CHAPTER 11 Michelle Mattingly1, Katie Osborn2 and Leon Prockop1 1 University of South Florida, Tampa, FL, USA 2 Geisel School of Medicine at Dartmouth, Hanover, NH, USA Toxic and metabolic forms of dementia (TMD) are critical to identify as they are often treatable, if not curable, unlike most other dementias discussed in this book. Cognitive impairment might be a direct consequence of medical illness or metabolic perturbations. Although technically not dementia, delirium should always be considered as an etiology of cognitive impairment. First, we discuss toxic causes of dementia, followed by a review of major metabolic etiologies of cognitive impairment. Exposure to toxic substances, either acutely or chronically, can lead to dementia. Table 11.1 includes a list of several toxic agents that might cause dementia [1]. This section of the chapter will focus on three agents that are among the most common causes of toxic cognitive dysfunction—carbon monoxide, ethanol, and lead. Table 11.1 Toxic causes of dementia (differential diagnosis). Carbon monoxide (CO) is a leading cause of death from poisoning in the United States and is responsible for 40 000 emergency department visits and 5000 to 6000 deaths per year [2]. CO is a tasteless, odorless, nonirritating but highly toxic gas, which makes it difficult to detect. Therefore, the true incidence of CO poisoning is unknown. CO is a by-product of the incomplete combustion of hydrocarbons. Common sources of CO exposure include intentional suicides as well as unintentional exposures from home heating, automobile exhaust, and smoke inhalation [2]. CO poisoning may occur as a result of a building fire or from fuel-powered generators and heaters, particularly in poorly ventilated spaces. The latter commonly occurs during winter storms, earthquakes, and hurricanes following a power outage. VB, a 39-year-old woman, and SB, her 8-year-old daughter, had lived in an apartment for several years without incident. In the fall of 1998, both suffered recurrent illnesses characterized by lethargy, headache, and occasional vomiting diagnosed as “flu” when medical assistance was sought. On several occasions, they stayed with friends while recuperating. Symptoms cleared quickly, only to recur on resuming residence in the apartment. VB, an educator, noted new learning dysfunction. SB’s previously high grades declined. Suspecting an environmental toxin, VB summoned local health officials who found 106 ppm CO in a random apartment air sample. Although they vacated the apartment, headaches, malaise, and intellectual problems persisted such that VB discontinued efforts toward a PhD degree. SB experienced low school grades and had a B rather than a prior A average. On neurological examination, both were within normal limits except for cerebellar dysfunction characterized by loss of check with rebound in both with mild truncal ataxia in VB. Neuropsychological tests in VB demonstrated moderate to severe cognitive deficits, especially those involving executive functions. In SB, neuropsychological tests yielded scores from borderline to severe impairment in nonverbal sequences, complex copying, visuospatial judgment, and organization. Compared to premorbid estimated levels of functioning, both showed deficits involving higher-order cognitive functioning, reading comprehension, and qualitative conceptualization. MRI and MRS in both were normal. The brain and the heart are most susceptible to CO toxicity due to their high metabolic rates. Clinical symptoms of CO poisoning tend to be nonspecific and can mimic a variety of common disorders. Symptoms range in severity from mild, flu like (simulating a viral infection) to more severe leading to coma and death. The general symptoms of CO poisoning include headache, malaise, difficulty concentrating, dizziness, nausea, fatigue, and weakness. When not fatal, a wide spectrum of CO-induced encephalopathy ranges from reversible dysfunction to severe irreversible dementia. Table 11.2 lists some of the clinical signs and symptoms associated with CO poisoning and correlated carboxyhemoglobin (COHb) levels [2, 4]. Table 11.2 Clinical signs and symptoms associated with CO poisoning and correlated COHb levels. * At moderate to severe levels of COHb poisoning the correlation between blood levels and symptomatology is poor. Neurologic symptoms include dementia, cerebellar dysfunction, and parkinsonism [5]. A delayed neuropsychiatric syndrome may follow acute exposure by 3–240 days, with cognitive and personality changes and psychotic behavior [5, 6]. This