13 Neurosurgical Causes of Scoliosis
Abstract
The most common cause of scoliosis is idiopathic. However, in infantile and juvenile scoliosis, up to 20% of patients may harbor an underlying neurologic cause. 1 , 2 , 3 , 4 Recognition of a potentially reversible underlying neurologic process is vital in order to prevent progressive and irreversible neurologic injury. Several clinical conditions may predispose to the development and progression of scoliosis. Neurological signs and symptoms, such as pain, weakness, sensory changes, gait abnormalities, and bowel and bladder changes, as well as the presence of orthopaedic and cutaneous anomalies, are important indicators that a neurologic condition may exist. 5 Early age of onset, presence of a left curvature, increased kyphosis, and rapid progression are additional clinical indicators that should prompt the acquisition of a magnetic resonance image (MRI) of the spine. 6 Once a neurologic cause is identified, treatment is focused toward correcting the underlying pathology, and deformity correction is reserved for large or progressive curves following treatment. This chapter will focus on the etiology and pathogenesis, disease-specific deformity, comorbidities, techniques, and evidence-based outcomes of three specific causes of neurogenic scoliosis: Chiari I malformation, tethered cord, and split cord malformation.
13.1 Chiari I Malformation and Syringomyelia
13.1.1 Etiology and Pathogenesis
Although there are four recognized varieties of Chiari I malformations, they are vastly different pathophysiologic processes, and patients with both type I and II Chiari’s malformations may develop scoliosis. Chiari I malformations are generally defined as cerebellar tonsillar ectopia with descent of the tonsils greater than 5 mm below the foramen magnum. 7 , 8 , 9 In contrast, Chiari II malformations involve the herniation of the inferior cerebellar vermis and are associated with myelomeningocele and frequently hydrocephalus. 10 , 11 , 12 Although scoliosis is frequently encountered among patients with spina bifida and Chiari II malformations, scoliosis is unlikely to be the primary presenting sign, and, thus, this chapter will focus largely on Chiari I malformations. Among patients presenting with pediatric scoliosis, Chiari I malformations are the most common neural axis abnormality detected. 2 , 3 , 4
Marin-Padilla and Marin-Padilla suggested that a hypoplastic posterior fossa during fetal development may limit the expansion of the rhombencephalon, resulting in the herniation of the cerebellar tonsils through the foramen magnum. 13 Morphometric studies demonstrating a small posterior fossa provide further support for this theory. 9 , 14 Scoliosis may coexist with a Chiari I malformation in up to 42% of patients, 9 and an association exists between those with progressive scoliosis and the presence of a syrinx. 15 , 16 , 17 The association of syringomyelia with progressive scoliosis among patients with Chiari I malformation has been theorized to result from paresis of the axial musculature, congenital vertebral structural changes, and interference of postural tonic reflexes. 18
Several theories have been hypothesized to explain the development of syringomyelia in the setting of Chiari malformation. Gardner’s hydrodynamic theory suggests that cerebrospinal fluid (CSF) pulsations from the choroid plexus normally play a role in neural tube expansion during fetal development; unbalanced CSF pulsations between supratentorial and infratentorial compartments result in a small posterior fossa, tonsillar ectopia, and forced diversion of CSF into the central canal of the spinal cord due to obstruction of the fourth ventricular outflow tracts at the foramen magnum. 19 Williams postulated that transient intracranial pressure elevations due to Valsalva’s maneuvers resulted in bulk flow of CSF caudally; CSF outflow obstruction resists flow caudally and creates a differential pressure between the cranial and spinal compartments that contributes to worsening syringomyelia. 20 Although these theories offer insight into the etiology of syringomyelia in the setting of Chiari I malformation, they provide an incomplete explanation for the pathogenesis of syringomyelia because syringomyelia is often acquired and the syrinx does not always directly communicate with the fourth ventricle. Oldfield et al attempted to address these inconsistencies by postulating that the movement of the cerebellar tonsils during systole creates a systolic pressure wave in the spinal CSF that results in the movement of fluid into the spinal cord via perivascular and interstitial spaces rather than through the central canal at the obex. 21
13.1.2 Disease-Specific Deformity and Comorbidities
Chiari I malformations are defined by tonsillar ectopia of greater than 5 mm on magnetic resonance images (MRI). However, the degree of tonsillar descent does not always correlate with symptom severity, and Elster and Chen demonstrated that nearly 30% of patients with 5 to 10 mm of tonsillar herniation may be asymptomatic. 8 Patients with Chiari I malformations present with a myriad of symptoms and signs, but they most commonly present with occipital or cervical headache that is worsened with exertion or Valsalva. Weakness, paresthesia, numbness, nystagmus, gait imbalance, ataxia, dysphagia, dysarthria, and drop attacks are other symptoms that may result from Chiari I malformation. 5 Children may not portray typical symptoms and, instead, may present with irritability, opisthoclonus, or failure to thrive.
