11 Coregistration and Newer Imaging Techniques
In presurgical evaluation of medically intractable epilepsy, knowledge of the precise location of the seizure focus is of paramount importance. Despite advances in imaging technologies, it is difficult to evaluate data from multiple structural and functional imaging modalities in an integrated fashion. Thus, clinicians may not be able to synthesize all of the available data in the most effective fashion. As such, coregistration of multiple modalities has proven to be an invaluable tool in the armementarium of the surgical team and has led to an increase in the number of patients offered surgery for epilepsy in the United States.1 In addition, as coregistration has improved neurosurgical efficacy, newer imaging techniques have improved presurgical lesion detection as well as demonstrating abnormalities in nonlesional epilepsy, such as hippocampal atrophy or sclerosis, cortical dysplasia, or small vascular lesions or tumors.2
Coregistration
Direct data fusion technique superimposes multiple images and presents the surgeon with a coarse approximation of the location of functional regions. This may be adequate when the surgical target is single, discrete, and in noneloquent territory. However, if the target is multifocal, indistinct, or in eloquent cortex, an understanding of the correlation between structure and function is very helpful.
Coregistration is the process of volumetrically fusing processed functional images onto a structural image by using imaging data shared by all images3,4 and as such offers a significant advantage over direct image overlay. Image-to-image coregistration commonly relies on the identification of mutual points in both images known as tie-points. As such, software packages designed to coregister images, such as Statistical Parametric Mapping (SPM, The Math-works, Inc. Natick, MA) Free Surfer (A.A. Martinos Center for Biomedical Imaging, Boston, MA), 3D Slicer (Boston, MA), AFNI (Bethesda, MD), Computerized anatomical reconstruction and editing toolkit (Caret, St Louis, MS), commonly use an automated, area-based technique for identifying image tie-points. Coregistration software executes either a linear or nonlinear transformation; linear transformation is technically easier and faster than nonlinear transformation but yields significantly lower-quality image coregistration.5 Initially, functional data derived from positron emission tomography (PET) single photon emission computed tomography (SPECT) would be volumetrically coregistered with a standardized stereotaxic brain atlas such as that of Talairach and Tournoux,6,7 but variations in gross morphology and microstructure of the human brain8 make an atlas-based methodology unreliable in presurgical planning for individual patients.9 As a result, clinical coregistration generally implies that all data involved in volumetric fusion are derived entirely from the individual patient.
One of the first efforts to address the challenge of image data fusion for surgical planning was Mountz and colleagues’ description of the fusion of SPECT and computed tomography (CT) images of patients’ brains as a method of providing an accurate and noninvasive method for correlating function (in the form of blood flow) and neuroanatomy.10 The authors demonstrate lesion/pathology in clinical examples of tumor, developmental abnormality (autism), cerebrovascular disease, and, notably, in epilepsy via injection of tracer during ictal phase of seizure.10
The arrival of magnetic resonance imaging (MRI) heralded clinicians’ ability to define neuroanatomical targets with high precision. As such, MRI replaced CT for surgical planning, and MRI–PET coregistration eclipsed PET–CT coregistration as standard of care in presurgical planning (see Fig. 11.1 ). Evidence of this evolution was borne out by Viñas and colleagues’ study of MRI–PET coregistration in presurgical planning for epilepsy surgery in eloquent cortex.11 Twelve patients underwent preoperative MRI–PET coregistration with motor, visual, and language mapping and subsequently underwent an awake craniotomy with MRI-assisted image guidance and intraoperative cortical stimulation or visual evoked potentials. The researchers found that PET was reliable in identifying most (but not all) motor, visual, and language cortex, leading to their conclusion that MRI–PET coregistration is a useful tool for identification of eloquent cortex in the setting of neurosurgical preplanning.11 Although the authors concluded that intraoperative cortical stimulation and visual evoked potential remained the gold standard for cortical