18 Conventional Fractionation LINAC-Based Stereotactic Radiotherapy for Treatment of Sporadic Vestibular Schwannoma
18.1 Introduction
Conventional fractionated radiotherapy refers to the fractionation schedule that uses a daily dose of 1.8 to 2.0 Gy with five daily fractions per week. The entire treatment course usually is 5 to 6 weeks with total treatment doses in the range of 45 to 54 Gy for benign intracranial tumors. This method of treatment is widely used for benign and malignant primary brain tumors, with proven efficacy and a favorable toxicity profile.
18.2 Fractionated Stereotactic Radiotherapy
Conventional fractionated radiation treatment originally utilized 3D conformal technology. Over the past several decades, the development of stereotactic radiation techniques has allowed treatment to be performed with greater conformity, fewer complications, and potentially improved tumor control. Fractionated stereotactic radiotherapy (FSRT) has the radiobiological advantages of conventional fractionated radiotherapys. Literatur while maintaining the same superior dose conformity, precision, and steep dose falloff outside the target volume of stereotactic radiosurgery (SRS). In essence, FSRT has the same radiation dosimetric characteristics as SRS with the only difference being the number of fractions and dose per fraction.
Currently, several different stereotactic systems are available for patient treatment, including infrared camera guidance,s. Literatur relocatable frame,s. Literatur , s. Literatur , s. Literatur implanted fiducial markers,s. Literatur and thermoplastic mask fixation system.s. Literatur , s. Literatur , s. Literatur The thermoplastic head mask is most widely used due to its convenience and patient comfort. High precision can be reproducibly achieved with the current FSRT systems. One study using the Gill-Thomas-Cosman (GTC) relocatable frame demonstrated a total 3D displacement of 1.8 mm ± 0.8 mm (mean ± SD) with a range of 0.3 to 3.9 mms. Literatur which is similar to mask-based systems where the 3D displacement is usually less than 2 to 2.5 mm.s. Literatur , s. Literatur Accuracy of patient positioning reproducibility with a stereotactic mask fixation system can be further improved with daily imaging guidance (image-guided radiation therapy). There are three types of in-room kilovoltage (kV) X-ray imaging guidance systems commonly used in current treatment centers. CT-on-rails is a rail-track–mounted kV tomographic imaging system that consists of a conventional CT scanner installed in the treatment room such that it can be moved into a position for acquiring helical CT scans of the patient on the treatment couch. The stereoscopic X-ray imaging system utilizes kV X-ray tubes that are mounted permanently either to the ceiling or floor such that the tube and detector locations are fixed relative to the treatment room coordinates. Examples of this type of system are ExacTRAC (BrainLab, Westchester, IL) and CyberKnife (Accuray, Sunnyvale, CA). Gantry-mounted systems, such as Varian On-Board Imager (OBI) and Elekta X-ray Volume Imaging (XVI), rely on X-ray tube and detectors that are mounted on the treatment gantry orthogonal to the central axis of the treatment beam. The kV imaging system rotates along with the gantry and shares the same isocenter as the MV treatment beam. It can generate radiographs, fluoroscopic images, as well as tomographic images such as CBCT, which uses a cone-beam X-ray source that encompasses a large volume with a single rotation about the patient. Images are then reconstructed into 3D images. The ceiling/floor-mounted stereoscopic X-ray imaging system and gantry-mounted system can also be integrated into a single treatment room, such as the Novalis Tx (BrainLab, Westchester, IL) or Truebeam STx (Varian, Palo Alto, CA) configurations.
