12 Spine Fusion
12.1 The Bone Graft
The bone graft and the resulting bony fusion are the components of a successful spine stabilization operation that lead to the ultimate stability of the spine. No matter how secure an internal fixation device may appear to be, it will eventually fail unless bony fusion and stability are achieved. There exists a proverbial “race” between failure of the implant and the acquisition of bony fusion. After a fusion procedure, the implant and its interface with bone become progressively weaker and the bony union usually becomes stronger—unless, obviously, bone fusion ensues (Fig. 12.1). Therefore, most internal fixation techniques should be applied in conjunction with a bone graft. Of course, an exception exists when a spinal instrumentation procedure is performed without a fusion operation in cases of decompression and/or stabilization for spine cancer.

Ventral interbody bone grafts provide superb ultimate strength characteristics. 1 – 3 They are placed in the weight-bearing region of the spine along the instantaneous axis of rotation (IAR). Weight bearing itself promotes healing and bony fusion, according to Wolff’s law. 4 Care must be taken, however, to prevent progressive deformation following the placement of a ventral interbody fusion. Stauffer and Kelly have reported a high incidence of angular deformities following ventral fusions for cervical spine trauma in patients treated without instrumentation. 5 Dorsal stabilization procedures may be necessary (either alone or in combination with ventral decompression and fusion) to achieve acceptable stability and neural element decompression in such cases. Ventral plating techniques, likewise, may be used for this purpose. Without dorsal stability augmentation, however, their use for this purpose must be considered carefully because their ability to resist flexion is much less than their ability to resist extension.
Dorsal bone grafts generally are not, by themselves, weight-bearing. Spine flexion (which causes flexion ventral to the IAR) causes distraction of the segments to be fused (dorsal to the IAR; see Chapter 1). Unless ventral axial load–resisting support is provided (i.e., by a ventral intervertebral bone strut graft) or already exists (e.g., in patients with cervical locked facets without vertebral body fracture), dorsal bone grafts should be avoided unless an accompanying instrumentation construct provides the needed stabilization support. If the bone graft is applied in association with tension-band fixation in a flexion construct (such as with interspinous wiring), ventral axial load–bearing support must be provided if ventral weight-bearing ability is suspect.
Frequently, stabilization procedures are performed after decompressive operations. The reduction of a ventral mass impinging on the spinal cord, therefore, frequently requires an operative approach in addition to that used to place the dorsal instrumentation device. Furthermore, so that the dural sac is decompressed before spine manipulation, the ventral (decompression) aspect of the operation should be performed first (before placement of the dorsal instrumentation device). 6 In situations in which spinal distraction is the desired mode of application, the interbody bone graft should not be placed until the dorsal instrumentation devices have been applied (for fear of adversely altering spinal biomechanics by loosening the already-placed bone graft). 6 Theoretically, in this case, the most appropriate order of procedures should be as follows: first, decompression of the neural elements and loosening/relaxing of the spine by discectomy and corpectomy (e.g., a relaxing procedure); second, placement of the ventral bone graft. 6 If spinal compression is the desired mode of application, it may be desirable to place the interbody bone graft strut first (Fig. 12.2).

Bone grafts differ significantly regarding their integrity. 7 This is related to graft source, autograft versus allograft, allograft preparation techniques, and so forth. The greater the ratio of cortical to cancellous bone, the greater the axial load–bearing ability (and the less the implant fusion potential). The greater the surface area of contact, the greater the resistance to pistoning.
Allograft processing, in general, adversely affects graft integrity. Ethylene oxide sterilization, however, does not affect compression strength. Bone that is frozen then thawed is superior to freeze-dried bone for torsion and bending resistance. Of note, slow rehydration with saline improves the biomechanical characteristics of freeze-dried bone.
12.2 Ventral Spinal Fusion
Much thought should be given to selection of the specific location of ventral bone graft placement, particularly in the sagittal plane. The location of the interbody bone graft significantly affects the biomechanical efficacy of the construct. In general, for the optimization of axial load–resisting ability and torso support, the optimal location for interbody bone graft placement is in the vicinity of the IAR in the sagittal plane. This generally is also the location of the neutral axis (particularly if dorsal spinal element stability is deficient). The neutral axis is that region of the spine where flexion and extension do not significantly displace points located within the limits of the axis (see Chapter 7). Usually, it is located at the junction of the anterior and middle columns of Denis. 8 If dorsal spinal element stability is adequate, a slightly more ventral location for interbody bone graft placement may be optimal (see Chapter 7 and Fig. 12.3). In this situation, axial loads can be more effectively distributed between the strut graft and the existing dorsal element structures. 9 , 10 In general, the placement of ventral interbody struts in the anterior column region facilitates load sharing as described and prevents kyphotic deformation. Furthermore, the ventral cortex can bear axial loads more effectively than can the middle column. 11

