15 Multiple Subpial Transections
Abstract
The surgical treatment of epilepsy can be divided into resective and nonresective treatment approaches with the nonresective strategies focused on neuromodulation and disconnective procedures to inhibit the propagation of seizures. Multiple subpial transection is a nonresective, disconnective procedure that targets what we know about cortical circuitry and uses it to inhibit the propagation of seizures in eloquent cortex when resective surgical strategies are not an option.
15.1 Introduction
Epileptic seizures may be classified on the basis of their underlying etiology, clinical semiology, the anatomical region, or network involved, or by an associated syndrome. A commission of 120 European members of the International League Against Epilepsy (ILAE) chaired by the French neurologist Henri Gastaut attempted the first modern classification of seizure types in 1964. 1 Recognizing the contemporary limitations of the field, the commission warned that its scheme “must of necessity be a tentative one” and “subject to change with every advance in scientific understanding of epilepsy.” 1 In this tradition, the ILAE in 1981 reimagined the classification of seizures after reviewing a large cohort of video electroencephalographic (EEG) data. 2 This system, in turn, is the direct progenitor of the most recent 2017 revised operational classification of seizure types. 3 Fundamentally, seizures are categorized based on their onset: focal, generalized, or unknown. Within the focal onset type, further descriptors may be applied in a methodical way including “with impaired awareness,” “motor onset,” and “focal to bilateral tonic–clonic.”
Within the context of the ILAE framework for seizure classification, focal onset seizures occupy the historical center of epilepsy surgery based on the straightforward proposition that a focal semiology is the clinical manifestation of a discrete anatomical substrate underlying ictogenesis—and that such an anatomical site might be excised with the goal of seizure freedom. This mode of approaching epilepsy surgery is complicated by several facts. First, any individual ictal event is composed of seizure onset, propagation, and termination, which are all both physiologically distinct processes and likewise distinct opportunities for therapeutic intervention. Second, electrophysiological and imaging studies may provide data discordant with the clinical semiology leading to uncertainty about the seizure onset zone. Third, the epileptogenic brain tissue may not be amenable to surgical excision, or an attempt at such a resection may result in substantial neurological deficits. Additionally, focal onset seizures may in fact be multifocal and contain several seizure onset zones. Another, and by no means last, consideration is that primary generalized seizures or multifocal seizures may also benefit from surgical intervention aimed at limiting the clinical scope of the disease for the patient.
While taking into consideration the sometimes complex nature of seizure genesis and propagation can problematize the role of the epilepsy surgeon, it can equally invite creative solutions. In our own time, neuromodulation is an area of active investigation, for example. However, chief among these solutions, historically, are the so-called disconnection procedures aimed at limiting the propagation of seizures or, in some cases, at disrupting the neural network responsible for seizure generation. Multiple subpial transection (MST) is a nonresective surgical technique in which small regions of neocortex are anatomically disconnected from immediately adjacent areas within a gyrus in order to prevent ictal genesis and propagation across cortical topology.
