17 Motor, Sensory, and Language Mapping in Epilepsy Surgery
Abstract
Motor, sensory, and language mapping techniques have greatly expanded the scope of safe resections for pharmacotherapy-resistant epilepsy. Due to individual variability in topographical patterns of function, particularly in patients with electrophysiological or structural abnormalities, anatomic landmarks and structural imaging are insufficient to minimize the chance of a postoperative deficit when operating near eloquent cortex. Various imaging modalities, Wada testing, and extraoperative (stimulation) mapping may be useful adjuncts, but none have matched the reliability of intraoperative electrical stimulation mapping (ESM). Fortunately, a variety of intraoperative techniques have been developed and refined to make them relatively safe and reliable, though building an experienced team is critical. Appropriate patient and task selection is also essential for reliability. While there remains variability in approaches between institutions and uncertainty with regard to the implications of certain intraoperative findings for postoperative functional recovery, there is a growing understanding of the predictive ability of ESM with specific tasks and in various anatomic locations. The identification of essential functional cortex near or within the epileptogenic zone may limit the extent of surgery, and it is critical to establish the patient’s priorities preoperatively. Continued diligent data collection combined with adjunct imaging techniques will likely lead to further improvement in the reliability of mapping techniques for epilepsy surgery.
17.1 Introduction
Surgical resection is an effective and important treatment option for patients with medically refractory epilepsy in whom the seizure onset zone can be well localized. 1 However, the epileptogenic focus may overlap with eloquent cortex, including regions integral to motor, sensory, and language functions. As such, resections performed in epileptic patients have historically carried a risk of producing postoperative deficits. 2 , 3 In order to minimize these deficits, several methods can be employed to map function and guide safe resections.
17.2 History
Early in the history of resective procedures for epilepsy, most of the left hemisphere was deemed “forbidden territory” so as to protect patients from postoperative aphasia and other language deficits. 4 , 5 Based on initial understanding of the topographical organization of the cortex, surgeons attempted to preserve function by a variety of anatomic landmarks. 6 , 7 , 8 However, resections adhering to most of these guidelines, particularly for language preservation, have been associated with postoperative deficits. 9
In the late 1800s, it was discovered that direct electrical stimulation (DES) could influence cortical activity. 10 Within a few decades, this tool was used in patients undergoing awake epilepsy surgery to map out cortical functions, 11 beginning with the demarcation and subdivision of motor and sensory cortex. 12 , 13 Although DES evoked “positive” responses in motor and sensory areas (e.g., observed movement or subjective sensation), DES in language cortex was found to generate “negative” responses, impairing relevant task performance. 14 , 15 Penfield and Roberts used electrical stimulation mapping (ESM) to identify cortex where DES interfered with object naming and they thereby developed a representation of language function. 4 Ojemann and colleagues later described patterns and variability of language function and found that resection of sites where DES repeatedly interfered with naming predicted postoperative language deficits. 7 , 16
The importance of intraoperative identification of patient-specific language, motor, and sensory sites has been underscored by findings of substantial variability in the localization of cortical functions, particularly in patients with neuropathologies including epilepsy and mass lesions. 14 , 17 , 18 By using intraoperative ESM to localize and then spare cortex critically involved with language, movement, and sensation, surgeons have expanded the scope of safe resections for pharmacotherapy-resistant epilepsy. 4 , 19 , 20 More recently, various methods of preoperative functional and anatomic mapping, including magnetic resonance imaging (MRI), 21 , 22 functional MRI (fMRI), 23 , 24 magnetoencephalography (MEG), 25 , 26 positron emission tomography (PET), 27 , 28 and diffusion tensor imaging (DTI), 29 , 30 have also been explored as potential tools for surgical planning.