The placement of a ventral interbody fusion can provide a substantial increase in axial load–resisting ability. 2 The bony strut itself and the sites of attachment to the vertebral bodies (purchase sites) must be strong to offer such support. The needed strength may be lacking, for example, when thin iliac crest, rib, or morcelized bone is used as the graft substrate. Yet, stronger fusion masses, such as the fibula, may penetrate (farther than desired) through the accepting purchase sites in the rostral and caudal vertebral bodies, much as a toothpick may penetrate a piece of expanded polystyrene foam. For this reason alone, cages, ceramics, and even hydroxyapatite should be carefully considered, particularly for an osteoporotic spine. The resistance of an interbody strut to vertebral body penetration (subsidence) is proportional to the cross-sectional area of the strut–vertebral body interfaces (Fig. 12.4a) and to the stresses at the interfaces (Fig. 12.4b–d). A bone graft that is of lesser integrity than the vertebral bodies may fail. Therefore, provided that there are no extenuating circumstances, the consistency and integrity of the bone graft should be similar to the bone of the vertebral bodies that accept it (Fig. 12.5). An exception to this may be the case in which the endplates of the vertebral bodies themselves may be used for axial load–resisting support (Fig. 12.6).



The acute stabilizing effect of an interbody bone graft depends partly, among many other factors, on the angle that the disc interspace forms with the horizontal plane when the patient is in the upright position. If the angle is zero (i.e., if the interspace is parallel to the floor when the patient is standing), axial loads will not produce any shear forces at the level of the fusion (stable vertebra). If, on the other hand, the disc interspace and fusion site are more vertically oriented (as in the lumbar spine, particularly at the lumbosacral junction), a shear force is added to the axial load (Fig. 12.7). The axial load promotes bone healing; the shear forces disrupt it. This may explain, in part, the not uncommon fusion failures observed with the posterior lumbar interbody fusion (PLIF) technique without dorsal stabilization supplementation, particularly in the low lumbar and lumbosacral region. 12 Of note is that ventral cervical discectomies with and without fusion have similar clinical results, 13 thus diminishing the significance and relevance of fusion acquisition—at least theoretically.

Interbody fusion operations, including the PLIF and transforaminal lumbar interbody fusion (TLIF) operations, can use the phenomenon of parallelogram distraction to advantage. This phenomenon is based on the inherent strength of the fibroligamentous complex surrounding the vertebral body and connecting one vertebra to its neighbor—that is, the annulus fibrosus and the ventral and dorsal ligaments. Spondylolisthesis, by its nature, results in a parallelogram-like distortion of these structures and the adjacent vertebral bodies (Fig. 12.8a). This is accompanied by a stretching of the fibroligamentous complex surrounding the vertebral body or, more likely, by an associated compensatory loss of disc interspace height. By taking advantage of the integrity of the fibroligamentous complex, the surgeon can distract the spine, thereby reducing the translational deformation in the sagittal plane. A bone graft or other interbody strut/cage alternatives can then be used to maintain this alignment of the vertebral bodies, by acting as a spacer, until bony union takes place (Fig. 12.8b).