syndrome is characterized by a relapse of symptoms associated with CO poisoning, occurring after a transient period of improvement. Whereas the physical and cognitive symptoms associated with severe acute exposure are obvious, the cognitive sequelae of low-level exposure are more easily misdiagnosed or overlooked [7]. Affective and personality changes following CO exposure are occasionally more prominent and/or life changing than cognitive impairment. Depression, anxiety, obsessive–compulsive behaviors, delusions and hallucinations, violent outbursts, fear, elated mood, apathy, lack of motivation, and irritability are commonly reported symptoms [8, 9]. Depression and anxiety likely result from a combination of factors that include neural injury and psychological reactions to the physical and cognitive sequelae that occur following CO poisoning. Although not necessarily specific to CO intoxication, common neurological exam findings may include abnormalities on the Romberg test, impaired heel–toe gait, dysdiadochokinesis, and poor performance on finger nose testing and serial sevens. Impaired fine movements, nystagmus, ataxia, compromised mental status examination, impaired reflexes, and intention tremor may also present. In severe cases, a tonic disorder or decerebrate rigidity associated with increased deep tendon reflexes and extensor plantar responses may be observed. Cortical blindness may be transitory and characterized by visual loss but intact pupillary reactions to light. Visual field defects, paracentral scotomas, decreased light sensitivity, and decreased dark adaption have been reported [10]. Flame-shaped superficial retinal hemorrhages have been reported in long-term, subacute CO poisoning but are nonspecific [11]. Papilledema can be evident on funduscopic examination. The classic cherry-red discoloration of the skin and cyanosis are rare. Cognitive impairment occurs in 15–49% of individuals exposed to CO [12]. No consistent pattern of cognitive deficits following CO poisoning has been observed, but impaired memory, attention, information processing speed, and executive function are common sequelae. Persistent cognitive dysfunction lasting more than one month appears to occur in 25–50% of individuals with associated loss of consciousness or COHb levels greater than 25% [13–16]. Even those individuals with less severe and lower level CO poisoning might develop cognitive impairments [17]. In general, neuroimaging demonstrates evidence of morphologic damage that correlates to that reported in postmortem pathological studies. A distinct constellation of brain and MRI abnormalities includes globus pallidus lesions, white matter changes, and diffuse low-density lesions throughout the brain. Abnormalities in the globus pallidus is common on both CT and MRI studies. Cerebral edema might occur early and sometimes predicts poor outcome (e.g., death or severe permanent damage). Globus pallidus changes do not always predict outcome. Diffuse deep white matter MRI abnormalities correlate better with clinical status and prognosis; these changes are probably related to hypoxia/ischemia associated with CO toxicity [18–20]. The brain MRI might appear normal in some cases of dementia caused by CO exposure; in such circumstances, brain MRS abnormalities are often present. As MRS examines brain metabolites, the abnormal MRS often reflects decreased n-acetylaspartase in the basal ganglia bilaterally [3]. Ambient air levels of CO should be obtained as soon as possible after CO exposure. The Centers for Disease Control and Prevention (CDC) [21] guidelines indicate the key to confirming the diagnosis in measuring the patient’s COHb level, which can be tested either in whole blood or exhaled air. COHb level should be obtained immediately as the half-life of COHb is 4–5 h. Normal levels are <2% nonsmokers and 5–13% for smokers. An elevated COHb level of ≥2% for nonsmokers or >12% for smokers strongly supports a diagnosis of CO poisoning. Pulse oximetry is unreliable for the diagnosis of CO intoxication because it is unable to distinguish oxyhemoglobin from COHb. A thorough and accurate assessment of clinical symptoms and CO exposure history is critical to determine the type and intensity of treatment as COHb levels do not correlate well with severity of illness, outcomes, or response to treatment. CO toxicity is caused by impaired oxygen delivery and use, leading to cellular hypoxia. Inhalation results in CO binding rapidly to Hb, leading to the formation of COHb. The oxygen-carrying capacity of the blood decreases, causing