18 Halo-Gravity Traction: An Adjunctive Treatment for Severe Spinal Deformity
Abstract
Halo-gravity traction (HGT) has evolved from halo-femoral and halo-pelvic traction to become a safe and effective adjunctive tool in the treatment of severe spinal deformity in ambulatory and nonambulatory patients. HGT has been shown to potentially reduce the need for vertebral column resections as well as provide a means of optimizing a patient from a medical and nutritional standpoint prior to spinal surgery. HGT can be applied either before spinal surgery or between stages of a multistage procedure, with traction weight calculated based on a patient’s total body weight. The timing and duration of HGT is surgeon-specific and is typically based on curve severity and rigidity, as well as the health of the patient. The indications for pre- and/or perioperative HGT include severe scoliosis or kyphosis > 100 degrees with limited flexibility (<20%). Relative contraindications are cervical kyphosis and/or stenosis, ligamentous laxity, and open fontanelles. Complications during HGT are typically minor and include pin site irritation or infection, which can be treated with pin care, antibiotics, or pin removal. Neurologic injury, although extremely rare with HGT, has been reported. Studies on patients with HGT commonly involve a heterogeneous patient population; thus, it is challenging to reach a definitive consensus on indications and treatment protocols. However, a growing number of reports are substantiating the potential benefits of HGT for severe spinal deformity.
18.1 Overview
Since the late 1950s, various forms of halo traction have been utilized as an adjunctive form of treatment in spinal deformity. Perry and Nickel 1 revised Bloom’s fascial traction device to an aluminum tiara fixed to the skull with threaded pins. This was initially indicated for cervical paralysis related to poliomyelitis, but was later expanded to include severe scoliosis. 2 Several forms of halo traction have been described to date including halo femoral, 3 , 4 halo tibial, 5 halo pelvic, 6 and, more recently, halo gravity. 7 , 8 , 9
Halo-femoral traction was utilized by Kane and colleagues 3 in 30 patients with scoliosis in whom the average curve was reduced from 112 to 58 degrees after final correction. Bonnett and colleagues 4 later reported a 53% correction with halo-femoral traction for patients with paralytic scoliosis. However, 28 of 37 patients sustained fractures after treatment was completed, 17 of which involved the femurs during simple range-of-motion exercises posttreatment. This study highlighted the potential significant complications associated with halo-femoral traction and bed rest as it relates to the loss of bone mineral density.
Halo-gravity traction (HGT) was then introduced building on work by Klaus Zielke and Pierre Stagnara. 7 HGT offers a distinct advantage over the previous treatments in that it affords significantly more versatility. HGT may be applied in a bed, a wheelchair, and/or a walking frame, allowing the patient to be upright and out of bed during treatment 8 (Fig. 18‑1).

There are several published series on HGT for severe spinal deformity. 7 , 8 , 9 , 10 , 11 , 12 The study by Sponseller and colleagues 9 is the lone level III study of the existing literature on HGT in which a retrospective, multicenter, nonrandomized comparison study of pediatric patients with severe scoliosis was performed. In this study, patients were treated with or without HGT based on surgeon preference. The study found no statistically significant difference between the HGT and the non-HGT groups with regard to final curve correction, spinal length gain, blood loss, operating time, or complications. However, in this study, patients who underwent HGT required significantly fewer vertebral column resection procedures compared to patients who did not undergo HGT (3 vs. 30%, respectively; p = 0.015).
Rinella and colleagues 8 retrospectively reviewed 33 patients with severe scoliosis at a single center and demonstrated a 46% curve correction after HGT and spinal fusion with no neurologic deficits. No mention was made of curve correction while the patients were in HGT. Sink and colleagues 7 retrospectively reviewed 19 pediatric patients with severe scoliosis treated with HGT for 6 to 21 weeks and reported an average Cobb’s correction of 35%. Watanabe and colleagues 10 reported a single-center retrospective review of 21 consecutive pediatric patients with scoliosis >100 degrees. The study reported the greatest improvement in Cobb angle correction after 1 week of HGT (17.5%) and plateaued at 23.3% correction after 3 weeks of HGT. The reported final curve correction after fusion and HGT was 51.3%. No significant improvements in correction of the Cobb angle were noted with > 3 weeks of HGT.
