12 TECHNIQUE AND NUANCES OF SURPASS STREAMLINE FLOW DIVERTER
Abstract
Manufacturing of the Surpass flow diverter (FD) is based on scientific and biological concepts that were developed over the past three decades in endovascular treatment of brain aneurysms. The braided tubular chromium-cobalt implant is engineered to address regional flow conditions and fine-tune the needs of porosity and mesh density of the implant. Braid angle and introduction of larger number of wires provide biomechanical stability, consistent and reliable deployment, and prevent kinking and torque of the Surpass FD even under extreme conditions. Development of the Streamline delivery system addresses challenges inherent to the neurovascular system and allows for a torque-free, over-the-wire deployment of the device. The maintenance of continuous distal access allows for the recapture and redeployment of the device. In tortuous vasculature, the use of a triaxial access system is required.
12.1 Introduction and Basic Concepts of Flow Diversion
The introduction of flow diverters (FDs) in the management of intracranial aneurysms (IAs) is truly a great example how accumulated knowledge in physiology, biomedical engineering, and clinical science was used to solve an important health problem. No other IA treatment has involved a more rigorous scientific effort and scrutiny than the concept of flow diversion. The final design of the Surpass FD (Stryker, Kalamazoo, MI), one of the flow diverters in clinical use, was based on a careful evaluation of the hydrodynamics involving the parent artery–aneurysm complex using Computational Fluid Dynamics (CFD), Laser-Induced Fluorescence (LIF), and Particle Imaging Velocimetry (PIV) studies, along with preclinical in vivo studies. 1 , 2 , 3 , 4 , 5 These studies showed that the reconstitution of the blood flow of the diseased segment to near-physiological condition was dependent on the FD design.
When properly engineered, the inflow from the parent artery into the aneurysm is reduced sufficiently to form a stable clot within the aneurysm pouch while maintaining the flow within covered side branches and perforators. 5 Various factors were critical in manufacturing Surpass, such as the choice of the metal alloy. Other parameters included the manufacturing modality used (i.e., laser cut, knitting, and braiding) that determines the flexibility and kink resistance of the product and the vessel wall apposition in tortuous intracranial arteries. Radial and crush force is pivotal to maintain sufficient outward force and mechanical stability during the cardiac cycle to prevent device migration. However, excessive radial forces may create undesired intimal response. The type of material used should also withstand fatigue-related fractures and galvanic corrosion over time.
Given these challenges and its long history of use in the medical domain, the Surpass FD utilizes a cobalt-chromium alloy and fine-wire braiding to control the mesh size of the device. Cobalt-chromium is a well-known alloy for its excellent biocompatibility and fatigue properties, corrosion resistance, and mechanical strength. 6 Additionally, the use of chromium-cobalt braided FDs in the hemodynamically challenging, elastase-induced aneurysm model in rabbits was very favorable. 7 , 8 , 9 The Surpass FD resisted migration and fracture when placed close to the heart in the rabbit subclavian artery. This location is subjected to excessive motion during each cardiac cycle (heart rate on average of 180 beats/min) resulting in a biomechanical stress not encountered in the human cerebrovasculature.
12.2 Surpass FD Implant
CFD, as well as semi-quantitative LIF, followed by quantitative PIV methods were utilized to refine and optimize the properties of the Surpass FD. The goal was to optimize blood flow reduction into the aneurysm and increase the circulation time of blood within the aneurysm, while maintaining flow through side branches and perforators. 5 Two major parameters were found to be important for FDs to be hydrodynamically effective: (1) porosity and (2) pore or mesh density of the implant. The in vivo and in vitro hemodynamic studies indicated that there was a fine-tuning required to balance the porosity (metal free/metal area) and pore or mesh density (number of pores/mm 2 ) of the FD to optimize the effect on flow reduction. 2 , 3 , 4 , 5

The FD creates a resistance to the flow at the aneurysm neck and subsequently decreases the hydrodynamic circulation and the peak and mean kinetic energy transfer from the parent artery into the aneurysm with each pulse cycle. The FD modifies the predominantly convective flow to a more diffusive form that eventually leads to aneurysm thrombosis and occlusion. 7 , 8 , 9 Before finalizing the Surpass FD, various samples were manufactured and tested for their hydrodynamic properties with follow-up testing in an in vivo rabbit elastase aneurysm model ( Fig. 12.1a, b).

