15 Implantation of Strip, Grid, and Depth Electrodes for Invasive Electrophysiological Monitoring
The main goal of resective surgical interventions in the management of medically refractory epilepsy is the complete removal of the epileptogenic zone while preserving adjacent functionally critical cortical areas. Therefore, the precise localization of the epileptogenic zone in epilepsy surgery has critical significance for achieving postoperative seizure-free outcome.1–3 This is especially true for the patients with extratemporal epilepsy and radiologically occult abnormalities. Although scalp electroencephalography (EEG) is very helpful in determining the location of the epileptogenic zone, it does not delineate the surgical target precisely in magnetic resonance imaging (MRI) negative cases and in many extratemporal epilepsy cases. The main contribution and value of extraoperative monitoring with intracranial electrodes in epilepsy surgery is its role in the precise determination and mapping of the epileptogenic zone and eloquent cortex. Invasive monitoring is required more frequently in children than adults because of the high frequency of extratemporal epilepsy and dysplastic cortical lesions in the pediatric age group. In these cases, preoperative data obtained with non-invasive monitoring techniques may be inconclusive with multiple foci or even unclear lateralization. Invasive monitoring is frequently required in these patients and constitutes 25 to 40% of cases in some pediatric series.2,4–6
Indication
Invasive monitoring is indicated if preoperative assessment with noninvasive tests
is inconclusive with unclear lateralization or localization of epileptogenic zone;
provide noncongruent data; or
reveal the following:
multiple epileptiform areas,
multiple structural lesions with ill-defined electrographic focus,
no clearly defined structural lesion on MRI despite electrographic evidence of epileptogenic zone in a certain cortical region,
electrographic evidence implying a larger epileptogenic zone than lesional zone on MRI, or
epileptogenic zone adjacent to eloquent cortex.
However invasive monitoring is not an exploratory procedure and is helpful only if the noninvasive workup is suggestive for certain cortical locations. In these cases, further delineation and accurate localization of the epileptogenic zone is required to be able to offer surgical resection as a management option to the patient.2–9
Although other indications can be defined, the most common indications for invasive monitoring in temporal lobe epilepsy (TLE) include the following:
Presence of noninvasive electrographic data showing bilateral temporal seizure onset
Semiological and electrographic difficulty in differentiating temporal lobe seizures from possible frontal lobe seizures
Presence of electrographic data suggestive for unilateral temporal onset in presence of bilateral imaging abnormalities
Ill-defined electrographic abnormalities with a unilateral structural abnormality on imaging studies
Sperling defined some major and minor criteria for the role of invasive monitoring in TLE. He did not recommend invasive monitoring if the patient has concordant data fully or with either two major plus one minor or one major plus three minor criteria listed in Table 15.1 . If tests are discordant or there are an insufficient number of concordant test results, he recommends invasive monitoring with intracranial electrodes.6
In extratemporal epilepsy, invasive monitoring is indicated not only to define the epileptogenic zone but also to map the eloquent cortex. Even in cases with an extratemporal lesion with well-defined borders, if it is adjacent to eloquent cortex, invasive monitoring with grid placement may still be needed for stimulation and mapping purposes. This is also true for cortical dysplasia cases that often have ill-defined borders and more extensive electrographically abnormal areas than imaging studies suggest.2,3,5,6
Invasive Monitoring Techniques
Several invasive monitoring electrodes may be used in epilepsy patients. The most commonly used electrodes are subdural strip, grid, and depth electrodes ( Fig. 15.1 ). Epidural peg electrodes are also available but not as widely used. Invasive monitoring electrodes can be chosen among commercially available electrodes or can be custom made based on the specific needs of the case. These can be used separately or in combination to determine the epileptogenic zone. The extent of the coverage, ideal electrode type, and configuration should be determined by the epileptologist, epilepsy surgeon, and neurophysiologist together after review of the available data for each patient. The initial step in invasive monitoring is determining the cortical area with the highest likelihood of epileptogenicity to cover with invasive electrodes. Scalp EEG data and MRI studies provide the most valuable data in defining the cortical area that needs to be covered.5,6 Invasive electrodes are frequently placed bilaterally, but if lateralization of the seizures is clear and localization is questionable, coverage may be asymmetrical with more extensive sampling from the suspicious hemi-sphere.6 Each electrode and invasive monitoring modality has certain advantages and disadvantages. Although depth and strip electrodes are more useful to lateralize the seizure onset, grids are much more helpful to further localize the epileptogenic zone.

