12 Radiology in Cranial Neuropathy
Abstract
When patients present with cranial neuropathies, imaging is often a valuable adjunct to the history and clinical examination. Each cranial nerve has a unique relationship to surrounding vascular, bony, and soft-tissue landmarks, and an understanding of the normal anatomy of cranial nerves is essential to interpreting radiographic images. The clinician must choose an appropriate imaging modality for the study of single or multiple cranial neuropathies and possible associated regional anatomy. The cranial nerve in its entirety is often best depicted with magnetic resonance imaging. Computed tomography is frequently a complementary adjunct to depict foramina and surrounding bony structures. This chapter will review common clinical presentations of cranial neuropathies, associated cranial nerve anatomy in brief, relevant radiographic studies, and important imaging findings.
12.1 Introduction
The skull base is an anatomically complex region encompassing the brain, orbits, cranial nerves (CNs), vessels, soft tissues, and multiple distinct bones. Many parts of this region are not directly accessible for clinical evaluation; thus, imaging plays an critical role in diagnosis and management. While a variety of imaging modalities are available, magnetic resonance (MR) imaging and computed tomography (CT) are the most commonly used studies to evaluate the skull base and CNs. These studies are complementary to each other and often are used in conjunction. 1 , 2 , 3 , 4
MR imaging with contrast is helpful for the evaluation of the soft tissues surrounding the skull base, central nervous system (CNS), and CNs. MR imaging better characterizes soft tissues and is particularly sensitive in its depiction of intracranial, perineural, and perivascular spread of tumors. In addition, MR images can be acquired in any plane, allowing the examiner to tailor the study to the specific structure in question. For discussion purposes of this chapter, MR is the best modality for evaluation of the CNs themselves. On the other hand, CT is the imaging modality of choice for evaluating bony architecture and CN foramina. It is more sensitive in detecting calcifications within lesions and evaluating bony anatomy and pathology including fractures, erosion/dehiscence, sclerosis, and/or hyperostosis. Because these studies are complementary to each other, both MR and CT are often performed in the evaluation of lesions of the skull base and CNs. 3 , 4 , 5 , 6
While imaging is often an important and necessary adjunct to the history and physical examination, the physician must be conscious of associated costs to patients. Price varies greatly among different examination types (CT vs. MRI), insurance groups (public vs. private), regional geography, and practice settings (academic vs. private). See ▶Table 12.1 for an example of cost comparison between different imaging modalities adapted from the Healthcare Bluebook website. Cost discrepancies also exist between practice types, as seen in a study comparing upper-tier academic hospitals to private radiology practices where the cost of a noncontrast head CT could range from $211 to $2,015 with the cost at academic centers significantly on the higher end of the spectrum. 7
Study | Fair price ($) | Low end ($) | High end ($) |
Noncontrast brain CT | 425 | 203 | 1,856 |
Contrast brain CT | 503 | 329 | 2,099 |
Noncontrast brain MRI | 808 | 414 | 3,456 |
Contrast brain MRI | 921 | 465 | 4,474 |
In addition, the physician should consider the potential safety issues associated with imaging studies. Measuring radiation exposure is complex, but the most widely accepted mechanism is for measuring effective dose of partial body irradiations. 8 Effective dose is reported in millisieverts (mSv) and is used to compare different types of radiation and imaging studies. 9 For reference, citizens of the United States are exposed to about 3 mSv (range 1–10 mSv) per year from ubiquitous background radiation. In comparison, a noncontrast brain CT may deliver 2 mSv and contrasted brain CT 4 mSv (range 1–10 mSv). This relatively low radiation dose is not thought to dramatically increase cancer risk. However, it should be kept in mind that multiple examinations over short periods of time may cause DNA damage that could lead to increased cancer risk. 10 , 11
Similarly, there are concerns currently about the possible effects of gadolinium-based contrast material used in MR imaging studies. Concerns include deposition, impaired clearance from the body, and acute physiologic and hypersensitivity reactions. Deposition has been found to occur in skin, brain, bones, and liver. Elevated gadolinium presence in tissues theoretically can cause toxicity, but gadolinium deposition, even within the perivascular spaces of the brain, has not been associated with any known symptoms, and this is not currently well studied. 12 The only clinical entity that is definitively linked to gadolinium deposition is nephrogenic systemic fibrosis that occurs in some patients with renal dysfunction. 12 , 13 , 14 Acute adverse reactions range from mild to severe and include pruritus, edema, nausea/vomiting, chest pain, laryngeal edema, anaphylactic shock, hypotension, and/or seizures. The average acute adverse reaction rate is 0.06 to 0.3% of patients, which is similar or lower than with iodinated contrast agents. 12 Therefore, unintended patient safety outcomes from imaging studies must always be weighed with the clinical usefulness of the examination.
12.2 Cranial Nerve I: Olfactory Nerve
The anatomy of the olfactory nerve is unique. It is the shortest CN and does not arise from the brainstem. Instead, the cell bodies of the olfactory neurons are in the olfactory neuroepithelium, which is located in the upper part of the nasal cavity, the nasal septum, and the inner aspect of the superior nasal concha. The cell bodies transmit odor signals to the olfactory nerves, which pass through the cribriform plate of the ethmoid bone to reach the cranial cavity (▶Fig. 12.1). Nerve bundles form the olfactory bulbs and signals are then transmitted through the olfactory tract to the brain. 15 , 16 , 17