15.2 From Bench to Operating Theater
Frank Morrell and colleagues at Rush Medical College conceived of MSTs within the context of a bourgeoning scientific understanding of neocortical anatomy and physiology. Two centuries prior to Morrell’s breakthrough work, Francesco Gennari published a first glimpse of cortical laminar organization, observing a prominent white band running parallel to the pial surface within the calcarine cortical gray matter. 4 Over the next century, advances in microscopy and histology allowed the founders of modern neuroanatomy including Gustaf Retzius and Santiago Ramón y Cajal to further characterize the laminar structure of the cerebral cortex. This pursuit culminated with the work of Korbinian von Brodmann who exquisitely detailed the architectonics of whole human pallium and defined the lamination of the isocortex as six distinct layers. 5
An understanding of the laminar structure of the cortex necessarily points to a vertical interconnectivity among the laminae: for example, prominent apical dendrites of layer III and V pyramidal cells notably ascend across multiple cortical layers seeking their synaptic input. In the 1950s Vernon Mountcastle in his studies of the feline somatosensory cortex demonstrated a functional similarity with respect to input across all radially oriented layers underlying any given point on the cortical surface. 6 He hypothesized that the primary somatosensory cortex was divided into narrow cylinders or columns each representing a distinct receptive field, and he demonstrated a similar finding in the nonhuman primate cortex. 7 Over the course of his career, Mountcastle developed a theory by which the entire neocortex was divided into discrete modular columns each containing a similar microcircuitry within itself and each serving as a functional and even quantal unit of information processing. 8 , 9 Concomitantly to Mountcastle, David Hubel and Torsten Wiesel were performing pioneering studies characterizing the mammalian visual system. They similarly found a vertical orientation of any given receptive field in the primary visual cortex. 10 , 11 , 12 Most significantly, they discovered a functional significance to the columnar organization of the primary visual cortex since both orientation-selectivity 13 , 14 and ocular dominance 15 , 16 were segregated into discrete vertical units. Roger Sperry, who shared the 1981 Nobel Prize with Hubel and Wiesel, performed a series of powerful lesioning experiments in multiple regions of the mammalian cortex supporting its organization into functionally discrete columnar units and emphasizing the importance of radial intracortical connections over tangential ones. In one experiment, knife cuts made perpendicular to the cortical surface through the gray matter of the primate sensorimotor cortex did not produce any apparent deficit in motor function or coordination. 17 In a similar set of experiments, tantalum wire and mica plates were inserted into the feline and macaque visual cortex in order to segregate it into discrete vertical columns, and indeed, no difference in shape or pattern perception could be detected at a behavioral level. 18 , 19
Frank Morrell began his own experiments among the emerging understanding of cortical columns as described earlier, directly inspired by the lesioning work of Roger Sperry. Additionally, he determined the appropriate interval of his subpial transections based on electrophysiological experiments performed by Hans Lueders and colleagues in a pharmacological seizure model. The generation of independently stable epileptiform spike activity, thought to arise from the synchronous activity of a large population of cortical neurons, required a minimal area of distribution of approximately 12.5 mm2 empirically. Similarly, two ictal spikes separated by more than 6 mm were nearly always independently generated, but those separated by less than 4 mm were dependent upon each other and discharged simultaneously. 20 , 21 , 22 Taken together, these data suggested that a minimal columnar volume of cortex is required to generate enough synchrony to spawn ictal activity. Thus, Morrell recommended that transections should be performed at 5-mm intervals across a gyrus. It should be noted, however, that a very large body of in vitro data demonstrated that seizure-like activity can be generated in acute brain slices only several hundreds of micrometers thick and a couple of millimeters wide. Both the significance of and the physiological basis for this discrepancy are unclear. Nonetheless, one potential mechanism of MSTs may be to parcel the cortex into small columns incapable of sustaining enough cortical synchrony to generate a clinically significant ictal event.
