16 Gamma Knife Radiosurgery for Treatment of Sporadic Vestibular Schwannoma
16.1 Introduction
Gamma Knife radiosurgery (GKRS) was developed by Lars Leksell, a Swedish neurosurgeon, in the 1950s as reviewed in Chapter 2.s. Literatur Over the past more than 60 years, and especially in the past 30 years, GKRS has become a well-established, safe, and effective treatment for vestibular schwannomas (VSs) worldwide. According to the international treatment registry kept by the Leksell Gamma Knife Society, a total of 100,609 VSs were treated with GKRS between 1968, when the registry began, and 2016, the most recent year with complete data.s. Literatur There is a clear worldwide trend over the past 15 years for an increasing number of VS patients per year being treated with GKRS (Fig. 16‑1 ). By 1995, a total of 4,810 patients, by 2005, 32,610 patients, and by 2016 more than 100,000 VS patients had been treated with GKRS. In 2016 alone, there were 7,709 VSs treated worldwide with GKRS, 1,160 of which were treated in North America. There are currently 116 Gamma Knife centers in the United States, and as of February 1, 2017, a total of 325 gamma units have been installed worldwide.

The general principle of GKRS involves application of a stereotactic head frame, which allows for rigid head fixation during treatment, and also allows for the establishment of a coordinate system. Next, a fiducial system attaches to the head frame during imaging, allowing a computer dose-planning system to reference the fiducials with the desired images, creating a stereotactic space. The dose-planning system then calculates summated isodose curves of individual “shots of radiation” taking into account the cumulative effect of isocenter placement, collimator size, weighting, and head angulation to create a highly conformal prescription isodose to cover the tumor.
We installed our first gamma unit (Model U) at the Mayo Clinic in 1990 and then subsequently upgraded to the Model B, Model C, 4C, and since 2007 have used the model Perfexion (Fig. 16‑2 ). The previous units used 201 Co60 radiation sources arranged more or less concentrically to provide an ovoid isocenter of radiation. The radiation beams could be collimated to 4, 8, 14, or 18 mm. Some of the 201 channels could be blocked in order to protect radiosensitive structures such as the lens of the eye, or to slightly “shape” the isocenter. Admittedly, the process of blocking more than a few beams was very labor intensive, and rarely employed. Thus, multiple differentially weighted isocenters were each assigned a unique x (right/left), y (anterior/posterior), and z (superior/inferior) coordinates in the stereotactic space. When the radiation contribution of each isocenter is summed, this then results in a very conformal radiation plan that highly matches the shape of the VS. The Perfexion unit, instead, uses 192 Co60 sources also arranged concentrically. The isocenters are further subdivided into eight sections of 24 sources. Each section can be automatically collimated to 4, 8, or 16 mm, or completely blocked. Thus, with this latest version of the gamma unit, there are thousands of different possible isocenters one could create. This allows for more flexibility when shaping each individual isocenter, which at times proves helpful to avoid radiation to critical structures. Also, a very conformal plan can be created with fewer individual isocenters or “shots,” making treatment more efficient.

The purpose of this chapter is to review the technique and results of GKRS for VS, using contemporary techniques and dose. Primary endpoints of interest include tumor control, facial nerve, hearing and other cranial nerve morbidity, and other treatment-related side effects.
16.2 Technique
16.2.1 Patient Consultation
At our institution, all patients with sporadic VS are initially evaluated by a neurosurgeon and a neurotologist, and counseled regarding the options available for treatment of their tumor. Currently, we offer GKRS as a treatment option to all patients with tumors that have a maximum posterior fossa diameter of less than 2.5 cm. We have occasionally treated larger tumors and it is highly dependent on patient comorbidities, history of prior treatment, and preferences.s. Literatur Patients who select GKRS are then also seen by a radiation oncologist the day prior to undertaking treatment, and the goals, risks, benefits, and alternatives of VS GKRS are reviewed yet again. An updated audiogram including pure-tone average (PTA) and speech discrimination scores (SDS) is obtained. Patients are instructed not to eat or drink anything after midnight the evening before the procedure. They are allowed to take any daily medications with a sip of water the morning of treatment and then are instructed to report to the hospital the morning of the outpatient treatment of their tumor. Patients are given 1 mg of oral midazolam for mild sedation and then transported to the gamma unit.