In order to quantify the end-to-end positioning uncertainty of an image-guided fractionated stereotactic radiotherapy (IG-FSRT) procedure, several independent groups have reported their results of hidden target tests, which involved simulating a complete typical treatment of a hidden metal target in a rigid phantom with measurement of the localization accuracy. The average reported mean localization error is 0.8 mm.s. Literatur , s. Literatur , s. Literatur , s. Literatur , s. Literatur , s. Literatur , s. Literatur , s. Literatur As a result of the modern technology, a planned target volume (PTV) margin of 1 to 2 mm is sufficient. Such small PTV margin for vestibular schwannoma treatment allows better sparing of critical brain structures adjacent to the tumor, such as the brainstem and cochlea. Moreover, advanced radiation treatment planning, such as intensity-modulated radiation therapy (IMRT) and volumetric arc therapy (VAMT), can further carve the radiation distribution to achieve additional normal structure sparing which is unable to be performed with the 3D conformal techniques. Literatur (Fig. 18‑1 ).

18.3 Radiobiology
Understanding the basic radiobiological rationale of FSRT will help interpret the clinical data, particularly the differences in efficacy and toxicity profile between FSRT and SRS for the treatment of vestibular schwannomas. The relationship between time, dose, fractions of FSRT, and its biological effect is based on basic principles of radiology, defined as the “4Rs” (repair, repopulation, redistribution, and reoxygenation) of radiation.s. Literatur The most important biological principle that influences FSRT for vestibular schwannoma is “repair.” Fractionation allows time between treatments for repair of sublethal damage. Due to faulty repair mechanisms, vestibular schwannomas are less efficient at repair compared to normal tissue and these differences between tumor and normal tissue are magnified with increased number of fractions.s. Literatur , s. Literatur This leads to the separation of normal tissue with highly fractionated radiation treatment. Fractionation also allows reoxygenation of tumor tissue and redistribution of cells in cell cycle. The ultimate result is an increased therapeutic index of radiation. The radiobiology of high-dose single-fraction treatment exerts major effects through lethal DNA damage and endothelial damage.s. Literatur Though SRS may be favorable for tumor cell kill for vestibular schwannomas due to its low α/β ratio, it also has more adverse effects on the normal structure, such as injury to the cochlea and cochlear nerve.s. Literatur , s. Literatur Protection of normal tissue is achieved through steep dose fall off and high conformity of SRS technology. Unfortunately, due to the adjacent proximity of vestibular schwannomas to the cochlea and overlapping proximity with the cochlear nerve, these structures frequently cannot be adequately spared. Damage to the cochlear sensory cells, particularly the hair cells of organ of Corti, and cells of the stria vascularis is one major mechanism of radiation-induced hearing loss.s. Literatur
The biological effects of SRS and FSRT on hearing can be further estimated based on the RET formulas. Literatur which implies that the SRS dose should not exceed a threshold of around 11.2 Gy to obtain high rates of hearing preservation. Similarly, a cumulative FSRT dose above 45 Gy could result in higher rates of hearing loss. Based on our previous analysis, even a median value of 13 Gy has resulted in serviceable hearing losses ranging from 17 to 67%, while a median FSRT dose of 47.5 Gy has resulted in serviceable hearing losses ranging from 29 to 43%.s. Literatur These findings are further supported by clinical evidence of loss of serviceable hearing as being statistically related to a threshold dose to the cochlea above 40 Gy.s. Literatur , s. Literatur , s. Literatur Further discussion regarding the principles of radiobiology relevant to SRS and FSRT for vestibular schwannoma can be found in Chapter 15.