MR imaging is the imaging modality best suited for visualizing the olfactory nerve. A standard examination protocol often includes thin-section T2-weighted images in the fast-spin echo mode in the coronal plane. This view allows for assessment of the anatomy of the olfactory apparatus, visualization of parenchymal lesions, and volumetry of the olfactory bulbs. CT is often a helpful clinical tool for evaluating the bony structure of the cribriform plate. It is most useful for the evaluation of craniofacial trauma in the acute setting. It also can be used to characterize bone disruption in chronic settings such as in cerebral spinal fluid (CSF) leak. Other modalities and imaging techniques, such as X-ray, angiography, and positron emission tomography/CT (PET-CT), are often low yielding in assessing the olfactory nerve and are not often used. 2 , 4 , 16 , 17 , 18 , 19
The clinical importance of the olfactory nerve is exhibited in cases of anosmia. The best first study for the evaluation of isolated anosmia is a sinus CT scan, including coronal plane reconstructions, because it can identify most lesions of the nasal vault and cribriform plate. In more complex cases of anosmia, MR of the brain with close attention to the anterior cranial fossa and sinonasal region can be used. The etiology for anosmia is broad and can include trauma, which transects the cribriform plate (▶Fig. 12.2), congenital disorders like Kallmann’s syndrome in which the olfactory bulb is hypoplastic or absent (▶Fig. 12.3 and ▶Fig. 12.4), inflammatory disorders like sarcoidosis or granulomatosis, and neoplastic disorders, such as meningioma or esthesioneuroblastoma (▶Fig. 12.5 and ▶Fig. 12.6).





12.3 Cranial Nerve II: Optic Nerve
The optic nerve is an extension of the CNS and thus is surrounded by CSF and the meninges. Visual information is received by the rod and cone cells, which transmit signals to the retinal ganglion cells in the back of the globe. Retinal ganglion cells coalesce to form the optic nerve, which then passes posteriorly and medially in the orbital cavity to travel through the optic canal. The nerve then assumes a posteromedial direction to reach the optic chiasm where the optic nerves from each eye come together. In the chiasm, the temporal fibers of each optic nerve pass posteriorly and the nasal fibers of each optic nerve cross the midline to join the contralateral optic tract. Nerve fibers in each optic tract then pass visual signals along to the primary visual cortex of the brain through the optic radiations. 15 , 16 , 17
MR is uniquely suited to image the entirety of the visual pathway from the globe to the visual cortex of the brain. A standard protocol for imaging the optic nerve, optic chiasm, and surrounding CSF involves T1-weighted axial and coronal images, and heavily T2-weighted images and short-tau inversion recovery (STIR) images. Intracranial portions of the optic nerve can be imaged using standard T1- and T2-weighted images (▶Fig. 12.7). 2 , 4 , 16 , 17 , 20 Complementary information can be provided by CT, which is a particularly sensitive modality for identifying fractures of the orbit and skull base that may affect the nerve. CT may also be used to evaluate the extraocular muscles, detect conal mass lesions, and characterize edema. 21 Other less common but useful imaging studies include optic coherence tomography (OCT) and orbital B-scan ultrasound. OCT can be used to distinguish retinal pathology versus optic neuropathy. Orbital B-scan ultrasound can be valuable in assessing abnormalities in which the optic nerve is thickened. 21