16.2.2 Head Frame Application
With the patient in the seated position, the head and hair are cleaned with 70% isopropyl alcohol and the Leksell model G stereotactic head frame is applied using local anesthesia (2:1 mixture of 1% lidocaine with 1:100,000 epinephrine and 0.25% Marcaine including 10% NaHCO3) and four-point pin fixation. The head frame is positioned slightly eccentric to the side of the tumor with the ear bars resting in the ear canals. It is optimal to keep the base ring of the head frame as low as possible to minimize distortion artifact on the planning imaging. Five to 6 milliliters of local anesthetic are injected at the expected location of each of the pins, and after allowing ample time for the sites to become appropriately anesthetized, the pins are inserted through the skin to the outer table of the skull. We simultaneously tighten opposing pins (right anterior and left posterior; left anterior and right posterior) using hand tools only. We prefer that these be tightened to finger pressure tightness only. It is obviously critically important that the head frame does not move during imaging or treatment, but if the pins are over torqued it can bow the upright supports that can then warp the base ring and potentially alter how the fiducial boxes sit on the frame during imaging. This can introduce additional error into the stereotactic accuracy of the imaging. Most patients find the head frame application mildly uncomfortable and are left with a sense of head pressure for several minutes to an hour after application that then becomes minimally bothersome.
16.2.3 Imaging
The patient is then transported to the magnetic resonance imaging (MRI) suite where they undergo postgadolinium axial imaging with a standard fiducial box in place. The protocols we use to scan our patients are listed in Table 16‑1 . One-millimeter-thick axial postgadolinium images are used for planning. Sagittal and coronal reconstructions are performed by the Gamma Plan software (Elekta AB, Stockholm, Sweden) to also aid in dose planning. These sequences usually result in a mean stereotactic error of 0.1 to 0.3 mm and a maximum error of 0.3 to 0.7 mm. We will accept a mean error up to 0.3 mm, but we will rescan or repeat imaging on a different MR scanner for anything greater, until satisfied. A thin-slice noncontrast stereotactic computed tomographic (CT) scan of the temporal bones is then obtained to better define the bony margins of the internal auditory canal (IAC) and the inner ear structures such as the cochlea and vestibule (Table 16‑1 ). The MRI and CT imaging can be fused as well to allow a hybrid-type image to visualize the tumor optimally (MRI), as well as the surrounding inner ear structures (CT). In addition, nonstereotactic imaging such as FIESTA (Fast Imaging Employing Steady-state Acquisition), heavily T2-weighted, thin-slice axial imaging, obtained prior to GKRS for diagnostic purposes, can be coregistered with the stereotactic images on the day of GKRS in some cases for more definite estimation of the degree of fundal extension, for instance. Of note, all the stereotactic imaging must have a large enough field of view (FOV) to incorporate the fiducial markers to allow stereotactic registration. In our experience, it is difficult to acquire FIESTA imaging, for example, with a sufficiently large FOV without a bothersome amount of distortion while the patient is in the stereotactic head frame. All the imaging is transferred via the Ethernet to the planning computer workstation.
Skull and frame measurements are taken per a standardized protocol, and likewise entered into the workstation. These measurements provide an estimation of the size and shape of the patient’s head, which assists in the computerized calculation of the dose contribution of each individual beam of radiation to the total dose. They also serve as a check to make sure there will not be any collisions between the head frame or the patient’s head and the treatment unit.
16.2.4 Dose Planning and Treatment
Treatment planning has been greatly facilitated by progressive improvements in the Gamma Plan software over the past 20 years. As detailed earlier, a combination of isocenters is selected, placed, and differentially weighted to create a highly conformal dose plan primarily based on the post-gadolinium axial SPGR (Spoiled Gradient Recalled Acquisition in the Steady State) imaging dataset (Fig. 16‑3 ). There has been a trend toward more isocenters per plan over the past two decades, but the plan may be rarely as simple as a single isocenter to more than 20, depending on size and shape of the VS. From 1990 to 1997, a total of 88 VS patients were treated at Mayo Clinic with a mean number of 5.3 isocenters per case, and between 1997 and 2004, the mean number of isocenters increased to 7.9 per case (n = 205 patients).s. Literatur The evolution of dose planning, dose selection, and subsequent reduction in morbidity from the first decade of our practice is outlined in Table 16‑2 . During the last decade, there has been significant interest in cochlea dose as a possible risk factor for hearing loss following GKRS, and therefore blocking of certain sections of the isocenter can be employed to try and limit dose extending in the direction of the cochlea.