tissue hypoxia. The affinity of Hb for CO is 210 times its affinity for oxygen, and CO easily displaces oxygen from Hb. COHb also impairs the release of oxygen from Hb by increasing oxygen binding to Hb, resulting in decreased oxygen delivery to cells. CO also might directly cause mitochondrial damage [22]. The neuropathology of CO toxicity has been well described in postmortem studies [23] and in acute cases, includes petechial hemorrhages of the white matter, in particular involving the corpus callosum. In cases surviving more than 48 h, there is multifocal necrosis involving globus pallidus, hippocampus, pars reticularis of the substantia nigra, laminar necrosis of the cortex, and loss of Purkinje cells in the cerebellum, along with white matter lesions. The typical pallidum lesions are well defined—bilateral globus pallidus macroscopic infarctions, usually asymmetrical, extending anteriorly, superiorly, or into the internal capsule. Occasionally, only a small linear focus of necrosis is found at the junction of the internal capsule and the internal nucleus of the globus pallidus. Less commonly, hemorrhages in the hippocampus are seen. CO intoxication usually spares the hypothalamus, walls of the third ventricle, thalamus, striatum, and brainstem. Myelin damage is constant and ranges from discrete, perivascular foci in the corpus callosum, the internal–external capsule and the optic tracts usually seen in comatose patients who died within 1 week, to extensive periventricular demyelination and axonal destruction observed in comatose patients with longer survival, sometimes leading to formation of plaques of demyelination [5]. Tissue hypoxia is the major outcome of CO intoxication; therefore, based on chemical and pathophysiological data, oxygen is the “natural antidote” [24]. The CDC [21] recommends the following guidelines for the management of CO poisoning: Although some nonrandomized studies indicate that HBO reverses both the acute and delayed effects of CO poisoning, other data are conflicting. Therefore, it is not clear whether HBO influences the rates of either late sequelae or morbidity. It is generally agreed that HBO hastens resolution of symptoms and is usually indicated [25–29]. Ethanol is a specific type of alcohol, and the only type that can be consumed. Within this section ethanol and alcohol will both be used to refer to the effects of Ethanol, given that alcohol is the more frequently used term. Ethanol intoxication is the consequence of an accumulation of it and its metabolites in the bloodstream that occurs faster than can be metabolized by the liver. In the United States, roughly 10% of adults meet diagnostic criteria for an alcohol abuse or dependence disorder. Of those, approximately 50% who become dependent exhibit neurocognitive impairments when assessed 3–4 weeks following abstinence [30]. A variety of dementia symptoms and neurological dysfunction can occur after ethanol ingestion [31] (Table 11.3). Table 11.3 Major dementia issues in alcoholics (sometimes nutritional). Ethanol acts as a central nervous system depressant (Table 11.4). Acute overconsumption can lead to tremors, seizures, hallucinations, ataxia, neuropathy, stroke, stupor, respiratory depression, coma, and death. Visual findings may include suppression of rapid eye movement during sleep, slow saccadic eye movements, and interrupted jerky pursuit movements. Gait and balance disturbances are also common and may persist after detoxification [32, 33]. Chronic overconsumption can cause liver damage, as well as severe withdrawal symptoms, including seizures, hallucinations, and autonomic dysfunction (i.e., delirium tremens) [34]. Table 11.4 Metabolic causes of dementia (differential diagnosis). Ethanol affects cognition both directly and indirectly. Ethanol is a direct neurotoxin and when sufficiently abused may lead to dementia. Ethanol, or possibly the components of alcohol, such as certain compounds in red wine including resveratrol, however, appear to have neuroprotective properties and reduce the risk of dementia, including Alzheimer’s type, when ingested in low-to-moderate amounts [35]. Further, both abstaining from and chronically abusing alcohol are associated with elevated risks of dementia. The relationship between drinking behavior and risk for dementia is described as a J-curve, meaning those who abstain from alcohol completely appear to develop dementia at higher rates than those who regularly drink moderate amounts of alcohol (e.g., up to three glasses of alcohol per day), whereas individuals who