubicin in a patient with bulky metastases from giant cell tumor of bone refractory to “standard” chemotherapy.. Am J Clin Oncol 1995; 18 (2) 144-148 PubMed 7900706
- 297 Zorlu F, Selek U, Soylemezoglu F, Oge K. Malignant giant cell tumor of the skull base originating from clivus and sphenoid bone.. J Neurooncol 2006; 76 (2) 149-152 PubMed 16205965
- 298 Kaiser U, Neumann K, Havemann K. Generalised giant-cell tumour of bone: successful treatment of pulmonary metastases with interferon alpha, a case report.. J Cancer Res Clin Oncol 1993; 119 (5) 301-303 PubMed 8440744
- 299 Kaban LB, Troulis MJ, Wilkinson MS, Ebb D, Dodson TB. Adjuvant antiangiogenic therapy for giant cell tumors of the jaws.. J Oral Maxillofac Surg 2007; 65 (10) 2018-2024 PubMed 17884531
- 300 Chang SS, Suratwala SJ, Jung KM et al. Bisphosphonates may reduce recurrence in giant cell tumor by inducing apoptosis.. Clin Orthop Relat Res 2004; (426) 103-109 PubMed 15346059
- 301 Cheng YY, Huang L, Lee KM, Xu JK, Zheng MH, Kumta SM. Bisphosphonates induce apoptosis of stromal tumor cells in giant cell tumor of bone.. Calcif Tissue Int 2004; 75 (1) 71-77 PubMed 15037971
- 302 Martin-Broto J, Cleeland CS, Glare PA et al. Effects of denosumab on pain and analgesic use in giant cell tumor of bone: interim results from a phase II study.. Acta Oncol 2014; 53 (9) 1173-1179 PubMed 24834795
- 303 Tse LF, Wong KC, Kumta SM, Huang L, Chow TC, Griffith JF. Bisphosphonates reduce local recurrence in extremity giant cell tumor of bone: a case-control study.. Bone 2008; 42 (1) 68-73 PubMed 17962092
- 304 Balke M, Campanacci L, Gebert C et al. Bisphosphonate treatment of aggressive primary, recurrent and metastatic giant cell tumour of bone.. BMC Cancer 2010; 10: 462 PubMed 20799989
- 305 Yu X, Xu M, Xu S, Su Q. Clinical outcomes of giant cell tumor of bone treated with bone cement filling and internal fixation, and oral bisphosphonates.. Oncol Lett 2013; 5 (2) 447-451 PubMed 23420680
- 306 Arpornchayanon O, Leerapun T. Effectiveness of intravenous bisphosphonate in treatment of giant cell tumor: a case report and review of the literature.. J Med Assoc Thai 2008; 91 (10) 1609-1612 PubMed 18972907
- 307 George S, Merriam P, Maki RG et al. Multicenter phase II trial of sunitinib in the treatment of nongastrointestinal stromal tumor sarcomas.. J Clin Oncol 2009; 27 (19) 3154-3160 PubMed 19451429