In cases of suspected trauma, CT is the most appropriate first choice for imaging. CT allows for assessment of orbital and/or skull base fractures, orbital hematomas, intracranial injuries, and possible radiopaque foreign bodies (▶Fig. 12.8). If there is no evidence of a metallic foreign body, MR may be useful to assess avulsion of the optic nerve, optic nerve sheath hematoma, axonal injury, or nerve ischemia. 20 , 21

Patients with diseases of the visual pathway or surrounding structures will often present clinically with vision loss. The overall differential diagnosis for visual loss is broad, and the type of visual field defect (monocular loss, bitemporal hemianopia, homonymous hemianopia, quadrantanopia, etc.) may help the physician narrow the differential. This section will provide a brief glimpse of optic nerve pathology, specifically demyelinating disorders and neoplasms.
Multiple sclerosis (MS) is a commonly recognized demyelinating disease that is known for lesions that are disseminated in space and time. Many patients with MS suffer visual disabilities at some point in their disease. Specifically, the initial diagnosis of MS may result from the workup for optic neuritis that is seen on MRI as edema and avid optic nerve enhancement (▶Fig. 12.9). Various other autoimmune and infectious processes can also cause an optic neuropathy. Ophthalmic manifestations can be seen in cases of sarcoidosis, and rarely systemic lupus erythematosus. In all the above-listed circumstances, MRI in itself is often not diagnostic of the disease and further serologic studies, neuroimaging, and/or body imaging may need to be performed. 21 , 22 , 23

There are many neoplasms that can involve the visual apparatus either directly or indirectly by compression. For patients with a suspected neoplasm, MR is the imaging modality of choice because it allows for the most complete evaluation of the optic nerve, optic chiasm, and extent of intraorbital and intracranial involvement. However, in many cases, CT is also necessary for differentiating between tumor types as it may reveal findings that are not detected on MR. 20 , 21 , 22 For example, MR in conjunction with CT is useful for differentiating between optic nerve gliomas (ONGs) and optic nerve sheath meningiomas (ONSMs). On MRI, ONGs often have a fusiform appearance and the optic nerve may appear kinked at the midorbit. ONGs are typically isointense on T1-weighted images and isointense to hyperintense on T2-weighted images (▶Fig. 12.10). In contrast, ONSMs may be tubular, globular, fusiform, or focal on MR. They are hypointense to isointense on T1-weighted images and slightly hyperintense on T2-weighted images. CT is particularly valuable in assessment of ONSMs, as it may show dural tail, CSF cleft, bony erosion, and/or hyperostosis in the region surrounding the lesion. ONSMs also characteristically exhibit tram track or bull’s eye signs, which are hyperdense enhancement of meninges surrounding a hypodense nerve, and this can be seen on both MR and CT (▶Fig. 12.11). 20 , 21 , 22


12.4 Cranial Nerves III, IV, and VI: Oculomotor, Trochlear, and Abducens Nerves
For the purposes of this chapter, these three ocular nerves will be discussed together. High-yield anatomy of the oculomotor, trochlear, and abducens nerves is reviewed briefly in this section. The oculomotor nerve arises from the anterior surface of the midbrain and extends anteriorly and laterally, passing between the posterior cerebral artery (PCA) and superior cerebral artery (SCA) vessels (▶Fig. 12.12). The nerve then enters the oculomotor cistern within the superior and lateral wall of the cavernous sinus, and then continues superiorly and laterally through the superior orbital fissure. It passes through the annular tendon, and then branches into a superior and inferior division, which then supply a majority of the extraocular muscles including the medial, lateral, dorsal rectus, and ventral oblique muscles as well as the levator palpebrae superioris muscle. 15 , 16 , 17

The trochlear nerve has the longest intracranial cisternal course and is the only nerve to arise from the dorsal aspect of the midbrain, and it then crosses midline to the contralateral side, traveling lateral to the midbrain (▶Fig. 12.13). The nerve then passes through the lateral wall of the cavernous sinus, exits through the superior orbital fissure, passes superior to the annular tendon, and terminates in the superior oblique muscle. 15 , 16 , 17

The abducens nerve arises from the pontomedullary junction off midline, then extends superiorly to enter the abducens cistern (▶Fig. 12.14). The nerve then passes through the cavernous sinus, then the superior orbital fissure, and ends in the lateral rectus muscle. 15 , 16 , 17