Depth Electrodes
Depth electrodes are most valuable in assessing deep cortical structures such as the amygdala, hippocampus, para-hippocampus, cingulum, and orbitofrontal cortex.10 Depth electrodes in pediatric epilepsy surgery are mostly used in older children, although still not as commonly as in adults. These are multicontact electrode arrays, consisting of up to 12 nickel-chromium or platinum contacts embedded in a thin, tubular, biologically inert Silastic material. They can be placed through a drill or burr hole using a stereotactic frame or frameless neuronavigation guidance. Typical indications for depth electrodes include bilateral mesial temporal epilepsy (MTS), dual pathology, differentiation between mesial and neocortical temporal epilepsy or temporal lobe and or-bitofrontal seizure onset, and presence of epileptogenic lesion located in deep brain parenchyma, such as in cases of hypothalamic hamartoma or periventricular heterotopia. Depth electrodes are also useful in clarifying conflicting data such as the presence of unilateral MTS on MRI and bilateral independent or nonlocalizing interictal/ictal epileptogenic activities in noninvasive EEG monitoring. Although depth electrodes provide excellent data from deep structures, they do not have cortical surface coverage and have very limited stimulation capabilities. Therefore, depth electrodes are frequently used in combination with strip electrodes.4 Bilateral hippocampal depth electrodes combined with bilateral temporal subdural strip electrodes provide perfect coverage for both neocortical and mesial temporal areas bilaterally. This already large coverage can be easily further extended to both orbitofrontal regions by adding two more subdural strip electrodes. Depth electrodes can also be placed in the cingulum and orbitofrontal cortex; for additional frontal coverage, they can be combined with strip electrodes, such as bilateral orbitofrontal depth electrodes with bilateral convexity, lateral frontal, and interhemispheric strip electrodes.4,7,10
Depth electrodes can be placed into the mesial temporal structures through two different approaches: occipitotemporal and temporal ( Fig. 15.2A–F ). The occipitotemporal approach provides a trajectory parallel to the long axis of hippocampus, whereas the temporal approach provides a trajectory orthogonal to hippocampus. We prefer the occipitotemporal approach because it has the advantage of placing multiple contacts throughout the amygdala and hippocampal head and body with a single-depth electrode. Because this approach would not provide any information regarding neocortical electrical activity, additional subdural strips can be easily