17.3 Patient Selection and Preoperative Functional Localization
The candidates for epilepsy surgery most likely to benefit from motor, sensory, and language mapping are those who exhibit related seizure semiology, as the onset pattern has implications for localization of the epileptogenic zone. For instance, features such as early speech arrest may indicate involvement of language regions, 31 whereas presentations such as Jacksonian march may indicate involvement of motor cortex. 32
Cortical localization of function is difficult to perform in patients with severe baseline deficits in the functions that are to be mapped. Language mapping may therefore be challenging in patients with poor language abilities (<70–75% accuracy on object naming tasks), while motor mapping may be challenging in patients with significant hemiparesis. Specifically, baseline deficits make it difficult to determine whether performance errors during stimulation are attributable to the stimulation or are merely reflective of baseline difficulties. Although ESM has been performed in patients as young as 2 years old, 33 mapping is used less frequently in children, in part because the cortex of young children appears relatively nonexcitable to DES (although this dampening of excitability may be overcome by complex stimulation parameters). 34 , 35 Also, children and uncooperative adults may find it difficult to tolerate intraoperative awake stimulation. 5 In these patients, asleep intraoperative mapping of motor function or extraoperative mapping of sensorimotor or language function via noninvasive imaging or implanted subdural or stereoelectroencephalography (SEEG) electrodes may be an alternate, albeit less optimal option.
Structural MRI can be used to initially assess the proximity of epileptogenic zones from likely eloquent cortical areas, particularly in cases where there is a clear lesional onset. The central sulcus can reliably be localized relative to the marginal ramus of the cingulate sulcus on axial and parasagittal imaging (▶Fig. 17.1), and the sulcal pattern of the dominant hemisphere frontal operculum can be used to approximate the ventral premotor speech motor area. However, given the substantial variability in the representation of function in these regions, particularly language function, 21 , 36 structural MRI is probably best used to identify candidates for further intraoperative mapping.

Other studies can inform the approach to mapping and resection, and guide shared preoperative decision making, but none have matched the reliability of stimulation mapping. Amobarbital (Amytal) Wada testing, also known as the “intracarotid amobarbital procedure (IAP),” has historically been the gold standard for determining language lateralization and hemispheric capability of memory support. 37 , 38 By catheterizing the internal carotid arteries of patients without significant cerebral vascular abnormalities and injecting them separately with amobarbital sodium, the procedure induces temporary hemianesthesia, enabling assessment of function of each hemisphere, separately. 39 , 40 If amobarbital is unavailable, there are viable alternative anesthetic agents including etomidate, methohexital, pentobarbital, or propofol. 41 Wada testing is, however, an invasive procedure carrying the inherent risks of angiography. 42 In addition, while more selective (i.e., posterior cerebral artery) Wada testing can be used to suppress hippocampal memory function while preserving language, Wada testing cannot localize language function beyond laterality.
