15 Biologics
15.1 Introduction
Achieving a solid fusion is crucial to achieving stable, long-term stability following a number of spine surgery procedures. 1 , 2 Open approaches provide a wide exposure and allow for placement of large quantities of bone graft in the posterolateral gutter. Minimally invasive surgery (MIS) approaches, however, typically require interbody fusion and MIS surgeons frequently use spinal biologics to help achieve this goal. 3 Spinal biologics enhance bone formation via osteoinductive, osteoconductive, osteogenic, or a combination of the three mechanisms. 4 , 5 , 6 Osteoinductive biologics stimulate pluripotent stem cells to differentiate into an osteoblastic, bone-forming phenotype. Osteoconductive materials, by contrast, provide a scaffold that supports the growth of new bone. Osteogenesis refers to continued production of bone by preexisting bone-forming cells.
15.1.1 Biologics Classification
In most MIS applications, spinal biologics are used in conjunction with an interbody biomechanical device and placed directly in the disk space. The disk space is approached via an anterior, lateral, or posterior approach and prepared for fusion. The interbody device is then loaded with the biologic material and positioned into the disk space to help achieve fusion. There are several choices for the biologically active material that may be placed into the disk space. These can be broadly divided into two categories: bone graft (autograft and allograft) and bone graft extenders (demineralized bone matrix [DBM], bone ceramics, mesenchymal stem cells, and recombinant human bone morphogenetic protein [rhBMP]).
Historically, autograft bone has represented the “gold standard” against which other biologic agents are measured. 3 , 6 , 7 Autograft bone may consist of cancellous or corticocancellous bone. In MIS applications, autograft may be obtained from one of the following three sources: iliac crest, 8 bone shavings from an oscillating burr, 9 , 10 and local bone (laminectomy/facetectomy bone). Autograft bone is considered desirable because it is the only graft choice that is widely accepted to be osteoinductive, osteoconductive, and osteogenic. Allograft bone, in contrast, is osteoconductive and may have some weak osteoinductive effects. 6 For this reason, allograft bone is typically used in conjunction with autograft bone or other osteoinductive agents (e.g., rhBMP). The benefits of allograft bone include the ability to place a larger quantity of bone graft and avoiding any potential donor site morbidity associated with iliac crest bone graft. 11 , 12 Corticocancellous allograft provides mechanical support in addition to osteoconduction. For this reason, corticocancellous allograft may be placed directly into the interbody space without a cage. This type of allograft is most commonly used in the cervical spine. 13 , 14
Bone graft extenders vary in their mechanism of action and efficacy. Major categories of bone graft extenders include DBM, bone ceramics, and rhBMP. DBM is created following chemical extraction of the mineralized portion of cadaveric human bone. 6 This process retains an osteoconductive scaffold for bone growth and also preserves bone proteins such as BMP to provide an osteoinductive effect. Bone ceramics are calcium-based substitutes that provide an osteoconductive scaffold for bone growth. 6 , 15 , 16 These graft materials are typically a mix of hydroxyapatite (HA) and tricalcium phosphate (TCP) that undergo gradual remodeling during bone formation. 17 rhBMP is a widely used adjunct to assist with spinal fusion. 7 BMPs are proteins within the transforming growth factor-beta (TGF-β) superfamily and induce bone formation by inducing the differentiation of pluripotent mesenchymal stem cells toward osteogenic and chondrogenic phenotypes and by stimulating angiogenesis and alkaline phosphatase activity. 17 RhBMP is widely utilized in MIS surgeries via the anterior, lateral, or posterior approaches to assist with interbody fusion. 3 , 18
15.1.2 Outcomes
Although several authors have examined the use of biologics in open spine surgery, there is less evidence of its use in MIS. The use of autograft in MIS varies by approach, but it is commonly collected and utilized in posterior MIS approaches to the lumbar spine and anterior approaches to the cervical spine. Kasliwal and Deutsch described their results in a series of 40 patients undergoing MIS transforaminal lumbar interbody fusion (TLIF) using a cage filled with local bone shavings. 9 The authors reported a fusion rate of 70% with good clinical outcomes in 92% of patients undergoing a MIS TLIF at minimum 1-year follow-up. 9 In a recently published meta-analysis, Parajón et al reported a 91.8% fusion rate for patients undergoing MIS TLIF with autograft bone only. 3 In these studies, autograft bone typically refers to locally harvested bone (e.g., bone shavings or osteotome cuts). Lopez et al, however, recently described a MIS approach to harvest iliac crest bone. 8 While the early results with this technique are promising, long-term fusion data have not yet been reported.