18.4 Tumor Control
FSRT was initially used as adjuvant therapy following incomplete surgical resection of vestibular schwannomas. In 1987, Wallner et al reported their initial experience, demonstrating that postoperative irradiation (>45 Gy) decreased the recurrence rate after subtotal resection from 46 to 6%.s. Literatur Subsequently, stereotactic radiation therapy has been demonstrated to achieve excellent tumor control equivalent to surgical resection, and may be effectively used as a definitive treatment option. The efficacy of primary radiation treatment for vestibular schwannomas was first established with Gamma Knife SRS.s. Literatur Over the past two decades, multiple studies have reported tumor control rates utilizing FSRT comparable to those achieved with SRS, potentially with lower risk of cranial nerve morbidity (Table 18‑1). A meta-analysis of 3,677 patients treated with SRS reported a 91% tumor control rate.s. Literatur Several large retrospective series of FSRT with long-term follow-up showed local control rate exceeding 94%.s. Literatur , s. Literatur , s. Literatur , s. Literatur , s. Literatur Other FSRT studies have reported a wider range of tumor control rates between 85 and 100% (Table 18‑1). The variance in results is largely related to the length of follow-up and definitions of tumor control. It is well recognized now that after FSRT, there may be transient tumor expansion (Fig. 18‑2 ).s. Literatur , s. Literatur , s. Literatur In the initial period after treatment, tumors may increase in size because of the effects of radiation, followed by a period of stabilization and often subsequent shrinkage. This transient expansion has been observed in 14 to 74% of patients at 9 to 16 months.s. Literatur , s. Literatur , s. Literatur In order to properly account for this phenomenon, definition of treatment failure should involve sustained tumor growth on two consecutive follow-up MRI scans separated by an adequate time period.s. Literatur , s. Literatur Another important factor for accurate assessment of tumor control is adequate follow-up. Series with long-term follow-up demonstrated decreased local control with over time, though failure is rare after 5 years.s. Literatur , s. Literatur , s. Literatur

Despite the overall high success rate of achieving long-term tumor control, the optimal dose and fractionation scheme for FSRT remains uncertain. There is a widespread variation in treatment practices at different institutions. The most commonly used radiation doses range from 45 to 57.6 Gy, administered in 1.8 to 2 Gy fractions (Table 18‑1). Fuss et al reported their experience of 51 patients treated with FSRT to the dose of 57.6 Gy.s. Literatur They demonstrated actuarial 2- and 5-year tumor control rates of 100 and 97.7%, respectively. The actuarial serviceable hearing preservation rate at 2 and 5 years was 85%. Lower doses were explored to reduce toxicity and improve hearing preservation, while maintaining tumor control. Andrews et al performed a nonrandomized prospective cohort analysis comparing outcomes after FSRT dose reduction from 50.4 Gy to 46.8 Gy.s. Literatur This study successfully improved hearing outcomes without reduction of tumor control. Long-term follow-up of the low-dose 46.8 Gy cohort showed tumor control rates at 3 and 5 years of 99 and 93%, respectively. The overall serviceable hearing preservation was 67% with 3 and 5 year rates of 66 and 54%, respectively.s. Literatur Importantly, hearing impairment after FSRT appears to have a delayed time to onset relative to SRS.s. Literatur Several series using doses as low as 45 Gy also showed similar high tumor control probability.s. Literatur , s. Literatur , s. Literatur However, doses lower than 45 Gy, particular lower than 40 Gy, appear to be associated with inferior tumor control.s. Literatur , s. Literatur Based on the current data, it seems that no dose–response relationship exists beyond 45 to 46.8 Gy, and this dose range may represent the threshold for high tumor control probability. Given the potential for reduced side effects and improved hearing preservation, low-dose FSRT (45–46.8 Gy) should be considered. Further dose de-escalation below 45 Gy should be carefully performed in the prospective setting with long-term follow-up.