Task-based fMRI, MEG, and PET have shown high concordance with Wada testing in identifying language laterality 24 , 28 , 43 , 44 and general language areas, 27 , 45 and have replaced Wada testing at some centers. However, the capacity of these modalities to identify more precise language regions has been inconsistent relative to ESM, 46 , 47 at least in part because of variations in functional maps generated by different tasks and protocols. 48 The preoperative use of fMRI may also be limited by its nonspecificity for areas of cortex essential for language, as opposed to more broad areas activated by language tasks. 49 DTI allows visualization of subcortical white matter tracts, which can provide another method for localizing eloquent cortex 29 , 30 and provide a more complete view of all relevant functional anatomy, particularly in cases of lesional epilepsy where tracts may be displaced. Applying these modalities in conjunction with ESM may improve overall sensitivity and specificity. 50 , 51
Extraoperative stimulation through implanted electrodes offers another opportunity for mapping. Extraoperative monitoring with implanted intracranial electrodes is frequently used to confirm or identify the epileptogenic zone. 52 After seizures have been observed and localized, antiepileptic drugs are returned to therapeutic levels and the recording electrodes can be stimulated to map functions. 53 ▶Fig. 17.2 (a, b) demonstrates the operative placement of subdural strips and grids as well as the resulting schematic of the proposed resection based on localization of the seizure onset zone and extraoperative language mapping. Electrodes can also be implanted with the primary goal of extraoperative mapping in patients who may not tolerate intraoperative mapping. However, the technique has several limitations. Extraoperative stimulation mapping is limited by the predetermined locations of the electrodes. Subdural strip and grids techniques are of limited utility for deep investigations and require relatively large craniotomies that may be impractical or excessively morbid for bilateral or multilobar studies. SEEG, long used at several European centers, is growing in popularity in the United States, with the adoption of robotic assistance 54 and accumulating evidence of safety. 55 SEEG carries the advantages of being able to sample deep, bilateral, and multilobar regions with relatively low complication rates. 56 , 57 However, it provides less electrode coverage on the cortical surface, limiting the precise differentiation between contiguous cortical areas. 58 , 59 , 60 There is likely a learning curve in using the information it provides for both localization and mapping. 61

While many extraoperative mapping methods can identify sites involved with language, these sites may not be essential and may not be reproduced by intraoperative DES. 62 , 63 As such, while extraoperative techniques can inform surgical planning, they are not a commensurate substitute for intraoperative ESM. 63 ▶Fig. 17.2 (c, d) further demonstrates additional language sites identified by ESM after extraoperative mapping and the final resection. To prepare for intraoperative language mapping, baseline testing is performed on the tasks to be used. For ESM to proceed efficiently, it is important to identify tasks and stimuli that the patient can perform at ease at baseline. Although baseline can shift in the operating room (OR) setting due to sedation and psychological stress, this allows the surgical team to be reasonably certain that any errors that occur with stimulation are, in fact, due to the stimulation. Otherwise, additional time will be required to determine whether the error rate during stimulation is significantly different from the patient’s baseline error rate using “unselected” tasks and test items. For both motor and language mapping, preoperative counseling with the neuropsychologist or other practitioner who will be directing the patient is helpful in order to prepare the patient for the awake portion of surgery. Antiepileptic drug levels are monitored to ensure that, at the time of surgery, they are in the high therapeutic range in order to minimize the risk of evoking a seizure during ESM.
17.4 Intraoperative Stimulation Technique