In addition to tonsillar ectopia, associated vertebral column or neural abnormalities are present in between 24 and 50% of patients with Chiari I malformation, and include Klippel–Feil deformity and atlantoaxial assimilation. 22 Syringomyelia occurs in between 50 and 75% of patients, is most commonly located in the cervical spine, and is associated with progressive scoliosis. 9 , 15 , 16 , 17 , 22 , 23 Overall, scoliosis is present in nearly 42% of patients. 9
13.1.3 Disorder-Specific Techniques
Treatment of Chiari I malformation–associated scoliosis is surgical and aims to relieve cervicomedullary compression at the foramen magnum in order to halt progression of scoliosis. Posterior fossa decompression is most commonly performed with a suboccipital craniectomy. There remains significant controversy regarding whether duraplasty is necessary and whether coagulation of the cerebellar tonsils should be performed in order to achieve adequate decompression. 24
13.1.4 Evidence-Based Outcomes
Arnautovic et al performed a meta-analysis and systematic review of 145 publications (8,605 patients) studying the role of decompressive surgery for Chiari I malformations. 25 The vast majority of included series involved suboccipital craniectomy with duraplasty. Neurological improvement or resolution occurred in 72%. Nearly 65% of patients harbored a spinal cord syrinx, and postoperative improvement in syringomyelia was noted in 78% of patients. Other series report that nearly 85% of patients note improvement in headache and neck pain. 22 , 26 These good outcomes, however, must be balanced by a reported median complication rate of 3.5%. Complications include pseudomeningocele, aseptic meningitis, CSF leak, meningitis, and neurologic injury. Only 11% of the series included by Arnautovic et al mentioned death as an observed complication of surgery; among these, the overall mortality rate was estimated at 3%. 25 In addition, although syringomyelia commonly improves after surgery, incomplete resolution or residual paresthesias may be present in over 50% of patients. 27
Several series have demonstrated that suboccipital decompression may halt or improve the progression of scoliosis. 15 , 16 , 28 , 29 A recent systematic review estimated that curve magnitude improved in 37% and progressed in 45% of patients with Chiari I malformations following surgical intervention. 30 Brockmeyer et al further demonstrated that curves improved or remained stable in nearly 91% of children younger than 10 years suboccipital decompression and duraplasty. 31 Other authors have linked older age, curves of greater than 40 degrees, double scoliosis patterns, kyphosis, and curve rotations with progression despite foramen magnum decompression. 17 , 31 , 32 , 33 The association of smaller curves with improvement or stabilization of curve magnitude highlights the importance of early detection and treatment in patients with Chiari I malformation and scoliosis (Fig. 13‑1, Fig. 13‑2).