The use of allograft bone and bone graft extenders is also quite common in the MIS approach. In their meta-analysis, Parajón et al reviewed results from 40 series that spanned 1,533 patients seeking to determine the optimal graft material for MIS TLIF. 3 The authors demonstrated that MIS TLIF resulted in high fusion rates regardless of graft material (91.8–99%), but that the fusion rates generally increased when rhBMP was utilized (96.6 vs. 92.5%). The lowest fusion rates were observed when autologous local bone was utilized without rhBMP or bone graft extenders (91.8%). The addition of rhBMP to local bone increased the fusion rate to 93.1%. Using autograft bone, rhBMP and an additional bone graft extender resulted in fusion rates of 99.1%. It should be noted, however, that this meta-analysis was limited by the substantial heterogeneity in surgical technique, follow-up, and assessment of fusion. 3 Finally, these authors were also unable to determine the clinical significance of fusion in patients undergoing MIS TLIF. 3
The use of biologic agents is more well established in the anterior lumbar spine, as this was the original indication for which rhBMP was approved. 7 It is generally accepted that in the anterior lumbar spine, the use of rhBMP can result in fusion rates that are noninferior (and potentially superior) to autograft bone. 19 , 20 Galimberti et al reviewed 16 studies with an rhBMP and control arm to determine the impact of rhBMP on fusion rates in the lumbar spine. The authors stratified their results by approach (anterior lumbar interbody fusion [ALIF], posterior lumbar interbody fusion [PLIF]/TLIF, and posterolateral fusion [PLF]). For ALIF, the authors reported an increase in the rate of fusion in three of four studies (odds ratio: 7.08, 95% confidence interval: 1.5–32.7). 20 The authors determined that there was no increased rate of fusion associated with rhBMP use and PLIF/TLIF.