Certain precautions should be taken in the preparation for awake mapping. Patient positioning is important for safety and comfort. In cases of temporal lobe language mapping, we prefer the lateral position with the falx approximately parallel to the floor. An axillary roll or saline bag can be used to protect the lower brachial plexus and the neck is kept in a neutral position in the Mayfield head frame in order to maximize comfort and protect the patient’s airway during sedation. The airway can also be protected with temporary use of a laryngeal mask or nasal trumpet. 64 Patients are typically anesthetized with a combination of propofol for sedation and a short-acting opiate such as remifentanil. A combination of short- and long-acting local anesthetics (e.g., lidocaine and bupivacaine) are used at the pin sites, skin incision, and in the temporalis muscle. 5 , 65 In order to minimize afterdischarges (AD) and seizure activity, mapping is conducted with antiepileptic drugs at therapeutic levels. 66 Cold saline (4°C) should also be kept at hand, as it is a practical and effective method for terminating stimulation evoked seizures. 67
In developing a patient’s mapping strategy, individual needs and the presumed seizure onset zone must be taken into account. 68 The seizure onset zone is particularly susceptible to stimulation-induced seizures, which can be dangerous and may prompt the discontinuation of ESM because of postictal decline in ability. This region is often tested last. 5 However, this factor should be balanced against intraoperative time considerations, namely, the patient’s ability to tolerate a long mapping procedure. 69 Testing near the seizure onset zone is the most critical to determining the functionality of tissue being considered for resection. 5
For language mapping, DES is applied to an interrogated site at the onset of the task, and error rates are compared either to baseline rates (i.e., control trials without stimulation) 70 , 71 or to set cutoffs. 70 , 72 During ESM, different neurosurgeons tend to use a fairly consistent set of technical parameters which have been well established. 5 , 71 , 73 These parameters are designed to minimize the risk of evoking a seizure while still effectively interfering with the action of eloquent cortex. An upper threshold current for AD is therefore determined prior to language testing and the DES current is typically set 0.5 to 1 mA below the AD threshold with a 10-mA maximum. 5 In practice, when a patient is awake in the OR, it is rarely necessary to stimulate beyond 4 to 5 mA of delivered stimulation (2–2.5 mA on the bipolar Ojemann stimulator setting) to induce either changes in patient function or AD. Stimulation up to 10 seconds with this amount of current has been observed to cause no histological damage to the human brain, 74 though much shorter durations (3–4 seconds) are typically used clinically. Constant current is usually delivered in a biphasic square waveform with 1- to 1.25-ms single-phase duration at frequencies of 50 to 60 Hz. 66 , 69 , 75 For comprehensively mapping the cortical boundaries of language, on the order of about 15 to 20 sites regularly require testing. Positive sites can be labeled with small paper tags or pieces of Gelfoam and photodocumented (▶Fig. 17.2c). Each potential language site is stimulated a total of four to six times, to test visual naming, auditory naming, and reading. This approach can be modified to focus on areas contained within the optimal planned resection. Areas where stimulation repeatedly produces errors—which may include semantic or phonologic paraphasias, nonresponses, neologisms, delays, and comprehension errors 6 —are considered central to language. Although positive sites (i.e., those where stimulation disrupts a task reliably) provide reassurance for the validity of negative stimulation sites, they are not essential to clear negative sites for safe resection if appropriate stimulation parameters are used. Fields of exposure need not be expanded when they solely consist of negative sites. 76