The oculomotor, trochlear, and abducens nerves can be divided into segments. Each nerve has four segments: intra-axial, cisternal, cavernous, and extracranial or intraorbital. The abducens nerve also has a fifth segment, which is intradural. MR is the best imaging modality for visualization of each one of the ocular nerves. Additionally, there are specific MR imaging sequences (thin-section T2-weighted or CSF bright) that can be reconstructed in oblique planes tailored to imaging each nerve segment (▶Fig. 12.2 , ▶Fig. 12.3, and ▶Fig. 12.14). Complementary CT can assist to better examine the skull base, osseous borders of the CN foramina, and the orbital walls. 24 , 25 , 26 , 27
Patients with lesions involving the ocular nerves often will present clinically with diplopia. It is important to note that patients are much less likely to present with an isolated ocular nerve palsy. More often, ocular nerve palsies present in conjunction with multiple other cranial neuropathies, and this constellation of symptoms may lead the clinician to a more specific diagnosis, and more focused imaging evaluations.
A complete oculomotor nerve palsy causes the eye to stay locked in a “down and out” position. Thirty percent of isolated oculomotor nerve palsies are caused by aneurysms, and these often arise from the origin of the posterior communicating artery (PCOM; ▶Fig. 12.15). Pupillary involvement is classic in these patients. In the cases where an aneurysm is highly suspected, with this clinical presentation, the best first imaging study remains MRI, which can demonstrate a variety of lesions, with an MR angiogram. A CT angiogram and/or a catheter angiogram can also assist with vascular evaluation. Additional causes of an isolated third nerve palsy include lesions near the brainstem. These lesions may include arteriovenous malformations and cavernous hemangiomas, which can bleed and cause palsy, infarctions, brainstem gliomas, schwannomas, demyelinating lesions, or traumatic shearing injuries. 4 , 24 , 25 , 27

Isolated trochlear nerve palsy is uncommon. However, if it were to occur, the patient would present with an oblique diplopia that worsens when the affected eye looks nasally. Because this nerve has the longest cisternal segment, it is most likely to be affected by shearing or stretching forces as the result of trauma, and head trauma is the commonest cause of injury. 25 Conventional MR imaging may demonstrate atrophy of the superior oblique muscle with prolonged injury, but high-resolution MR imaging is needed to visualize the nerve itself. Rarely, the nerve palsy may be the result of congenital absence or affected by infection, infarction, hemorrhage, or demyelinating lesions. 4 , 24 , 25 , 27 , 28
Isolated abducens nerve palsy causes a horizontal diplopia. Syndromes that cause elevated intracranial pressures may cause abducens palsy with compression of the nerve as it travels over sharp ridges of the clivus and petrous temporal bone. Similarly to the other oculomotor nerves, isolated abducens palsy may be caused by infection, infarction, hemorrhage, tumors, or demyelinating lesions. 4 , 24 , 25 , 27
Patients who present with multiple CN palsies, specifically CNs III, IV, V, and VI should draw high suspicion for involvement of the cavernous sinus. Lesions in this region may affect any one of these ocular nerves but commonly involve all three. The differential for cavernous sinus lesion is broad and may include carotid aneurysms, carotid dissection, carotid-cavernous fistula, cavernous sinus thrombosis, infarction, infection, tumor, and idiopathic orbital inflammatory disease (IOID). Less commonly, patients may have pituitary adenoma or lesions from the paranasal sinuses or nasopharynx that may invade the cavernous sinus. 4 , 24 , 25 , 27 Patients with cavernous sinus thrombosis typically exhibit symptoms of eyelid edema, conjunctival injection, orbital pain, and proptosis. With high clinical suspicion, the best initial imaging study is contrasted MR with MR venogram as it will show absence of enhancement within the cavernous sinus (▶Fig. 12.16). 4 , 24 , 25 , 27 A rare but often cited cause of cavernous sinus lesion is IOID (or Tolosa–Hunt syndrome). Patients characteristically present with severe unilateral headaches, orbital pain, and ophthalmoplegia. The classic imaging finding in this syndrome is asymmetric cavernous sinus enhancement from inflammation of the cavernous sinus. Patients exhibit characteristic MR imaging findings and often require serial imaging to assess for response to steroids (▶Fig. 12.17). 24 , 25 , 27 , 29