chronically abuse alcohol are also at high risk of developing dementia [36]. There is evidence of the beneficial effects of light–moderate, nonbinge consumption of alcohol, as well as experimental demonstration that moderate alcohol exposure can initiate cytoprotective mechanisms [37]. Intoxication, withdrawal, brain trauma, central nervous system infection, hypoglycemia, alterations in bodily systems (e.g., immune, hormonal), cerebellar degeneration, polyneuropathy, fetal alcohol syndrome, hepatic failure, and Marchiafava–Bignami disease are indirect effects of alcohol abuse, as are pellagra and Wernicke–Korsakoff disorder, resulting from nutritional deficiencies [31]. The precise etiology of neuropsychological impairment in alcohol abusers is controversial. Acute impairment tends to correlate reasonably well with BAC. Acute neuropsychological dysfunction is evident when BAC exceeds 50 mg/dL (see Table 11.5). The chronic effects of alcohol on the brain have been well studied. Alcohol dementia is characterized by gradually progressive, multidomain cognitive dysfunction with prominent neuropsychological deficits on tasks measuring visuospatial skills, abstraction, learning, and memory [30]. Language skills and global intellectual functioning remain relatively intact with chronic alcohol consumption. In contrast, Wernicke–Korsakoff syndrome, a brain disorder produced by thiamine deficiency, is characterized primarily by acute to subacute onset of severely impaired short-term recall, confabulation (fabrication), oculomotor abnormalities, and gait ataxia, with relatively preserved long-term memory and global intellectual functions. There can also be comorbidity of alcohol dementia and Wernicke–Korsakoff resulting in global cognitive impairment [30]. Cognitive impairment due to alcohol abuse likely reflects the combination of acute and chronic brain damage including alcohol intoxication, vitamin deficiency, metabolic disorders, and cerebrovascular diseases. The increased likelihood of psychopathology and head trauma/brain injury in individuals who abuse alcohol further complicate the diagnosis and prognosis. Ethanol-related dementia is believed to represent approximately 10% of all dementia cases [38]. Chronic alcohol consumption is associated with cerebral atrophy of both grey and white matter with preferential involvement of the frontal lobes [7]. Reports indicate that alcoholic patients with dementia have reduced numbers of neurons in the superior frontal cortex [39]. Cerebellar atrophy, particularly of the vermis, is also common. These pathological changes are confirmed by computed tomography (CT) or MRI brain imaging [40]. Research has indicated that drinking cessation might lead to a reduction in cerebral atrophy [41, 42], accompanied closely by improved cognition; amnesia associated with Wernicke–Korsakoff’s is generally permanent, however [31]. Symptoms associated with acute ethanol or alcohol toxicity tend to progress proportionally to the amount ingested, which is measured directly via BAC level. Although there is some interindividual variability, O’Connor [34] provides the guidelines for expected effects by BAC in Table 11.5. Table 11.5 Central nervous system (CNS) effects after ethanol ingestion as a function of blood-alcohol content (BAC). Diagnosis is typically clinical, but in cases of acute intoxication, BAC should also be measured, and blood glucose level should be checked to rule out hypoglycemia. In chronic alcohol abuse, complete blood count (CBC), magnesium (Mg), liver function tests, and prothrombin time/partial prothrombin time (PT/PTT) should be ordered. In cases of alcohol withdrawal, the clinician should rule out of other central nervous system injuries or infections [34]. Several blood markers have been used to assess the effects of chronic alcoholism in clinical practice. These include elevated serum gamma-glutamyltransferase (GGT) and aminotransferase (ALT, AST) levels and mean corpuscular volume (MCV). These have poor specificity and limited sensitivity to determine postmortem evidence of chronic alcohol use, however [43]. New markers include carbohydrate-deficient transferrin (CDT), fatty acid ethyl esters (FAEES), fatty acid methyl esters (FAMEs), ethyl glucuronide (EtG), phosphatidylethanol (PEth), 5-hydroxytryptophol to 5-hydroxyindole-3-acetic acid (5-HTOL/5-HIAA) ratio, sialic acid, beta-hexosaminidase, blood acetate, acetaldehyde adducts, and dolichol [44]. Ethanol neurotoxicity likely has several mechanisms, including glutamate excitotoxicity and oxidative stress, which is