mapping,11 further refinements of SPECT, PET, and MRI have given this type of coregistration increased precision and reliability in presurgical planning for epilepsy (see Fig. 11.2 ).12,13 A particularly effective refinement was that of “fuzzy modeling” in which Boussion and colleagues were able to demonstrate results in accordance with the “gold standard” investigation (deep electrodes or postsurgical outcome) in 11 of 12 patients in a clinical study when fuzzy logic was applied to modeling PET and MRI coregistration.14 With further refinements, PET–MRI coregistration has proven to be clinically valuable in patients outside the purview of medically refractory epilepsy: by differentiating actual hypoperfusion from artifactual hypoperfusion resulting from partial volume effects and also to improve the accuracy of asymmetry indexes in interictal patients, the use broadened to patients with partial epilepsy.12 Another challenge during the infancy of coregistration was speed—significant offline processing and manual registration work was necessary to achieve acceptably accurate coregistered PET–MRI images.15 By combining a multiresolution approach with an automatic segmentation of input image volumes into areas of interest and background, Cízek and colleagues demonstrated that with suitable preprocessing, the time to coregister PET and MRI images with robust accuracy could be reduced by ten-fold.16

The next step in coregistration was the combination of MRI, CT, and PET coregistration to assist in image-guided placement of subdural electrodes and then using the information gathered by the subdural electrodes (combined with the precise location of the electrodes by CT) to aid in surgical resection of the suspected epileptogenic focus in patients undergoing image-guided surgical treatment of epilepsy.17–20 The advent of readily available digital cameras has ushered in an even higher level of certainty in epilepsy surgery; because coregistration of digital photographs of the brain cortex with the results of three-dimensional MRI datasets is now possible,21,22 it allows for identification of anatomical details underlying the subdural grid electrodes and enhances the intraoperative certainty and precision of the neurosurgeon. In addition, surgical navigation systems found in most modern neurosurgical operating theaters allow for coregistration of preoperative datasets to intraoperative head position of the patient.23,24
The clinical effectiveness of multimodal coregistration has been borne out in several studies. In 2004, Murphy and colleagues evaluated the outcomes of 22 patients selected to undergo multimodal coregistration [PET–MRI, SPECT–MRI, or fluid attenuation inversion recovery (FLAIR)–MRI] for presurgical planning because of no lesion visible on conventional MRI sequences, multiple lesions, or one very large lesion that could not be completely resected without the risk of significant postoperative morbidity.1 Another group with lesions within eloquent cortex was included in the study and underwent further coregistration with subdural electrocorticography grids.1,17 After an average of 27 months postsurgical follow-up, the authors found that 77% of the patients had excellent outcomes for their seizures, and 86% had favorable outcomes. One patient suffered a permanent major deficit, whereas three other patients suffered permanent minor deficits. In light of the fact that all of these patients would have been poor surgical candidates without presurgical multimodal coregistration, this study provides a strong argument that this approach may allow the reevaluation of patients previously denied surgery because of the selection criteria outlined by the paper’s authors.

Murphy et al’s findings were confirmed in 2007 when Doelken et al studied 49 temporal lobe epilepsy (TLE) patients with coregistration of MRI, MRI spectroscopy, and SPECT and compared the imaging results with that of traditional non-invasive EEG-video monitoring and evaluated lateralization of affected hemisphere with regard to bilateral affection and postoperative outcome.25 The authors found that EEG and MRI had a high concordance in establishing unilaterality or bilaterality for TLE and epileptogenic focus and, under the circumstances of ambiguous laterality on MRI or EEG, SPECT and MR spectroscopy, aided in identifying an epileptogenic focus, and, if so, if the lesion was unilateral or bilateral.25 As a result, the study demonstrated that multimodal imaging for epilepsy helps in identifying bilateral involvement, which is important to identify the patients who will not benefit from epilepsy surgery.