The Surpass FD is braided out of cobalt-chromium alloy wires with small amounts of Nitinol (Ni-Ti) to form a tubular structure. The braid angle β ( Fig. 12.2) and the number of wires also help preserve the structural integrity of the device, the diamond cell shape, and mesh density, regardless of large variabilities in the arterial diameter over the length of the construct ( Fig. 12.2, Fig. 12.3). Consistent mesh density ensures a more even effect on flow reduction, preventing pockets of increased flow impingement zones within the aneurysm. Various lengths and diameters of the Surpass FD implants are available for the clinical use. Unlike other products, the device is manufactured in diameters of 1-mm increments. The number of wires for each device varies depending on the device diameter to maintain biomechanical stability and a consistent reduction in aneurysm inflow (up to 96 wires for the largest device diameter of 5.3 mm; Fig. 12.4, Fig. 12.5, Fig. 12.6). Additionally, the wire diameter used for braiding ranges, for currently available implants, from 25 to 32 µm. The diameter is limited by the alloy chosen and the risk of material fracture during fine-wire braiding. The mesh density of the device ranges from 21 to 32 pores/mm 2 . Twelve platinum-tungsten wires are braided into the implant to aid visibility under X-ray fluoroscopy without interfering with the mesh density or the biomechanics of the device. As recently shown, this increased number of wires may result in an earlier and more complete endothelialization of the diseased vessel. Interestingly, among other cellular mechanisms, CD + 34 progenitor cells are involved in endothelialization. 10





The percentage foreshortening of a Surpass FD varies, like for all braided implants, and depends on the device diameter and length, with approximately 38, 42, and 26% foreshortening—under nominal diameter—experienced for 3-, 4-, and 5-mm systems, respectively. However, unlike with other similar products, Surpass FD foreshortens significantly less due to the braid angle and larger number of wires. These features also prevent kinking and torquing of the FD and facilitate a more reliable and consistent device opening in tortuous vasculature.
Surpass FD has been determined to be MR conditional at 3T or less magnetic field strength according to the terminology specified in the American Society for Testing and Materials (ASTM) International, Designation: F2503; Standard Practice for Marking Medical Devices and Other Items for Safety in the Magnetic Resonance Environment. A patient can safely be scanned immediately after placement of Surpass FD under the following conditions: (1) static magnetic field of 3 Tesla or less; (2) maximum spatial gradient magnetic field of 720 Gauss/cm, a higher value for the spatial gradient magnetic field may apply if properly calculated; and (3) maximum MR system–reported, whole-body–averaged specific absorption rate (SAR) of 2 W/kg for 15 minutes of scanning (per pulse sequence). 11 In nonclinical testing, the FD produced an increase temperature of 6.0°C or less using an MR system–reported, whole-body–averaged SAR of 2 W/kg for 15 minutes (per pulse sequence) of scanning in a 3-Tesla MR system. Although MRI can be used, the image quality may suffer in the area adjacent to and within the center of the implant.