Koller and colleagues 13 retrospectively reviewed 45 adult and pediatric patients with severe scoliosis and/or kyphosis treated with HGT. An improvement of only 8 and 7 degrees, respectively, was achieved in the coronal and sagittal planes with preoperative HGT, while a 9% improvement in forced vital capacity (FVC) was noted during preoperative HGT. Bogunovic and colleagues 11 reported an average deformity correction of 35%, with the majority of the correction occurring in the first 3 to 4 weeks of HGT (average HGT weight: 35.4% of total body weight [TBW]). Pulmonary function (FVC and forced expiratory volume 1 [FEV1]) similarly improved an average of 9% during HGT in this study.
Nemani et al 12 reported on 29 patients with an average coronal curve of 131 degrees who underwent HGT for an average of 107 days prior to definitive posterior spinal fusion or placement of growing rods. They reported an average major curve correction of 31% after HGT and a final correction of 56% postoperatively, with deformity correction plateauing at 63 days.
18.2 Preprocedure Planning
18.2.1 Indications and Contraindications for HGT
Radiographic indications for consideration of HGT vary considerably among studies and institutions; however, a coronal and/or sagittal Cobb angle of ≥ 90 to 110 degrees is typical. Curve flexibility has also been shown to play a role in consideration of HGT. Sponseller et al 9 utilized HGT for curves > 90 degrees with < 25% flexibility, while Watanabe et al 10 used the criteria of a Cobb angle > 100 degrees and < 20% curve flexibility as an indication for HGT. True contraindications have also not been fully agreed upon, but generally cervical kyphosis, cervical stenosis, ligamentous laxity, and/or open fontanelles are considered relative contraindications to HGT.
A head computed tomography (CT) or skull radiograph can be performed prior to halo placement, although this is not the standard practice at the authors’ institution unless there are specific concerns. Approximately 50% of respondents in a survey of spinal deformity surgeons obtain pre–halo placement imaging of the skull. 14 One must be acutely aware of prior surgery, especially the placement of ventriculoperitoneal (VP) shunts, as fracturing of these devices has been reported in HGT. 15
18.2.2 Equipment
Halo Application
At our institution, we do not typically obtain studies prior to halo placement for evaluation of the skull. However, if the patient has undergone any cranial surgery (VP shunt), has poor bone density (osteogenesis imperfecta), or is very young (potentially open fontanelles), a head CT scan or skull radiographs should be obtained prior to halo placement. 16
The most common determining factor for the number of pins used in HGT is the patient’s age, followed by underlying diagnosis. Typically, in children younger than 8 years, four anterior pins are used, and for children 8 years and older, two anterior pins are used. Similarly, six posterior pins are utilized for children younger than 5 years, four to six pins for children 6 to 8 years of age, and four pins for children older than 8 years. In patients who have reached skeletal maturity, a total of four pins (two anterior and two posterior) may be sufficient.
The halo is applied under conscious sedation with local anesthesia in the operating room or procedure room. The halo is placed slightly below the equator of the skull, but above the orbits and superior aspect of the pinnae. 12 The ideal pin torque is also based on patient age and underlying diagnosis. We recommend the use of an adjustable torque wrench during halo placement starting at 2 inch-pounds (in-lb) and progressing in 1 to 2 in-lb increments. A goal of at least 4 in-lb is ideal. The greater the skull density, the more torque may be applied, to a maximum of 8 in-lb. If only a lower amount of torque is tolerated, the surgeon must then place additional pins to distribute the forces. The surgeon must be aware of avoiding the medial half of the orbits anteriorly so as not to injure the supraorbital nerve. Furthermore, avoiding pin placement in the temporal fossa is critical as this can injure the temporalis muscle, and the bone of this portion of the skull is relatively thin 16 , 17 , 18 (Fig. 18‑2).