sometimes exacerbated by thiamine deficiency [45–48]. The neuropathological correlates of alcoholism have been well documented via autopsy studies [45, 49–53]. In general, findings support the tendency for individuals with alcohol dependence to exhibit reductions in brain weights and increased pericerebral spaces consistent with hydrocephalus ex vacuo, as well as pronounced neuronal loss in the mammillary bodies, thalamus, basal forebrain, dorsal and medial raphe, and cerebellar vermis. Interested readers should refer to Table 11.6 for a brief overview of the medical management of ethanol intoxication and withdrawal [31]. Table 11.6 Medical management strategies for ethanol intoxication and withdrawal. There are a variety of inpatient and outpatient therapeutic alcohol treatment programs available in most countries, including the United States. Relapse rates are high, however, and psychotherapeutic treatment should follow medical detox. Lead is a natural, abundantly occurring metal used frequently in manufacturing and industry, and it has been extensively researched as a neurotoxicant [54]. Lead neurotoxicity can be due to acute or chronic exposure. Acute lead encephalopathy in children, attributed to pica or ingestion of flaking lead-containing paint, was first reported in 1904 [1]. Lead poisoning, however, is among the oldest occupational illnesses and remains a common cause of metal poisoning today. Acute lead exposure may involve the rapid onset of nausea, headaches, cognitive changes, and emotional dysregulation. Medical symptoms can include hypertension, abdominal pain, constipation, and joint/muscle pains. Chronic exposure, which is more typical of industrial workplace environments, reflects more prominent neurodegeneration and psychiatric manifestations. Lead-exposed workers frequently complain of fatigue, headaches, restlessness, irritability, and poor emotional control [55–57]. There is some evidence that chronic lead exposure may impair postural balance and speed of peripheral nerve function, as well as contributing to forearm extensor weakness. Psychosis with hallucinations, restlessness, and nightmares are typical psychological symptoms produced by organic lead exposure [55, 58]. Delirium, convulsion, and coma might even occur in high concentration exposure. In sum, the deleterious effects of lead on the brain range from mild cognitive decline to encephalopathy, parkinsonism, and dementia [59]. Although acute toxicity is rare, chronic toxicity causes both central and peripheral effects, the former more common in children and the latter in adults. Adults with chronic lead exposure and blood lead levels from 25 to 60 µg/dL often experience irritability, headache, and depressed mood, with signs of impaired visual–motor dexterity and reaction times. Even more overt effects, for example, weakness and atrophy of peripheral muscles with wrist drop, occur with long-term blood levels of 60 µg/dL or more. Peripheral neuropathy is usually accompanied by blood lead levels greater than 70 µg/dL. Chronic exposure over months or years adversely affects calcium-dependent enzyme systems, ATPases, and mitochondrial oxidative phosphorylation and cell growth; interferes with heme synthesis, membrane integrity, and steroid metabolism; and causes motor axon degeneration and demyelination [60]. Additional potential consequences include anemia, reduced sperm count, renal failure, chronic encephalopathy, delayed motor and sensory nerve conduction, hearing loss, and gout. Lead exposure is considered a significant risk factor for accelerated cognitive decline [61, 62]. A consistent pattern of neurocognitive deficits associated with lead exposure has not been identified, although visuospatial and visual memory impairments are frequently reported as the most prominent declines [63, 64]. A cross-sectional study of workers in a leaded glass plant found deficits in psychomotor speed, motor strength, and verbal memory [56]. Other studies, however, have found no or few abnormal cognitive deficits in exposed persons, which may be due to moderately low exposure or current blood levels. Leaded fuels contain multiple neurotoxic substances; therefore, the neuropsychological deficits likely result from a combination of triethyl lead, solvents, and possible hypoxia [65]. Chronic exposure to lead tends to be more harmful to cognition than acute exposure as bone lead levels predicted poorer cognitive performance, whereas blood lead level did not [66]. Anxiety, depression, phobia, and antisocial behavior are linked to lead exposure [67].