Suboptimal mortise crafting and interbody strut/cage “fitting” are perhaps the most common preventable errors leading to ventral interbody bone graft failure. The mortise must be cut deep enough and the bone graft must fit snugly into the mortise in such a manner that dislodgement is unlikely (see Fig. 12.6).
The use of a fibular strut graft for interbody grafting may have the advantage of providing sufficient length for long fusions in selected cases. The surgeon must recognize, however, that the fibula has a much higher ratio of cortical to medullary bone than does the vertebral body; thus, the aforementioned telescoping complication can occur. This, in turn, can result in graft collapse or cutout. Placement of the graft near the endplate, for additional axial load–bearing support, may help prevent such complications (see Fig. 12.6). Note that fusion healing in this circumstance may be less vigorous because of the smaller area of contact between the graft and the vertebral body. The origin of the graft (e.g., allograft vs autograft) also plays a role in the strategy determination process. 14 – 19 Other factors obviously play a role, as well. 20 – 28 Many of them pertain to bony integrity. 29
12.3 Dorsal Spinal Fusion
Dorsal spinal fusions are not well situated mechanically to resist axial loads—that is, they do not provide substantial acute axial support for the spine. In addition, because they do not bear significant axial loads, they may be expected to involute with the passage of time. 30 Dorsal spinal fusions can provide acute support of the spine only if secured in some way to the spine, as in dorsal wiring and fusion procedures. These are most often used in the cervical region. 31 Such fusion operations, however, are not practical in the lumbar region because of the size of the spinal segments and the obligatory stresses placed on the spine in that region.
Forces that enhance bone healing participate significantly in the fusion process. They explain the difference between the fusion rates of ventral interbody fusions and dorsal non–weight-bearing fusions (fusion being significantly more rapid in the former than in the latter). This is so because of the ability of bone healing–enhancing forces (compression) to encourage interbody fusion. 4 Dorsal fusions are not exposed to these bone healing–enhancing compression stresses, and autograft containment strategies do not seem to help. 32 Therefore, healing and fusion rates are somewhat diminished. In fact, dorsal bone fusion (which is non–weight-bearing and so not exposed to bone healing–enhancing stresses) volume diminishes with time (> 50% in 18 months). This also is not affected by spinal instrumentation or by pathology. Also of note is that the greater the volume of initial bone graft, the larger the fusion mass at 18 months postoperatively. 30 The source of the bone graft also affects the success of fusion. 33
If an axial load is borne by a spine with an accompanying dorsal fusion, the bone fusion mass itself does not bear a load. In fact, the fusion mass is usually placed under some tension (distraction) during axial load bearing.
Dorsal fusion masses may be difficult to assess radiographically. Creative imaging strategies may be of some assistance. 15
12.4 Fusing the Immature Spine
Fusing the immature spine has been observed to be associated with asymmetric spine growth. The term crankshaft phenomenon describes the continued progression of scoliosis after dorsal fusion. This phenomenon is due to continued ventral spine growth. Some believe that an open triradiate cartilage predicts the occurrence of this phenomenon postoperatively. It appears, however, that the simultaneous observation of an open triradiate cartilage and the performance of surgery before or during peak height growth velocity is a strong predictor of this phenomenon. Later surgery, in fact, is a strong negative predictor. 34 , 35
12.5 Pseudarthrosis