15.1.3 Complications
The use of rhBMP may be associated with several important and clinically relevant complications, including prevertebral swelling in the cervical spine, soft-tissue swelling, local inflammation, cyst formation, osteolysis, implant migration/subsidence, retrograde ejaculation, and ectopic bone formation. 21 , 22 , 23 , 24 , 25 , 26 , 27 , 28 Ectopic bone formation is an important consideration with the use of BMP in PLIF and TLIF procedures. 29 Although this bone formation is asymptomatic in a majority of cases, 29 it can lead to neuroforaminal impingement and symptoms severe enough to require reoperation. 28
Singh et al reviewed 610 consecutive patients undergoing an MIS TLIF with BMP and identified a 1.7% rate of reoperation (10/610) in this cohort due to BMP-related complications, including neuroforaminal bone growth, vertebral body osteolysis, and cage migration. 28 All 10 of the patients undergoing reoperation had neuroforaminal stenosis and 2 of the same 10 patients had osteolysis and cage migration. Singh et al also performed a systematic review to examine the complication rates of rhBMP and demonstrated that the rates of complications were highly spine site specific (0.6–20.1% in anterior cervical diskectomy and fusion, 3.5–14.6% in posterior cervical, 2.0–7.3% in ALIFs, and 1.5–21.8% in PLIF/TLIF, and 1.4–8.2% in PLFs). In their analysis, the authors identified that the only individual complication that increased with rhBMP use was retrograde ejaculation. 30 , 31 It is important to note, however, that the dosing of rhBMP varies widely by institution and surgeon and the complications associated with BMP may be dose dependent. 18
15.2 Bone Ceramics
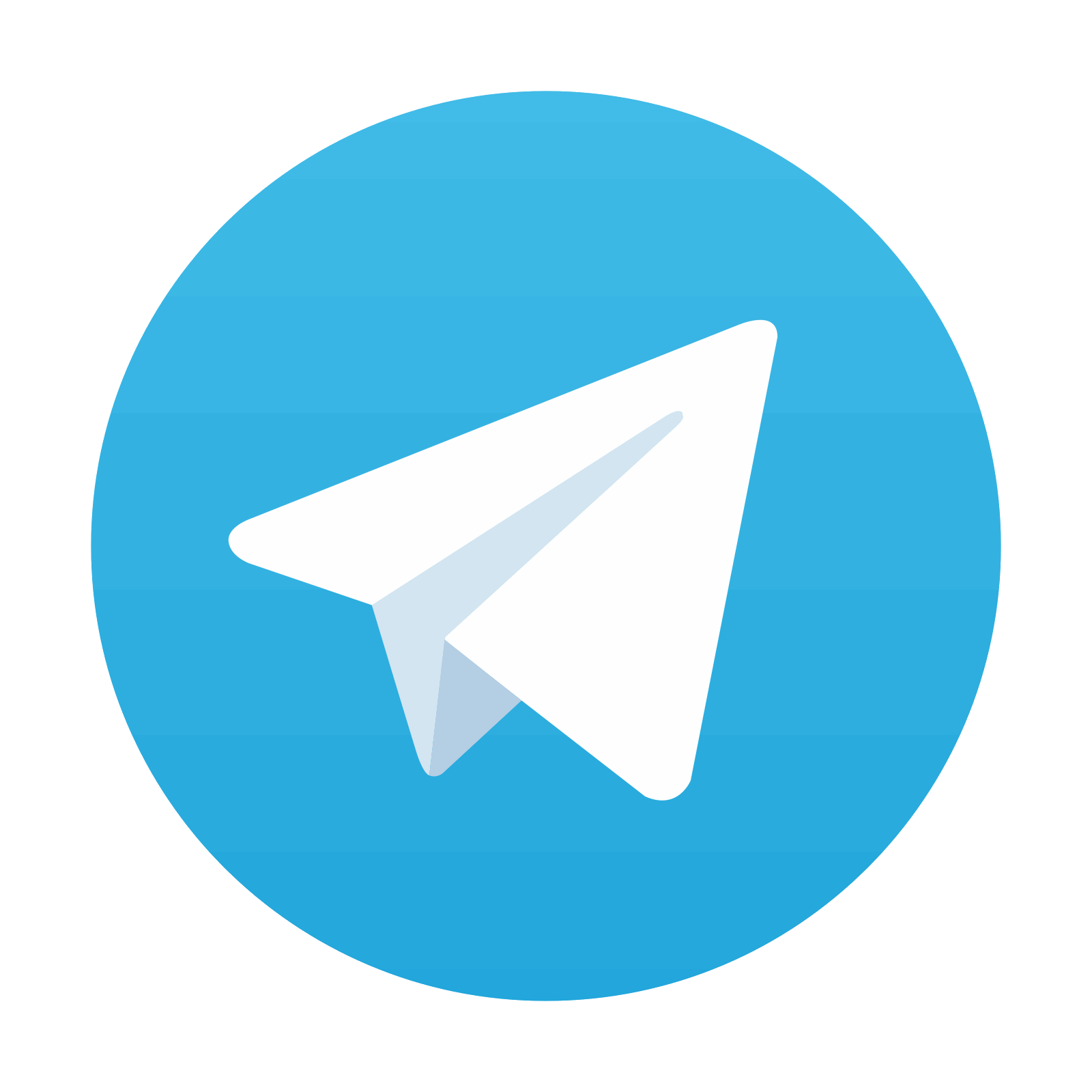
Stay updated, free articles. Join our Telegram channel

Full access? Get Clinical Tree
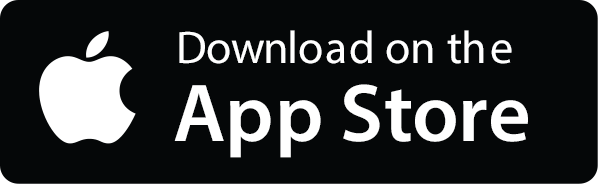
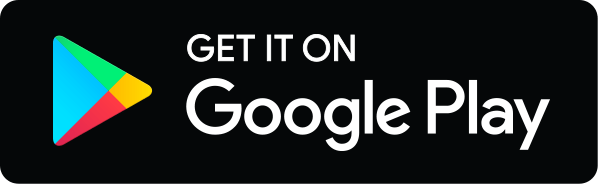