Toxic and metabolic dementias
Introduction
Toxic dementias
Agent
Occupational or Other exposure
Syndrome
Acute
Chronic
METALS
Arsenic
Pesticides, pigments, paint, electroplating, seafood, smelter, semiconductors
Encephalopathy with dementia
Neuropathy
Bismuth
Over ingestion of bismuth salicylates (e.g., PeptoBismol®)
Encephalopathy, ataxia, myoclonus
Possible irreversibility, death
Lead
Solder, lead shot, illicit whiskey, insecticides, auto body shop, storage battery manufacture, smelter, paint, water pipes, gasoline sniffing
Encephalopathy with dementia
Encephalopathy, neuropathy, motor neuron disease- like syndrome
Manganese
Iron industry, welding, mining, smelter, fireworks, fertilizer, dry cell batteries
Encephalopathy
Parkinsonism
Mercury
Thermometers, other gauges, dental office (amalgams), felt hat manufacture, electroplating, photography
Headache, tremor with dementia
Neuropathy, encephalopathy with dementia, tremor
Tin
Canning industry, solder, electronics, plastics, fungicides
Delirium
Encephalomyelopathy with dementia
RECREATIONAL ABUSE
Nitrous oxide
Dental offices
Encephalopathy with dementia
B12-deficient myelopathy
ENVIRONMENTAL/FOOD
Ciguatera (from fish)
Sensory neuropathy with temperature inversion
Shellfish
Acute neuropathy
Carbon monoxide
Accidental or deliberate exposure in motor vehicles, faulty gasoline-fueled heaters
Anoxic encephalopathy with dementia
Encephalopathy, delayed neuropsychiatric syndrome, dementia
Methyl alcohol
Contaminated illicit whiskey
Retinal blindness
SOLVENTS
Carbon disulfide
Rayon manufacture, preservatives, textiles, rubber cement, varnish, electroplating
Encephalopathy with dementia
Neuropathy, Parkinsonism
Trichlorethylene
Paints, degreasers, spot removers, decaffeination, dry cleaning, rubber solvents
Narcosis
Encephalopathy, trigeminal neuropathy, dementia
Hexacarbons
Paints, paint removers, varnish, degreasers, rapid-drying ink, glues, cleaning agents, glues for making shoes in poorly vented cottage industry, glue sniffing, MNBK in plastics
Narcosis
Neuropathy, encephalopathy, ataxia, dementia
INSECTICIDES
Organophosphates, carbamates
Manufacture, application
Cholinergic syndrome
Ataxia, neuropathy, myelopathy
Carbon monoxide
Case presentation (reprinted with permission from Prockop [3])
Chronic CO exposure
Clinical symptoms/features
Severity
COHb level
Signs and symptoms
Mild
<15–20%
Headache, nausea, vomiting, dizziness, blurred vision
Moderate
21–40*
Confusion, syncope, chest pain, dyspnea, weakness, tachycardia, rhabdomyolysis
Severe
41–59*
Palpitations, dysrhythmias, hypotension, myocardial ischemia, cardiac arrest, respiratory arrest, pulmonary edema, seizures, coma
Fatal
60 + %
Death
Neurologic exam
Neuropsychological findings
Neuroimaging
Laboratory findings
Pathophysiology and pathology
Treatment
Ethanol
Disorder
Clinical features
Probable deficiency
Wernicke syndrome
Dementia with lethargy, inattentiveness, apathy, and amnesia
Thiamine
Ophthalmoparesis
Gait ataxia
Korsakoff syndrome
Dementia, mainly amnesia, with or without confabulation
Thiamine
Cerebellar degeneration
Gait ataxia; limb coordination relatively preserved
Probably thiamine plus other vitamins plus ethanol neurotoxicity
Polyneuropathy
Distal limb sensory loss and weakness; less often autonomic dysfunction
Probably thiamine plus other vitamins plus ethanol neurotoxicity
Amblyopia
Optic atrophy, decreased visual acuity, central scotomas; total blindness rare.