Pseudarthrosis is often difficult to diagnose. A variety of techniques have been used to establish the presence or absence of pseudarthrosis. 36 – 53 Perhaps computed tomography is the most accurate. 54 Prudent decision making and clinical judgment, however, must be liberally used in difficult cases. The outcome of fusion is, likewise, difficult to quantitate and assess. The development of outcome assessment tools and models for objective assessment would seem prudent. Blount et al developed and validated such a model. 55 Finally, the treatment of symptomatic pseudarthrosis presents unique challenges, which can be overcome by applying the most appropriate operation for each given clinical circumstance. 56
12.6 Bone Grafts and Bone Substitutes
The surgeon has multiple options regarding the choice of bone fusion substrate. 57 Broad categories include autograft, allograft, and bone substitutes. Autograft is usually considered to be associated with the greatest success rate, particularly when used in conjunction with appropriate spine fixation devices, 58 although the use of no graft material with a cage has been shown to be associated with success, as well. 59 This observation most certainly challenges intuition regarding the acquisition of fusion. Complications at the bone graft harvest site present a deterrent to the use of autograft. Despite common belief, a relatively low rate of long-term complications associated with autograft harvest can be reasonably expected. 60 Modifications of the standard technique, the use of alternative sites for harvest, and an awareness of unique anatomical details may be used to further diminish complications at the graft harvest site. 61 – 63 Nevertheless, careful scrutiny of the clinical results is warranted. 64 The management of donor site pain, when present, can be challenging. Creativity is often required. 65
Allograft presents an appealing alternative, particularly in light of the elimination of the need for bone graft harvest. 66 Fusion rates, however, are diminished. Of note, it appears that there exists a nearly zero overt risk associated with the use of allograft bone in spine surgery from a disease transmission perspective. 67
Bone extenders, such as beta-tricalcium phosphate and calcium sulfate, have been shown to provide some utility regarding fusion acquisition. 68 – 70 One must nevertheless carefully consider the use of bone extenders and weigh their advantage against a diminished fusion rate compared with autograft. Obviously, if autograft harvest does not provide adequate bone volume, alternatives must be considered.
Structural bone alternatives, such as hydroxyapatite, have shown utility as vertebral spacers and struts. However, hydroxyapatite, in particular, is associated with suboptimal strength characteristics. 71 Such must be taken into consideration before clinical use.
Bone morphogenetic proteins (BMPs) should be considered as bone graft substitutes or even as bone graft extenders. The literature presents an abundance of information regarding BMP for fusion enhancement. Several BMPs have been developed and employed in the laboratory and clinical arenas. Recombinant BMP-2 has shown the greatest promise, 72 – 74 whereas BMP-7 has not. 75 Complications associated with carriers, dosing, bony overgrowth, soft tissue inflammation, and the risk for cancer have been either suggested or observed. 76 , 77 Although the future regarding the clinical application of BMPs still appears bright, their routine clinical application is currently being seriously questioned. Creativity regarding BMP delivery may lead to additional applications in years to come. 78 Research is ongoing. 79 , 80 Molecular biological “delivery” alternatives may play a clinically relevant role in the future. 81
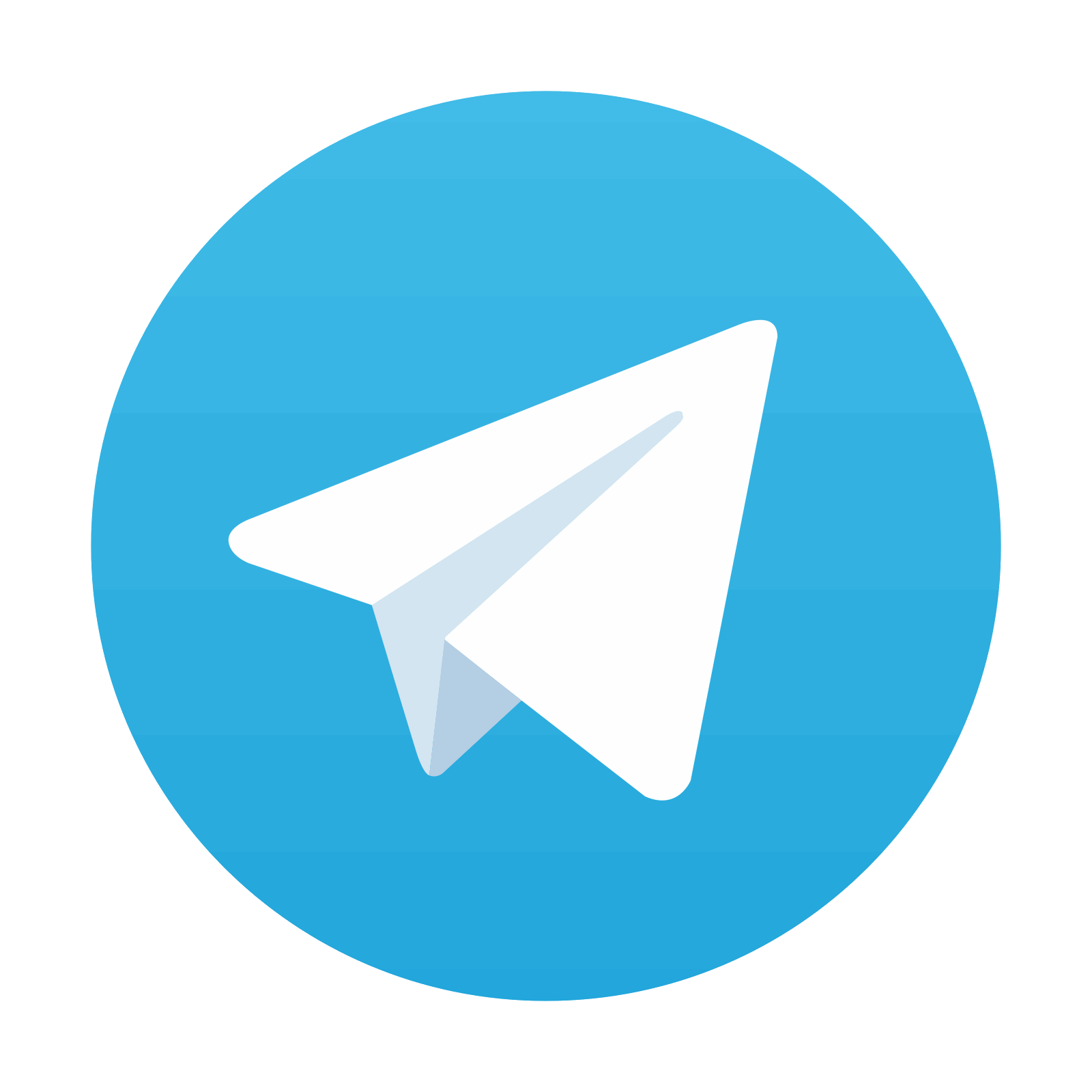
Stay updated, free articles. Join our Telegram channel

Full access? Get Clinical Tree
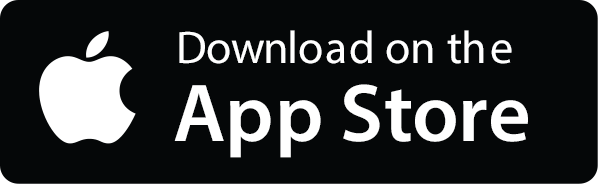
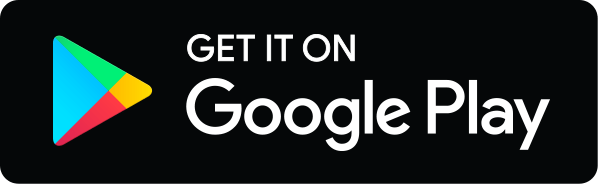