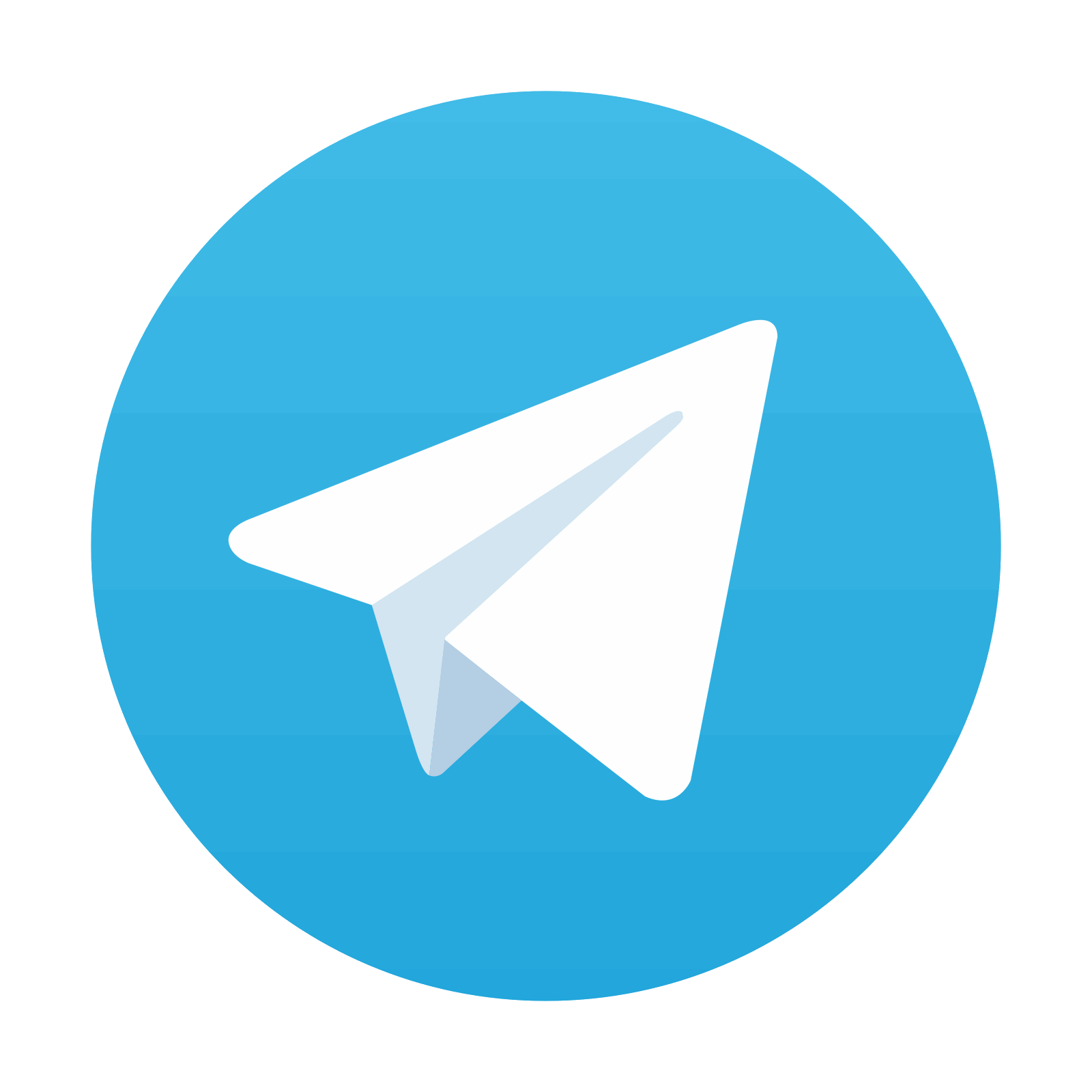
Stay updated, free articles. Join our Telegram channel

Full access? Get Clinical Tree
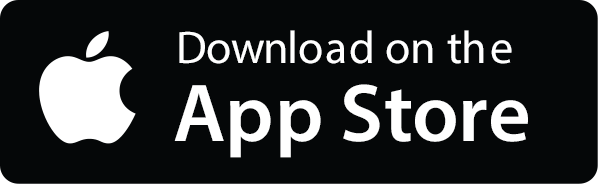
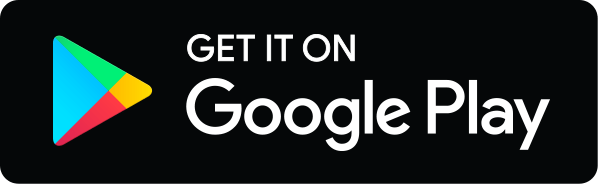