Almost all tumors are treated at the 50% isodose line, by convention, as this allows for the steepest dose fall off. The great majority of VSs are now treated with 12.5 Gy to the 50% isodose line. A few years ago, the physicists at Elekta AB calculated a new tissue maximum ratio (TMR), thus effectively changing the calculated dose delivered; and therefore to stay consistent with our dose practice from the first 25 years of our experience, we increased the prescribed dose by an additional 0.5 to 1 Gy to deliver the same amount of radiation as had been previously used for VS. The latest version of Gamma Plan will provide the calculated dose for both the old and new TMR; so, the dose can be precisely adjusted if desired.s. Literatur For patients with useful ipsilateral hearing (i.e., >50% SDS), we most commonly prescribe 12.5 to 13 Gy, and for patients who are already functionally deaf in their tumor ear, we use 13.5 to 14.5 Gy to the 50% isodose line. For larger tumors (i.e., volume >10 cm3), we usually limit the prescription dose to 12 Gy in order to reduce the risk of treatment-associated edema and hydrocephalus. While other centers have published series using very low-dose GKRS for VS, we have been reluctant to use less than 12 Gy for any indication as we worry about a possible decreased efficacy below this dose.
There has been much discussion in the radiosurgical literature regarding the influence of cochlea dose on hearing outcomes for VS.s. Literatur , s. Literatur , s. Literatur , s. Literatur , s. Literatur , s. Literatur , s. Literatur As a result, we routinely contour the cochlea on the planning of thin-slice CT of the temporal bones, and Gamma Plan allows determination of a mean, minimum, and maximum dose to the cochlea. However, we have not found cochlea dose to be an independent factor in predicting hearing outcome when accounting for other confounding factors.s. Literatur Likely, the cause for hearing deterioration after GKRS is multifactorial and is most heavily impacted by hearing status at the time of treatment, volume treated, and marginal dose. To a lesser degree, loss of serviceable hearing may also be impacted by radiation dose to the cochlear nuclei in the brainstem, the cochlear nerve, and various aspects of the cochlea such as the stria vascularis—a structure that is thought to be particularly sensitive to the effects of ionizing radiation.s. Literatur Based on current evidence in the literature, we attempt to limit the dose to the cochlea as much as feasible, but never at the expense of whole tumor coverage. That is, we always fully treat the lateral extent of the tumor as dictated by the detailed imaging at the time of radiosurgical planning, and we do not reduce the tumor marginal dose below 12 or 12.5 Gy to specifically reduce cochlea dose. Therefore, in our experience, if the tumor extends to the fundus of the IAC, there is no possibility of keeping the mean dose to the cochlea below 6 Gy for instance, and in some cases the cochlea dose may be as high as 10 Gy.s. Literatur , s. Literatur We do not routinely calculate dose to any other structures such as brainstem or trigeminal nerve, for instance, during dose planning.
During treatment, the head frame is locked into the treatment couch with the patient in a comfortable supine position. The patient’s head can be elevated or lowered to maximize comfort. The machine then moves the patient automatically, in turn to each x, y, and z for each individual shot for the prescribed time until the treatment is complete. Depending on the number and size of isocenters used, dose prescribed, age of the Co60, and to a lesser extent size of the patient’s head, treatment times may vary from as little as approximately 20 minutes to as long as 2 hours. Once treatment is complete, the patient is removed from the gamma unit, the stereotactic head frame is removed, a light head dressing is applied, and the patient returns to the outpatient observation area. We administer 4 mg of intravenous dexamethasone after completion of the treatment, and after approximately 2 hours the patient is discharged. A minority of patients will develop significant scalp, periorbital, or facial edema related to the moderately large volume of local anesthetic used during head frame placement and occasionally some will report experiencing complete unilateral eye closure related to swelling; however, this edema always resolves within 48 to 72 hours with head elevation and application of cold compresses. Pin site infection requiring oral antibiotics has occurred in less than a dozen cases in more than 6,000 head frame placements at our institution. There have been no cases of osteomyelitis or need for intravenous antibiotic treatment.