- 308 Nouri H, Hedi Meherzi M, Ouertatani M et al. Calcitonin use in giant cell bone tumors.. Orthop Traumatol Surg Res 2011; 97 (5) 520-526 PubMed 21723215
- 309 De Chiara A, Apice G, Fazioli F, Silvestro P, Carone G, Manco A. Multicentric giant cell tumor with viral-like inclusions associated with Paget’s disease of bone: a case treated by steroid therapy.. Oncol Rep 1998; 5 (2) 317-320 PubMed 9468548
- 310 Ziambaras K, Totty WA, Teitelbaum SL, Dierkes M, Whyte MP. Extraskeletal osteoclastomas responsive to dexamethasone treatment in Paget bone disease.. J Clin Endocrinol Metab 1997; 82 (11) 3826-3834 PubMed 9360548
- 311 Chawla S, Henshaw R, Seeger L et al. Safety and efficacy of denosumab for adults and skeletally mature adolescents with giant cell tumour of bone: interim analysis of an open-label, parallel-group, phase 2 study.. Lancet Oncol 2013; 14 (9) 901-908 PubMed 23867211
- 312 Rutkowski P, Ferrari S, Grimer RJ et al. Surgical downstaging in an open-label phase II trial of denosumab in patients with giant cell tumor of bone.. Ann Surg Oncol 2015; 22 (9) 2860-2868 PubMed 26033180
- 313 Roitman PD, Jauk F, Farfalli GL, Albergo JI, Aponte-Tinao LA. Denosumab-treated giant cell tumor of bone: its histologic spectrum and potential diagnostic pitfalls.. Hum Pathol 2017; 63: 89-97 PubMed 28235628
- 314 Skubitz K, Thomas D, Chawla S, Staddon A. Response to treatment with denosumab in patients with giant cell tumor of bone (GCTB): FDG PET results from two phase 2 trials.. J Clin Oncol 2014; 32 (5) (Suppl. Suppl) 10505 NOT_FOUND
- 315 McKinney AM, Reichert P, Short J et al. Metachronous, multicentric giant cell tumor of the sphenoid bone with histologic, CT, MR imaging, and positron-emission tomography/CT correlation.. AJNR Am J Neuroradiol 2006; 27 (10) 2199-2201 PubMed 17110693
- 316 Aoki J, Watanabe H, Shinozaki T et al. FDG PET of primary benign and malignant bone tumors: standardized uptake value in 52 lesions.. Radiology 2001; 219 (3) 774-777 PubMed 11376267
- 317 Strauss LG, Dimitrakopoulou-Strauss A, Koczan D et al. 18F-FDG kinetics and gene expression in giant cell tumors.. J Nucl Med 2004; 45 (9) 1528-1535 PubMed 15347720
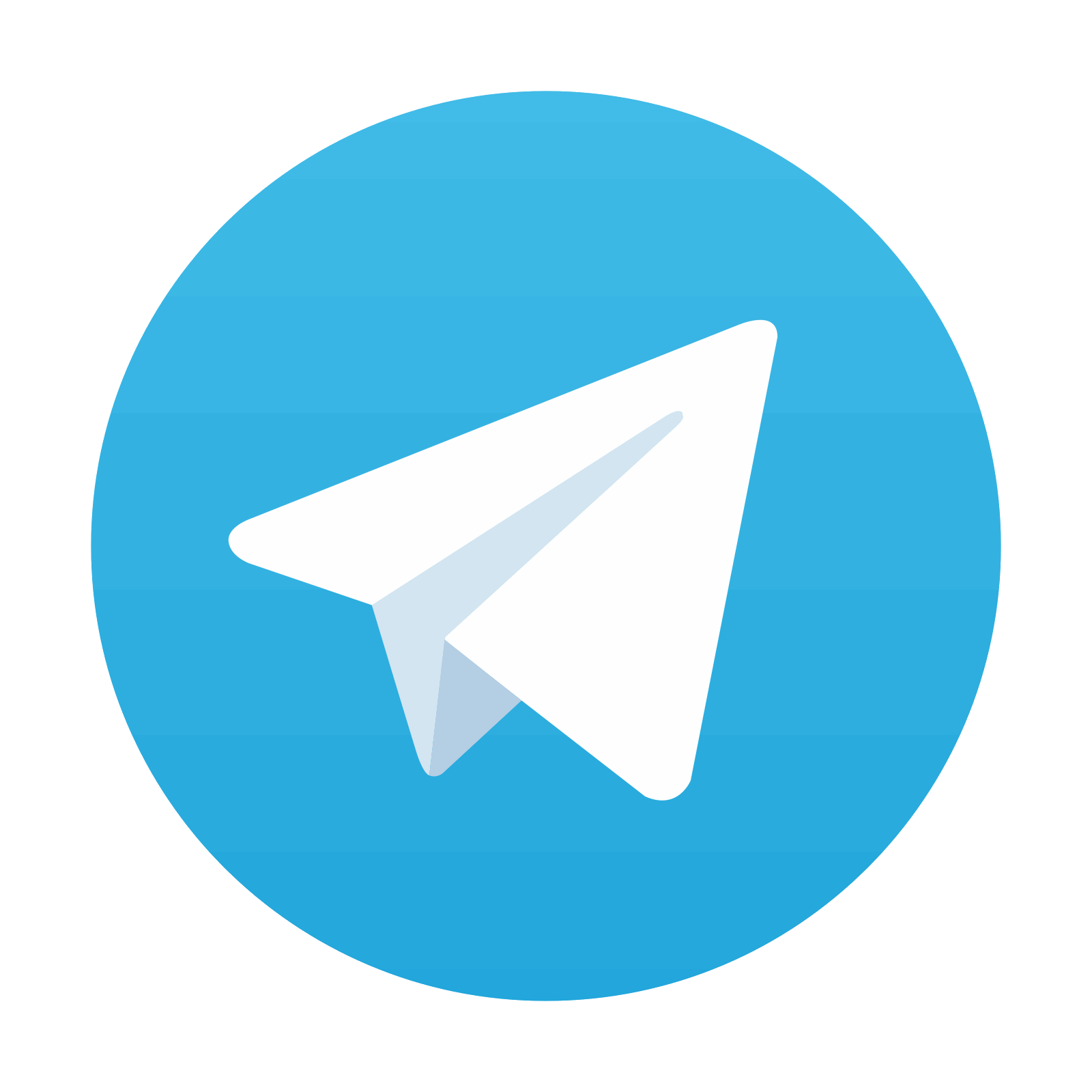
Stay updated, free articles. Join our Telegram channel

Full access? Get Clinical Tree
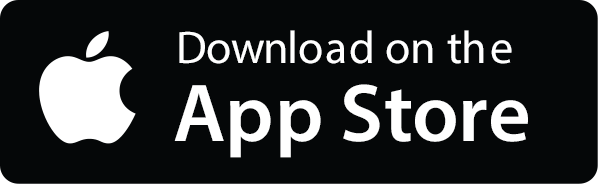
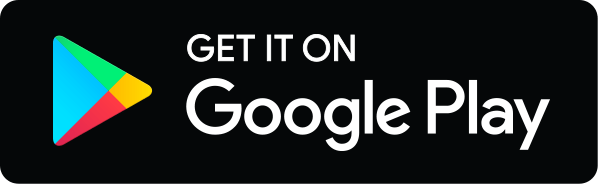