placed to cover temporal neocortex at the same time. Conversely, the temporal orthogonal approach has an advantage of providing data both from mesial as well as neocortical structures through a single-depth electrode. However, this approach often necessitates multiple-depth electrodes (two or three) on each side and results in only one or two contacts truly in the mesial structures whereas the other contacts are in neocortex. However, this approach provides very limited neocortical electrographic data compared with strip electrodes.

Subdural Strip and Grid Electrodes
Both subdural strip and grid electrodes are thin, biologically inert, Silastic or Teflon sheets with embedded nickel-chromium or platinum, electrically isolated electrode contacts. Each electrode contact is 2 to 4 mm in diameter, and generally the interelectrode distance is 10 mm. Subdural strips are a single row of contact electrodes with 10 mm interelectrode distances (1 x 4, 1 x 6, 1 x 8). Subdural grids are larger plates of rectangular arrays with several parallel rows of up to 64 electrodes (2 x 4, 2 x 6, 4 x 8, 8 x 8 …). Both subdural strips and grid electrodes are very thin and flexible sheets and can be contoured to the underlying cortical surface. They are also transparent and thus allow visualization of the underlying anatomical structures. There are many commercially available strip and grids with various configurations and shapes, such as curvilinear, dual-faced grid electrodes for interhemispheric coverage. Custom-made options are also readily available. Strip electrodes are placed through burr holes or can be slid under craniotomy edges if they will be used in combination with grid electrodes ( Fig. 15.3 ). Multiple subdural strip electrodes using different trajectories to sample large cortical areas can be placed through a single burr hole. Strip electrodes are ideal to lateralize and grossly localize the most suspicious cortical areas for seizure onset. This area would need further exploration by grid electrodes for more precise mapping to define the surgical resection area. Grid electrode placement is performed with a large craniotomy ( Fig. 15.4A,B ). Grid electrode recording is an ideal technique to locate the epileptogenic zone and to stimulate and map the adjacent eloquent cortex by covering large cortical surfaces more completely. Subdural grid electrodes are frequently used in pediatric epilepsy surgery to cover large extratemporal cortical areas.4–7,11


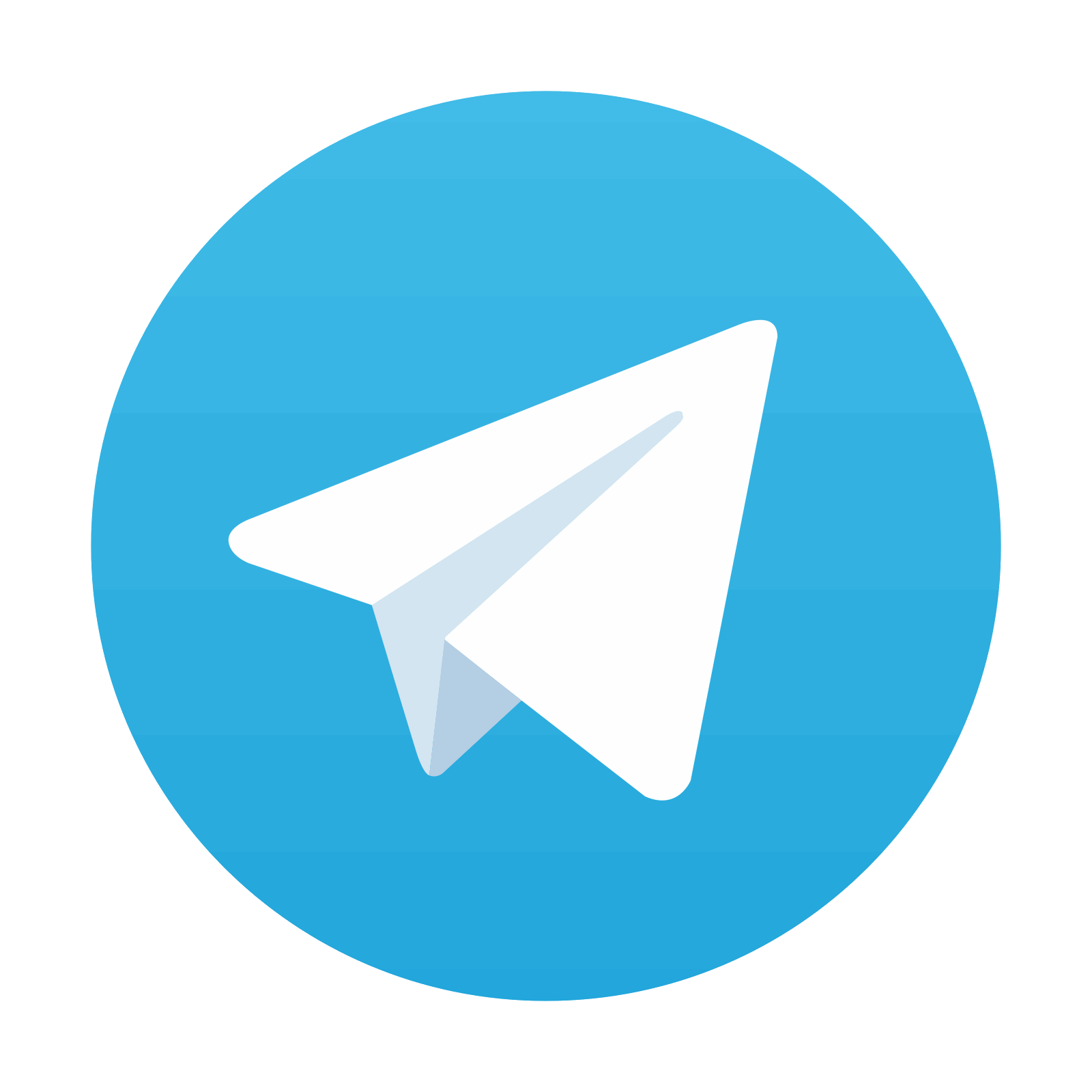
Stay updated, free articles. Join our Telegram channel

Full access? Get Clinical Tree
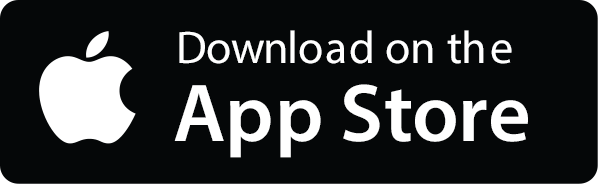
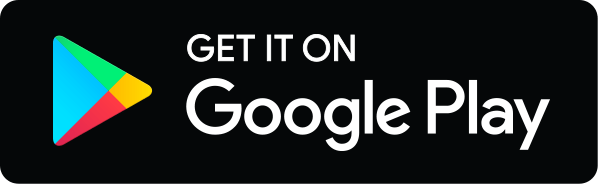