13.2 Tethered Cord Syndrome and Spinal Dysraphism
13.2.1 Etiology and Pathogenesis
Tethered cord syndrome (TCS) results from a number of congenital conditions and causes progressive neurological decline due to tension on the spinal cord. The filum terminale is a fibroelastic structure that extends from the conus to the sacrum and is theorized to stabilize the conus medullaris during spinal column movement. Pathological stretch applied to the spinal cord is associated with metabolic derangements resembling those seen with ischemia. 34 These metabolic changes may be related to reduction in blood flow to the spinal cord during flexion movements, impairment of oxidative metabolism, or physical neuronal damage related to tethering. Given that the spinal canal length changes by as much as 7% during flexion and stretch applied to neural tissue results in metabolic derangements, some authors have hypothesized that the signs and symptoms of TCS are due to failure of the filum terminale to alleviate spinal cord stretch during flexion-related changes in spinal canal length. 35 Although tethering by a tight filum terminale may be the causative problem in many patients with TCS, any pathology that limits spinal cord movement, including spinal dysraphism, may result in pathologic stretch and associated signs and symptoms. Mild or moderate damage may sometimes be reversible with alleviation of the pathological spinal cord stretch via detethering procedures; however, damage due to severe stretch may be irreversible, highlighting the need for early recognition and treatment. 35 , 36
Spinal dysraphism refers to a number of congenital anomalies of the spine that result from malformation of midline dorsal neural, mesenchymal, and cutaneous ectodermal structures during early embryogenesis. 37 Development of the spinal cord occurs via neurulation, canalization of the tail bud, and regression of the caudal cell mass (CCM). The process begins at gestational day 18 when differential proliferation of the neuroectoderm is induced by the underlying notochord, resulting in folding of the flat neural plate into a neural tube (neurulation). The neuroectoderm and ectoderm then separate and form a neural tube that is covered by cutaneous