An alternative or additive mechanism of MST may be to prevent the lateral propagation of a seizure through the cortex at a site distant from the seizure onset zone. Epileptiform activity propagates through multiple channels including deep corticos-triatal and corticothalamic networks, cortico-cortical association and commissural fibers, and direct lateral cortical spread. The last modality of seizure propagation might be inferred from the earliest descriptions of the “Jacksonian march,” whereby in a partial seizure the sensory or motor symptom begins in a restricted area and spreads to include more of the body in a way that is determined by cortical somatotopy. Early EEG and electrocorticography correlated physiological epileptiform spread across the cortex with this semiology. 23 More recent in vitro data suggest specificity among cortical laminae for mediating the horizontal spread of seizure activity within the cortex. In an acute brain slice pharmacological model of seizure activity, vertical cuts in the cortex were made so as to isolate laminar bridges through which an epileptiform wave must pass. Indeed, cortical layer V containing large pyramidal cells was both preferred and sufficient for supporting the lateral spread of an ictal wave through the cortex, whereas an isolated cortical bridge consisting of layers II to III required very high doses of proconvulsant drug to sustain an epileptiform discharge. 24 In the same experiment, focal application of an antiepileptic drug was most effective at aborting the propagating seizure-like wave when applied to layer V. A subset of intrinsically bursting deep layer V pyramidal cells are known to integrate lateral synaptic inputs across many laminae, 25 underlie cortical oscillatory activity, 26 and the so-called upstates, 27 and to be able to be synchronized with each other as a population via a local inhibitory circuit. 28 One may hypothesize that MSTs may disrupt one key modality of lateral propagation of seizures through the cortex involving deep pyramidal cells. One small study, however, failed to identify such intrinsically bursting neurons in surgically excised human epileptogenic cortex. 29
15.3 Surgical Technique
In the technique originally described by Morrell et al, 30 a punctate opening in the pia-arachnoid of the concerned gyrus was made with a no. 11 scalpel blade at the lateral aspect of the crown of the gyrus near the sulcus. A specialized instrument was devised which consisted of a heavy steel wire terminating in an obtuse-angled hook. Right-angled or acute-angled hooks were thought to risk snagging arachnoid or vessels. The terminus of the instrument was designed to be rounded and blunt so that it might ride along the pia arachnoid without violating it during the creation of the subpial vertical incision in the cortex. The length of the angled portion of the hook was 4 mm, approximately the thickness of the cortical gray matter to avoid damage to underlying U-fibers, projection fibers, and vasculature. The instrument was inserted vertically at the lateral border of the gyral crown deep along the sulcus, and then with the hook remaining in a vertical orientation the instrument was swept in an arc-like fashion directed toward the opposing sulcus. The tip of the hook was opposed to the overlying pia and then dragged along the pia-arachnoid to its entry point. In this way, a vertically oriented cut in the cortical gray matter was made perpendicular to the long axis of the gyrus. This process was repeated parallel to the first transection every 5 mm along the gyrus. Hemostasis was achieved with thrombin-soaked gel foam.
We perform the technique with some modifications from the original description. We choose as our entry point the middle of the crown of the gyrus, distant from the vascular sulcal border. The pia is coagulated at the entry point with irrigating bipolar electrocautery, briefly and at low amplitude. The pia is then opened with a 25-gauge needle at a single point to allow an avenue of insertion for the transector. Other surgeons have reported the use of micropoint diathermy to create the pial opening. In our technique, the transector is inserted twice into the same entry point and swept toward opposite sulci, in turn. We prefer a microsurgical ball probe terminating in a 4- to 5-mm hook bent at approximately 100 degrees. The tip is, of course, blunt to avoid perforating the pia or violating any sulcus or vessels. We similarly perform transections every 5 mm along the gyrus, perpendicular to its long axis. We avoid coagulation with electrocautery as much as is possible in eloquent areas of cortex—thrombin-soaked gel foam applied over the entry point is sufficient in most cases. In ▶Fig. 15.1 (a–c), intraoperative photographs display the stages as electrode placement, seizure mapping, and resection and adjacent MSTs. In ▶Fig. 15.2 (a, b), the postoperative magnetic resonance images (MRIs) (sagittal and coronal slices) display the actual anatomy of the transections.


We use intraoperative electrophysiology in all cases, similar to Morrell’s original description. In the vast majority of cases, abnormal electrocorticographic discharges cease after MST. If epileptiform waveforms persist, then the transections should be investigated to ensure that they are completely through the depth of the gray matter and include gray matter in the sulcus. Frequently, MST is used in combination with an excisional procedure if both eloquent and noneloquent cortexes are involved in the epileptogenic zone. We recommend the excisional procedure be performed prior to the MSTs. If an excisional procedure is not performed, a small cortical biopsy for pathology should be considered if safe.
In Morrell’s original series, all cases were performed awake. Given sufficient preoperative invasive mapping of seizure activity and of eloquent cortex in an epilepsy monitoring unit, coupled with intraoperative somatosensory-evoked potentials and motor responses induced by direct cortical stimulation without neuromuscular blockade, it may be safe to perform the procedure under general anesthesia for rolandic cases. For cases involving critical language cortex, an awake craniotomy is preferred, especially if MST is coupled with a resective procedure.