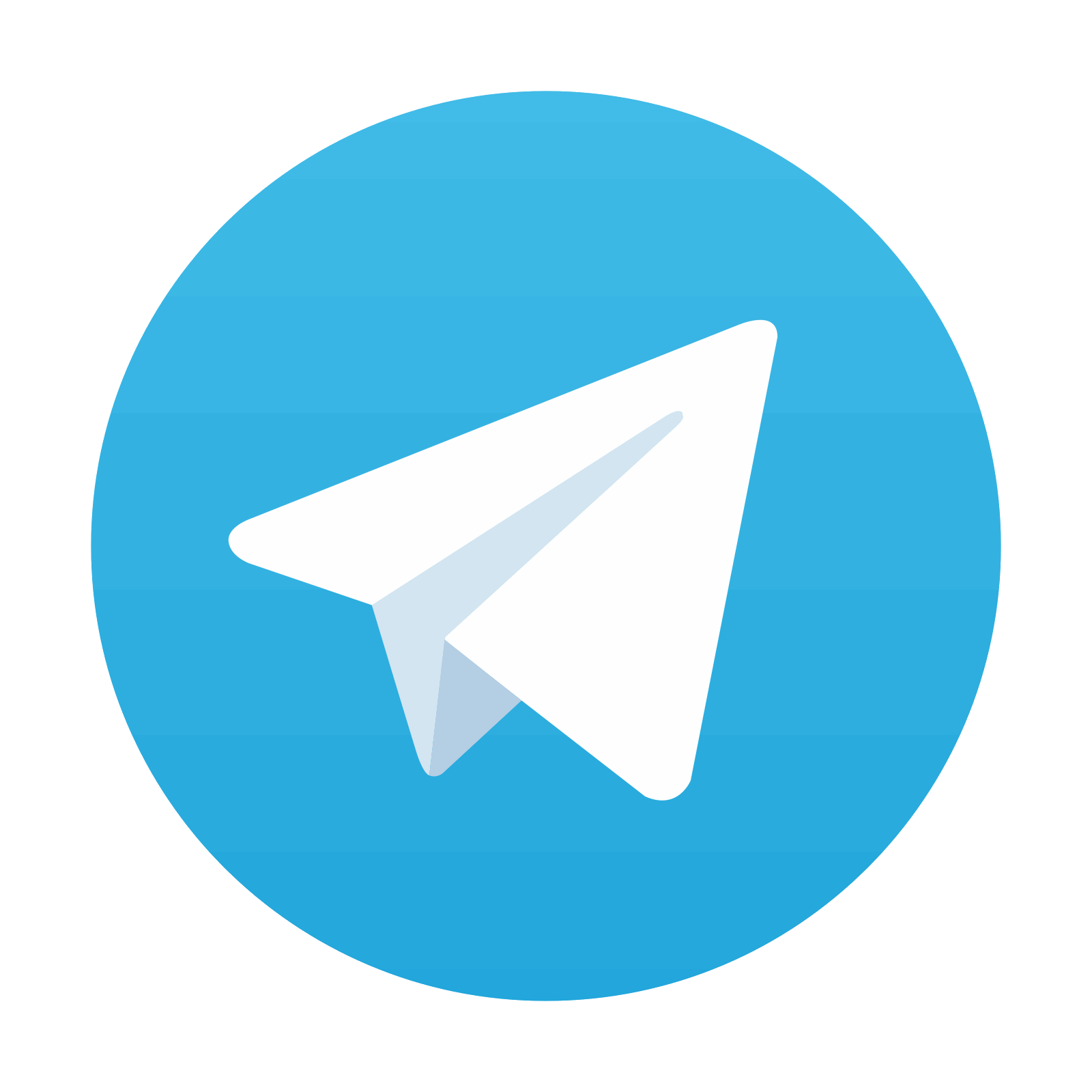
Stay updated, free articles. Join our Telegram channel

Full access? Get Clinical Tree
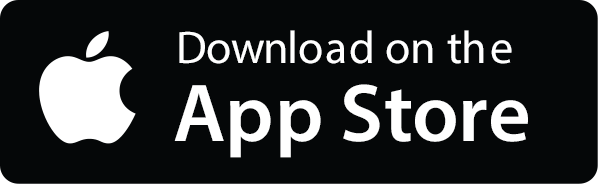
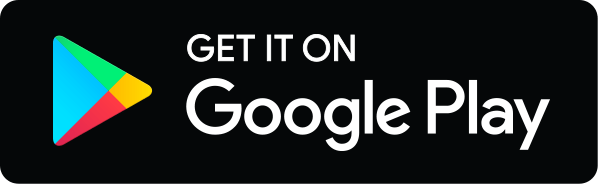