ectoderm (dysjunction). This process begins in the upper cervical region and extends rostrally and caudally toward L1/L2. This process results in the formation of the cephalic portion of the spinal cord. Caudal portions, including the conus medullaris and filum terminale, develop through canalization of the tail bud and regression of the CCM. The tail bud or CCM is formed by neuroectodermal cells that are located caudal to the neural tube; vacuoles form and coalesce within the tail bud to form a central canal beginning at day 28, continuing through day 48. The central canal then connects with the rostral neural tube and forms the embryologic basis of the lower lumbar, sacral, and coccygeal spine. Lastly, regression of the caudal portion of the CCM forms the filum terminale and terminal ventricle, which eventually forms the conus medullaris. 38 Errors in neurulation, dysjunction, canalization, and regression result in congenital malformations, including myelomeningocele, meningocele, lipomyelomeningocele, dermal sinus tract, dermoid and epidermoid tumors, and fatty filum, all of which may result in tethering and caudal traction on the spinal cord (Fig. 13‑3).

TCS is often associated with syringomyelia. Thus, progression of neurological symptoms may be due to both metabolic changes within the spinal cord related to stretch and cystic dilation of the spinal cord itself. The syrinx in patients with TCS is often located in the terminal spinal cord, suggesting that the traditional pathogenic theories noted previously may not be relevant to the development of dysraphism- or TCS- associated syringes. 39 The association of TCS with scoliosis was first demonstrated by McLone et al. 40 By demonstrating stabilization or improvement in scoliosis following detethering in patients who had previously undergone myelomeningocele repair, they postulated that tethering may cause scoliosis via ischemic spinal cord injury at the site of tethering, dysfunction of sensory tracts, and asymmetric paravertebral muscle tone. 5
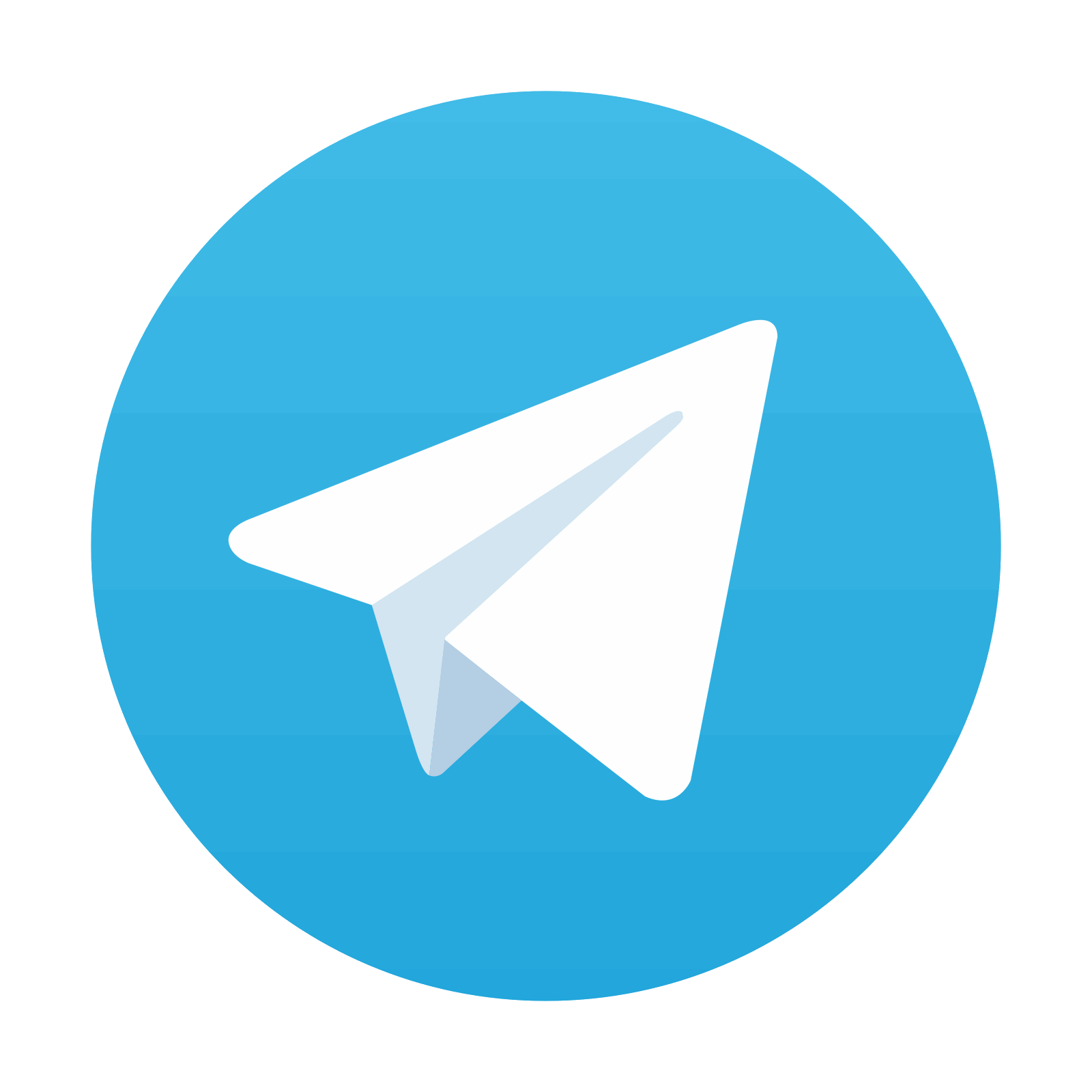
Stay updated, free articles. Join our Telegram channel

Full access? Get Clinical Tree
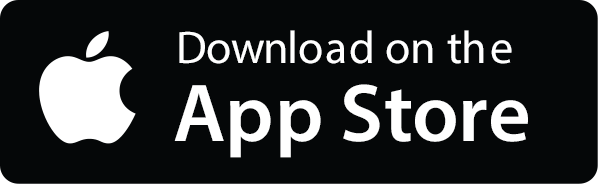
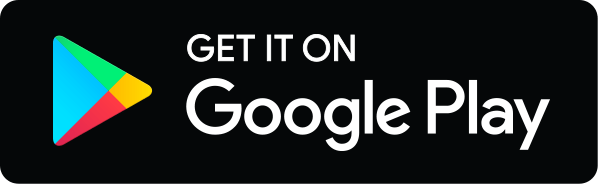