Probably thiamine plus other vitamins plus ethanol neurotoxicity
Clinical and neurologic symptoms/features
Disorder
Clinical features
Endocrine
1. Thyroid
(a) Hypo
Encephalopathy, dementia
(b) Hyper
Dementia
2. Pituitary
(a) Hypo
Encephalopathy, dementia
(b) Hyper
Visual disturbances/increased LCP
3. Adrenal
(a) Hypo
Lethargy, fatigue, dementia
(b) Hyper
Seizures, dementia
Hepatic
Encephalopathy, dementia
Cardiac/pulmonary/hypoxia
Encephalopathy, dementia
Vitamin deficiency
Encephalopathy, dementia, peripheral neuropathy
Electrolyte disturbances
Encephalopathy, dementia
Glucose derangement
Encephalopathy, seizures, dementia, peripheral neuropathy
Neuropsychological findings
Neuroimaging
Biomarkers/laboratory findings
mg/dL
Typical presentation
20–50
Tranquility, mild sedation, mild fine motor coordination deficits
50–100
Judgment impairment, further coordination deficits
100–150
Unsteady gait, nystagmus, slurring of speech, behavioral inhibition, impaired memory
150–300
Delirium and lethargy
300–400
Unconsciousness in the alcohol-naïve; chronic consumers may still appear unaffected in context of extreme tolerance
≥400
Potentially fatal
Pathophysiology and pathology
Treatment
Medication
Mechanism of action
Effects
Side effects/risks
Disulfiram
Interferes with metabolism of acetaldehyde (intermediary product in the oxidation of alcohol)
Alcohol consumption within 12 h of taking disulfiram leads to facial flushing, suffusion of conjunctivae, throbbing headache, tachycardia, hyperpnea, sweating, vomiting, and potentially fainting and collapse, all of which typically lasts up to 3 h. These intense discomforts discourage alcohol consumption
Adherence rates are poor; contraindicated for patients who are pregnant
Naltrexone
Opioid antagonist
Decreases relapse rate and number of drinking days during relapse