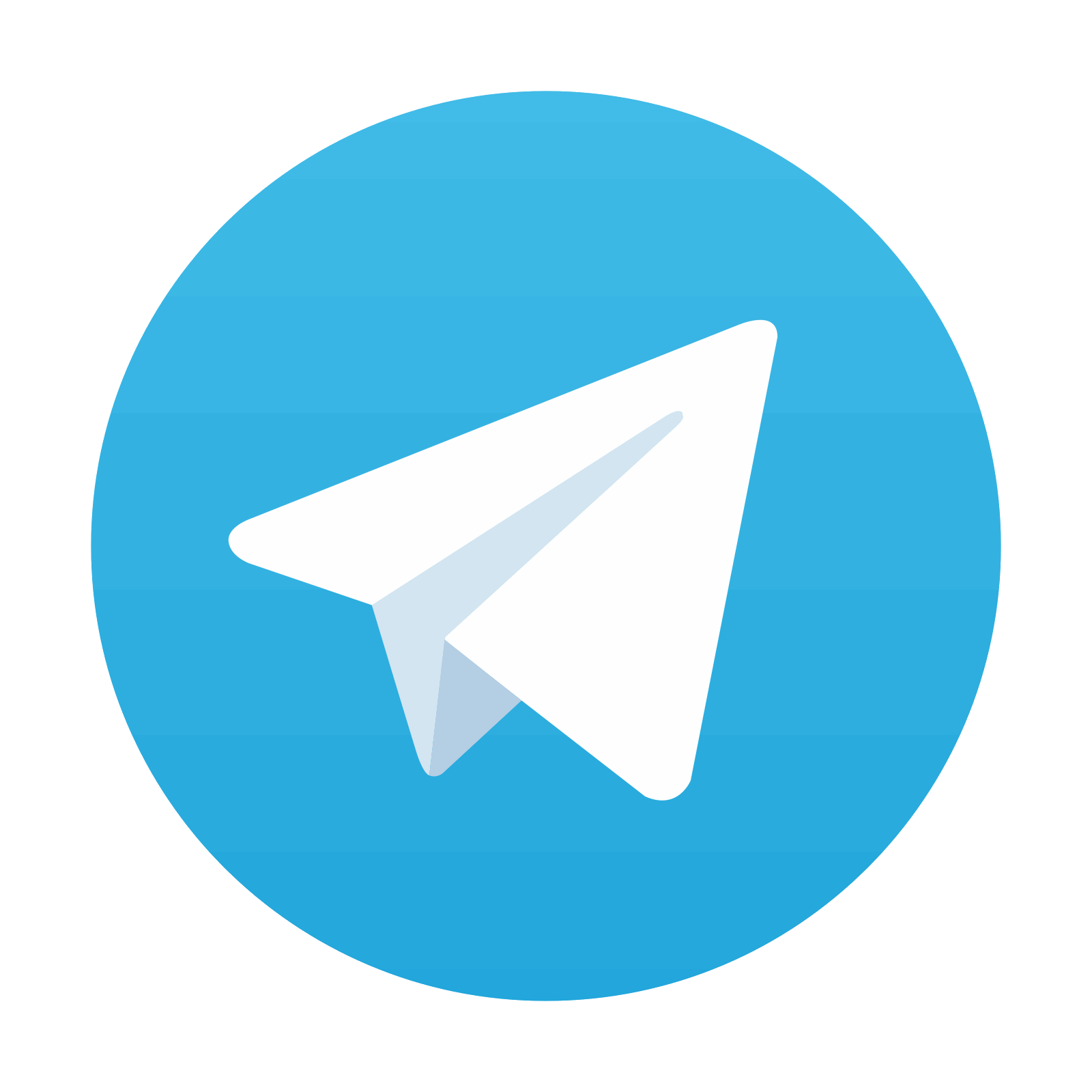
Stay updated, free articles. Join our Telegram channel

Full access? Get Clinical Tree
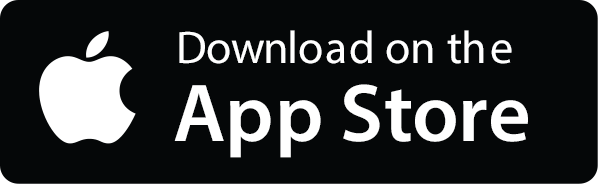
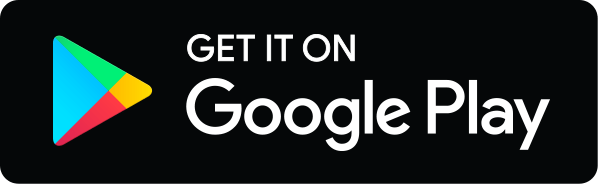