For sensory and motor mapping by DES, trains of biphasic square waveform pulses with frequencies of 50 to 60 Hz and single-phase durations of 0.2 to 0.5 ms are typically used. 6 Stimulation tends to begin at low levels of current (e.g., 2 mA), which is increased in 0.5- to 1-mA increments until a response is elicited or a maximum current, set as in language mapping, is reached. The patient is asked to repeatedly perform a motor task (i.e., hand opening and closing) and monitored for impaired function during stimulation, or is at rest and monitored for movement through observation and electromyographic (EMG) recording of the appropriate muscles. 77 Stimulation can also interrogate subcortical white matter for descending motor tracts, using the same stimulation amplitude as the overlying cortex. Elicited responses can include dysesthesias or paresthesias (which must be communicated by the patient), or compound muscle movements (particularly of the upper extremity). 78 Motor sites can also be localized by testing for mild weakness during stimulation, for instance, testing for hand grip or more proximal weakness if the resection approaches the hand motor area. 79 Motor mapping can be done with the patient either awake or asleep, while sensory mapping via DES requires a responsive and communicative patient.
Somatosensory evoked potentials (SSEPs) represent another method to identify primary motor and sensory cortex. They have the advantage over DES of not evoking seizures. They can readily be performed reliably in patients under general anesthesia. 80 SSEPs involve stimulating the median or tibial nerves (usually 2–5 Hz with 0.1–0.3 ms pulse duration), which produces a robust and mirror-image waveform across the central sulcus. 81 This waveform is measured by a strip electrode placed transversely to the presumed orientation of rolandic cortex, 4 cm above the sylvian fissure for the median nerve and by the vertex or along the medial paracentral lobule for the tibial nerve. Stimulation of the median nerve is generally easier to use for localization. It typically generates a negative wave after a latency of 20 ms and a positive wave after 25 ms (N20/P25) in the postcentral gyrus and the inverse P20/N25 in the precentral gyrus. 82 The changing wave polarity between the pre- and postcentral gyri in a bipolar montage, known as “phase reversal,” can be used to identify the central sulcus (▶Fig. 17.3). The trigeminal nerve can also be stimulated to determine facial motor and sensory areas. 83

The safety of resection around motor cortex can also be evaluated by monitoring motor evoked potentials (MEPs). After the central sulcus has been identified by ESM or SSEPs, the location of cortex with the largest SSEP phase reversal or the lowest motor stimulation threshold is repetitively stimulated while MEPs are recorded from various contralateral muscle groups (e.g., forearm flexors, thenar eminence, quadriceps muscle). 84 , 85 , 86 Stimulation is typically performed in short trains of around five monopolar anodal rectangular pulses of 0.2 to 0.5 ms at 200 to 800 Hz, and the intensity is increased stepwise until action potentials are elicited in targeted muscles or an arbitrary limit (e.g., 20 mA) is reached. 84 , 85 , 86 MEPs can then be observed continuously through surgery, for instance at two to three times per minute. 85 Irreversible loss of MEPs, reductions in amplitude (>80%), or prolongations in latency (>15%) are meaningful predictors of the likelihood and severity of lasting postoperative motor deficits. 85 , 86 , 87
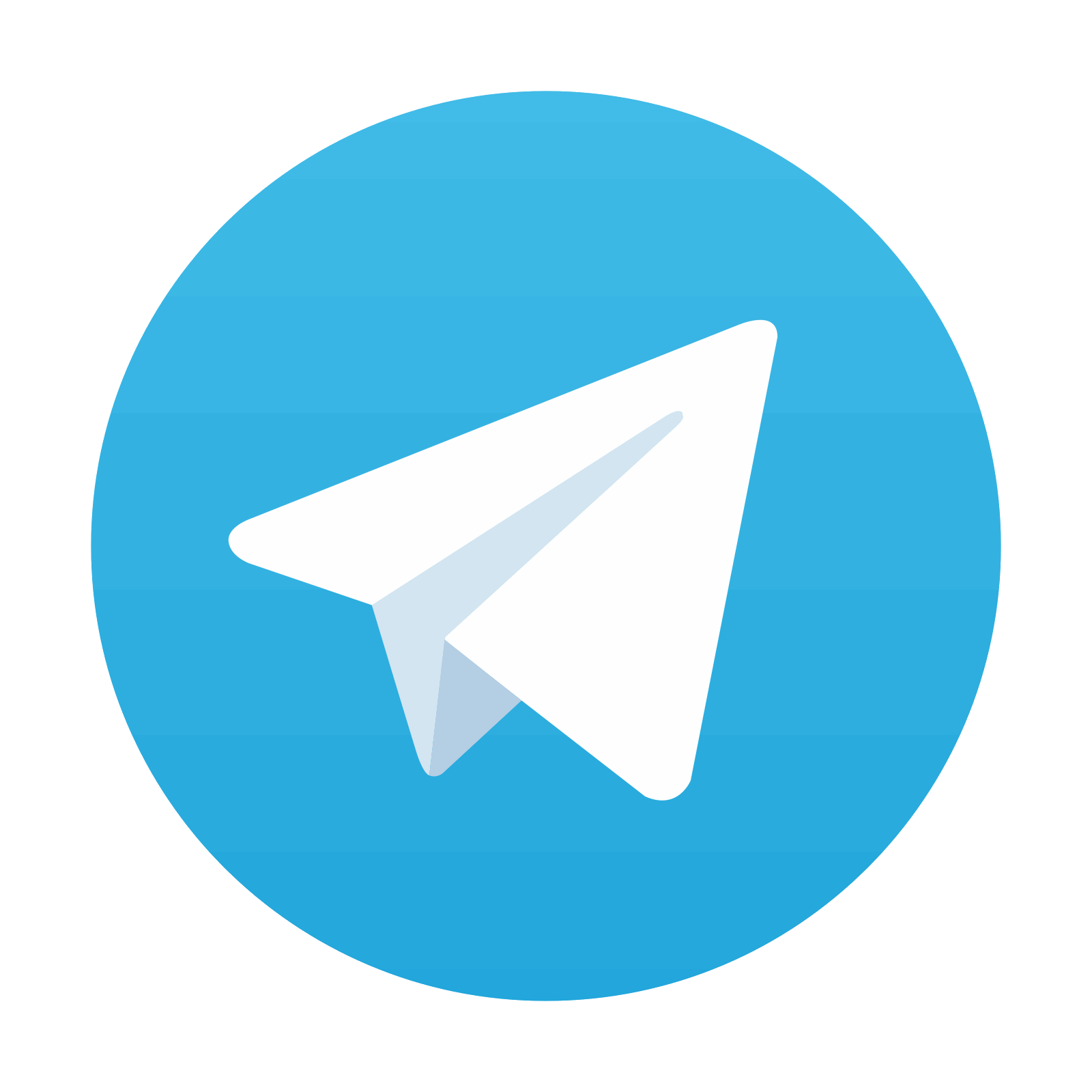
Stay updated, free articles. Join our Telegram channel

Full access? Get Clinical Tree
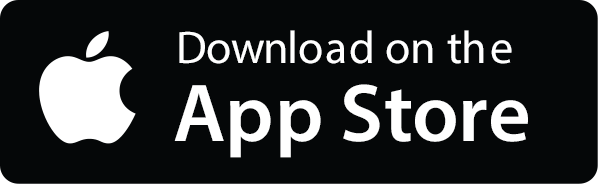
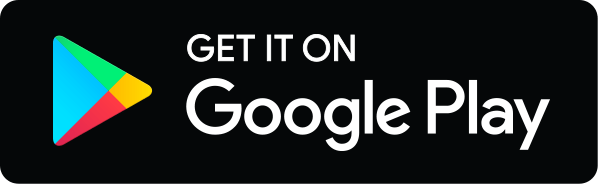