Adherence rates are modest; contraindicated in patients with acute hepatitis or liver failure
Acamprosate
Synthetic analog of y-aminobutyric acid
May decrease relapse rate and number of drinking days during relapse
Nalmefene
Opioid antagonist
May decrease relapse rate and number of drinking days during relapse
Still currently under study
Topiramate
Anticonvulsant/antiepileptic drug (AED)
May decrease relapse rate and number of drinking days during relapse
Still currently under study; some problems with memory and general neurocognitive functioning have been reported
Magnesium supplementation
Metal ion; necessary cofactor in thiamin-dependent metabolism
Correction of electrolyte abnormalities and thiamin deficiency
IV/IM administration should continue for at least 3–5 days; advanced disease necessitates hospitalization
Thiamine
Vitamin B1
Prevent Wernicke–Korsakoff syndrome and symptoms of severe withdrawals or delirium tremens
IV delivered; should remain in ICU over course of symptoms
Diazepam, lorazepam, chlordiazepoxide, carbamazepine oxazepam, etc.
Benzodiazepines
Counteracts and/or prevents withdrawal symptoms, especially delirium tremens and seizures with withdrawal from severe dependence
Can cause intoxication and dependence; for short-term management of withdrawal. For patients with significant liver problems, short acting benzodiazepines (e.g., lorazepam) or one metabolized by glucuronidation (e.g., oxazepam)
Phenobarbital
Barbiturate; sedative hypnotic
Acts as potential substitute when benzodiazepines are ineffective
Respiratory depression a risk with concomitant use
Lead
Clinical symptoms/features and neurologic exam findings
Neuropsychological findings
Laboratory findings
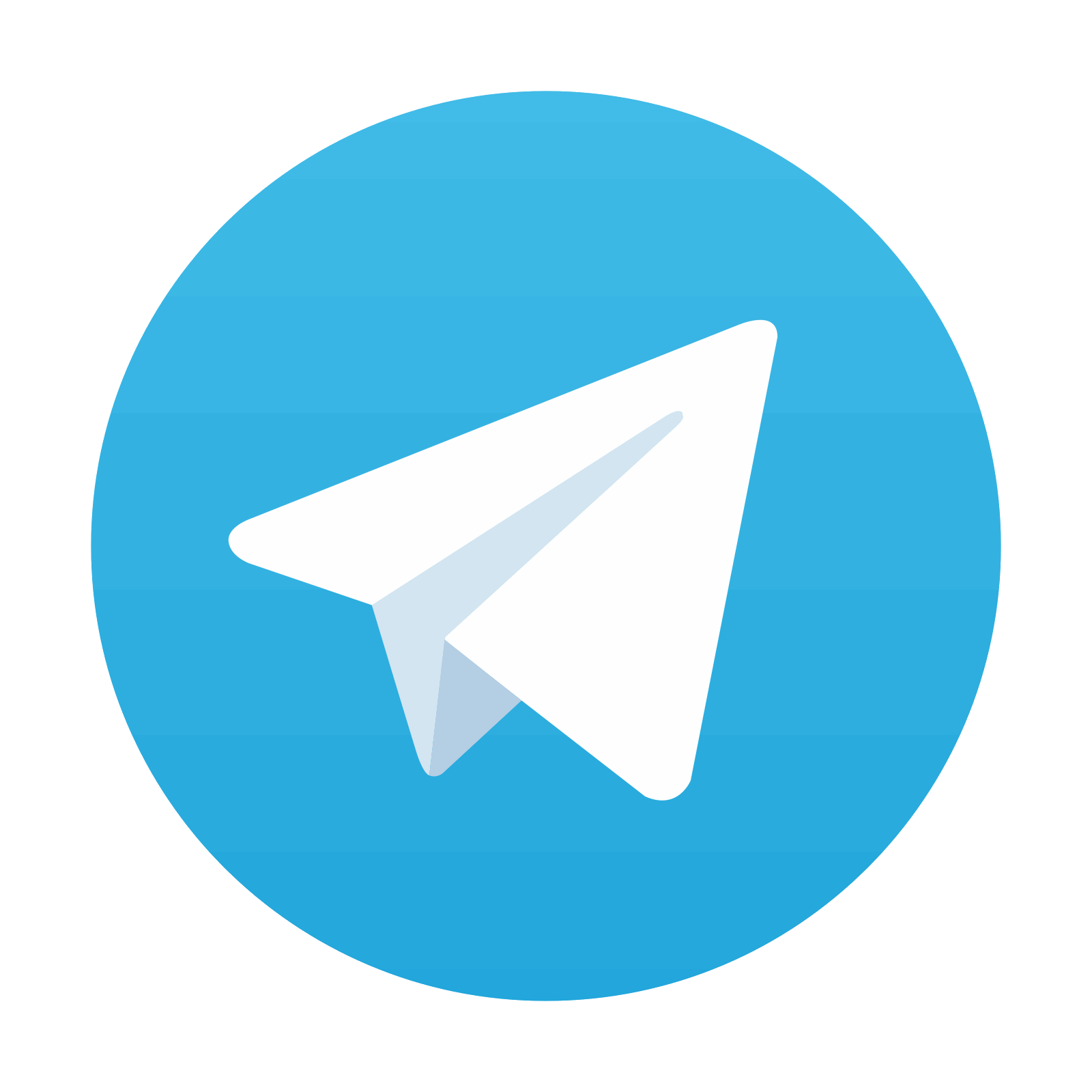
Stay updated, free articles. Join our Telegram channel

Full access? Get Clinical Tree
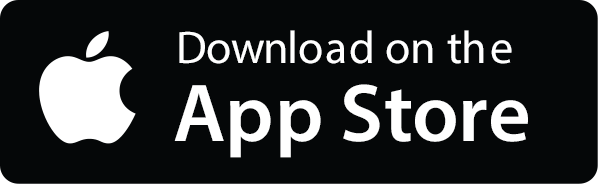
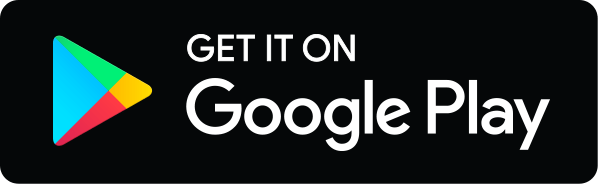