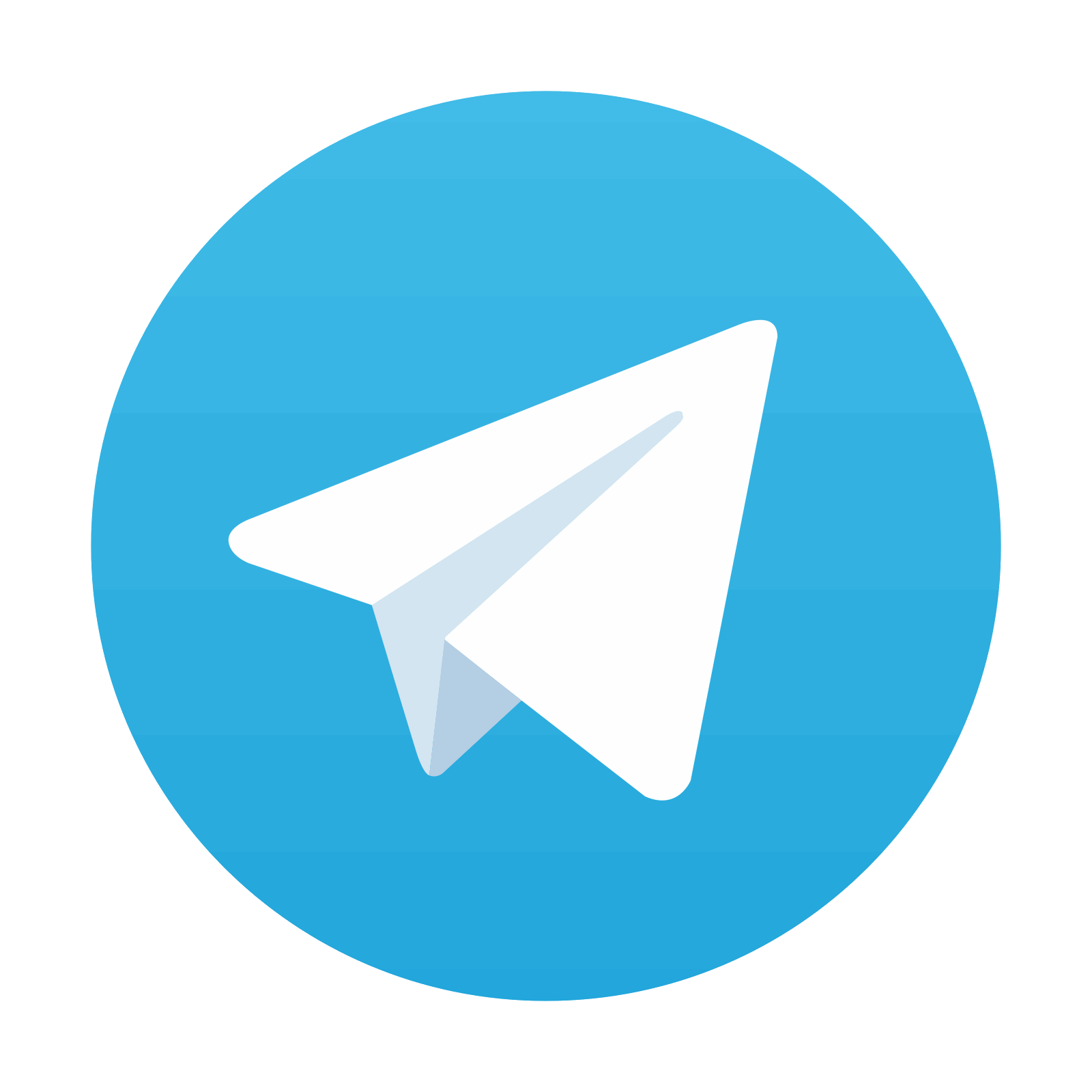
Stay updated, free articles. Join our Telegram channel

Full access? Get Clinical Tree
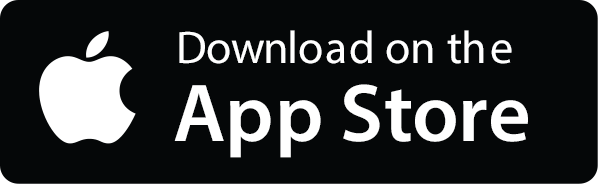
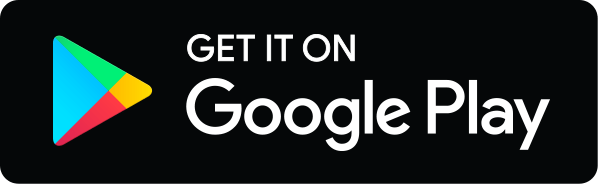