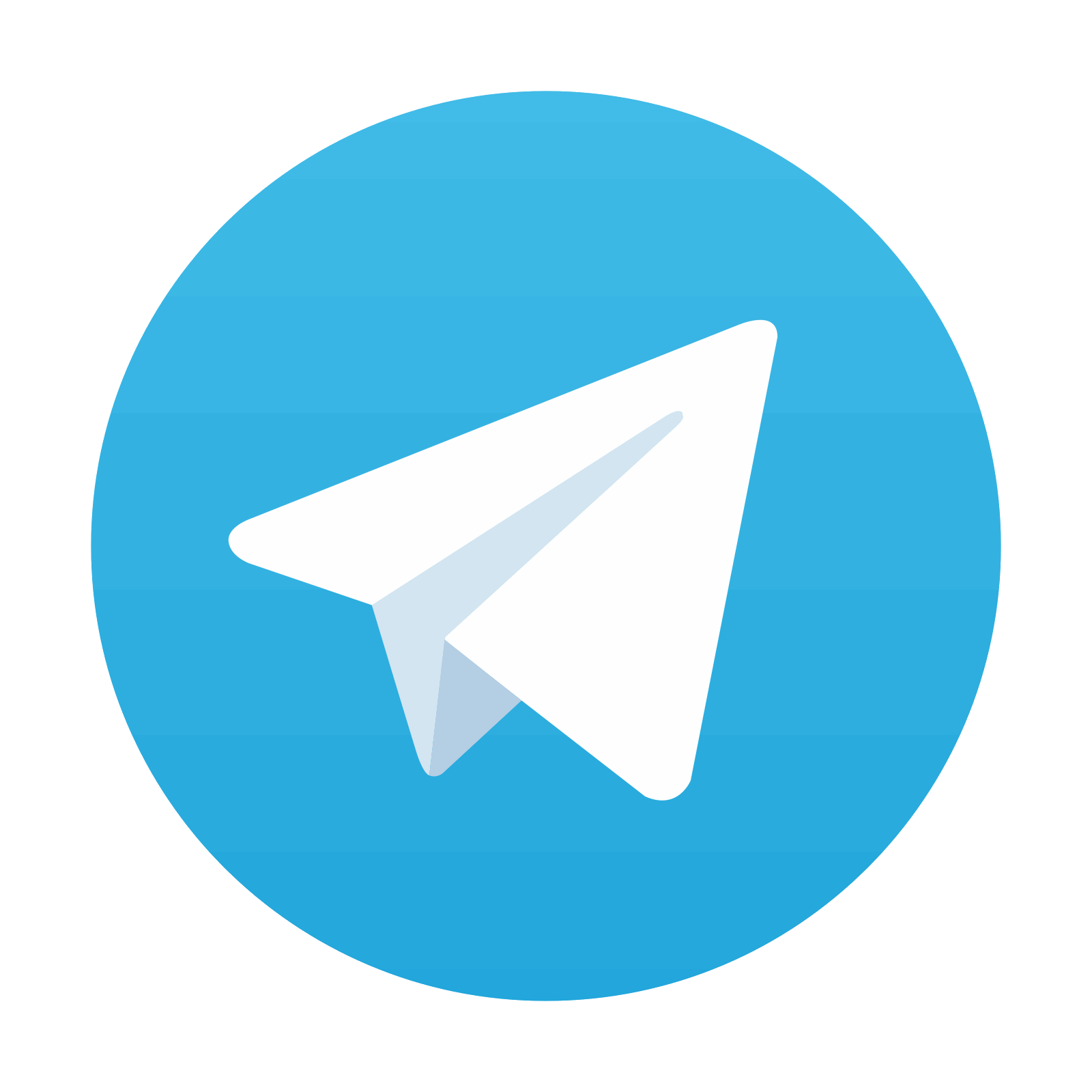
Stay updated, free articles. Join our Telegram channel

Full access? Get Clinical Tree
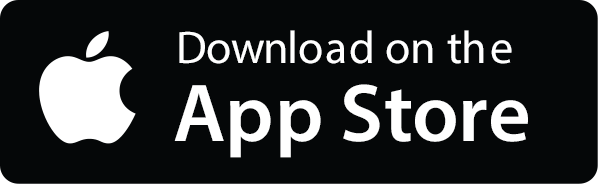
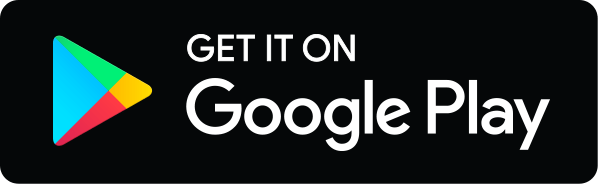