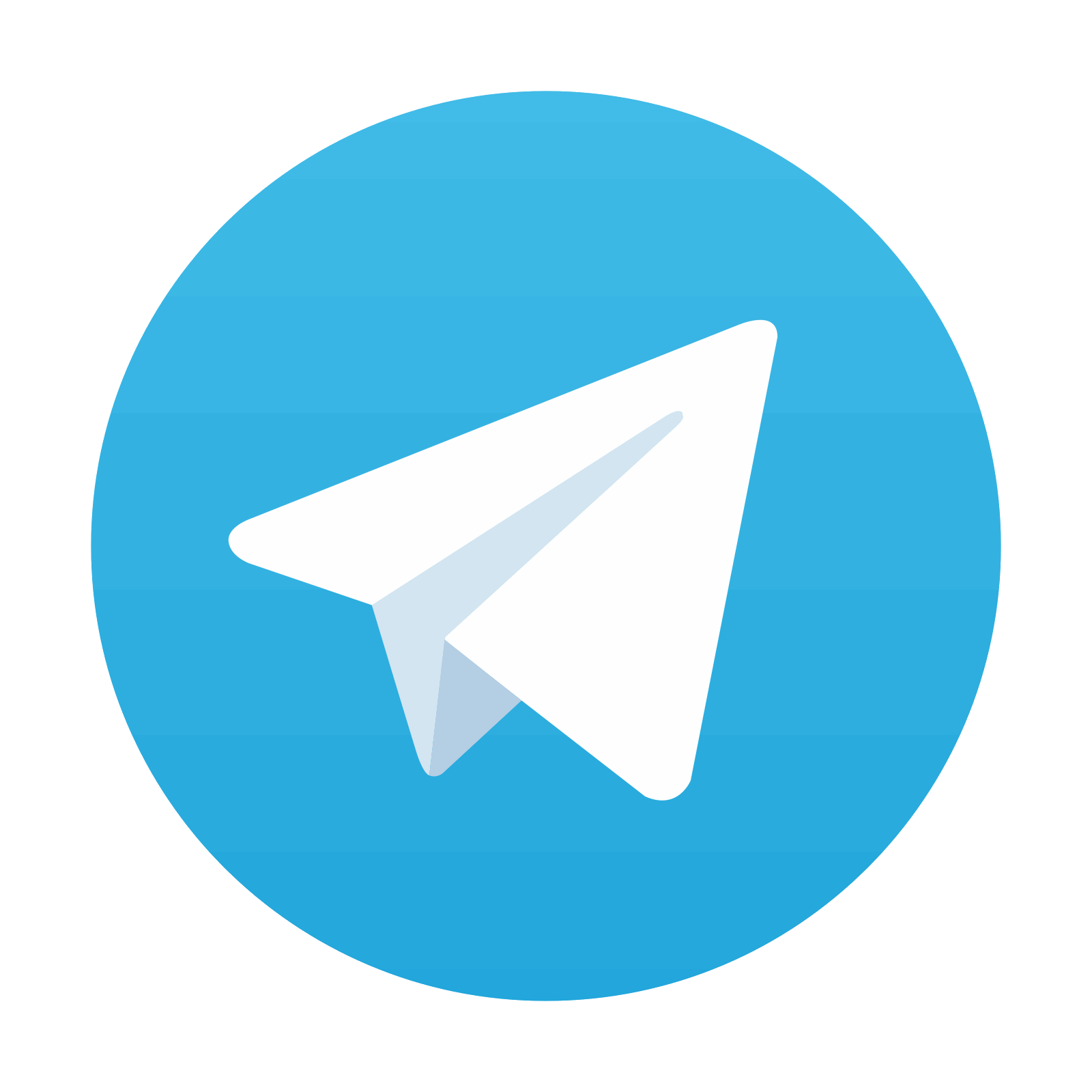
Stay updated, free articles. Join our Telegram channel

Full access? Get Clinical Tree
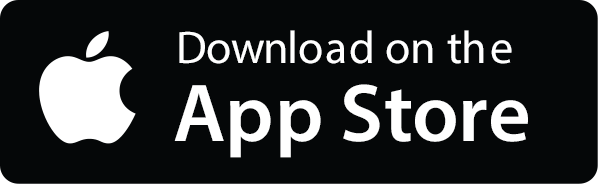
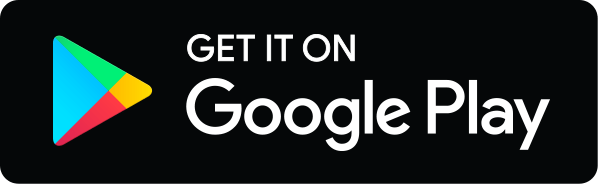