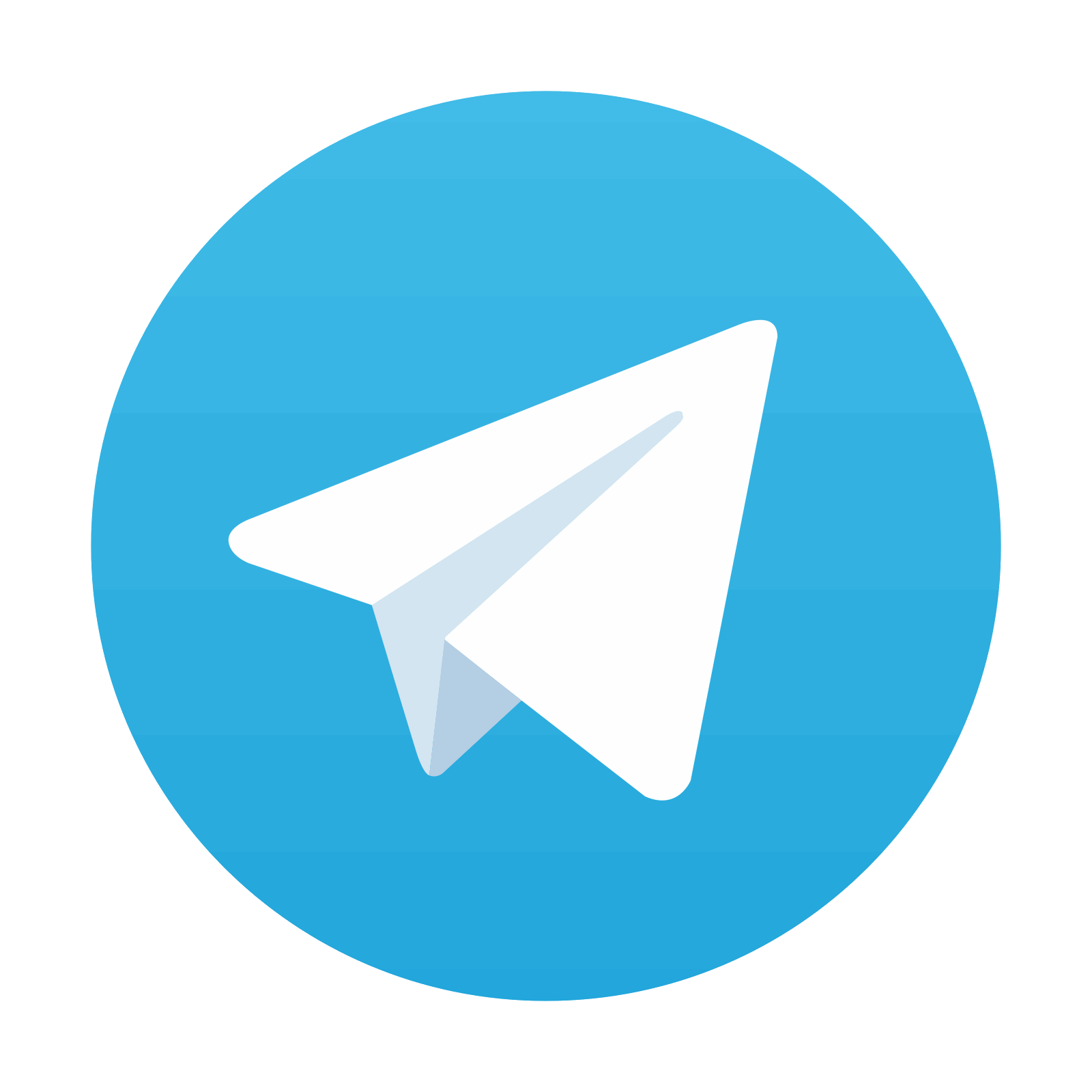
Stay updated, free articles. Join our Telegram channel

Full access? Get Clinical Tree
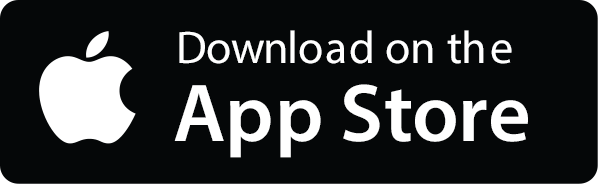
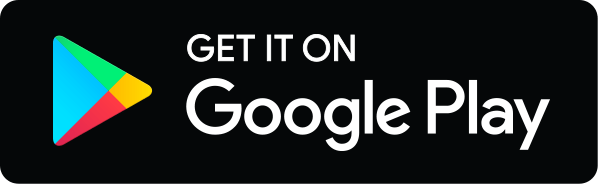