19 Endoscopic third ventriculostomy Indications, technique, outcome, and complications
Adult Hydrocephalus, ed. Daniele Rigamonti. Published by Cambridge University Press. © Cambridge University Press 2014.
Indications and outcome
Although the first endoscopic third ventriculostomy (ETV) was performed by Mixter back in 1923 [1], ETV has only become established over the past 20 years as the preferred therapeutic option for obstructive hydrocephalus. This is largely the result of improved endoscopic optics, cold light fountains, and miniaturized CCD cameras [2–10].
Overall ETV success rates range from 33% to 95% and are excellent in both (1) obstructive hydrocephalus caused by aqueductal stenosis (>75%) and (2) third ventricle outflow obstructions caused by benign space-occupying lesions (95%) [2,4–15]. O’Brien and coworkers also noted that primary ETV was highly successful in treating hydrocephalus related to spina bifida (88%), aqueductal stenosis (69%), and arachnoid cysts (100%). In a secondary ETV group (after shunt malfunction), the highest success rates were described for arachnoid cysts (100%) followed by aqueductal stenosis (68%) and spina bifida (55%) [16]. The procedure is also successful in treating tetraventricular hydrocephalus [13].
ETV has also been used in communicating hydrocephalus with conflicting results. Though it may be an effective treatment in certain patients [17], ETV success rates appear substantially lower in communicating than occlusive hydrocephalus.
Studies that have assessed nonobstructive hydrocephalic patients with a history of hemorrhage and/or infection have reported ETV success rates ranging from 0 to 100% [8,9,17–20]. In general, however, poor primary ETV outcomes were observed in patients with intraventricular hemorrhage (IVH)-induced hydrocephalus and hemorrhage and infection-induced hydrocephalus [16,20].
The discussion concerning factors that influence ETV success rate is ongoing. Factors that might affect ETV outcome include the type of hydrocephalus, age of the patient at the time of intervention, previous shunt placement, previous brain radiation, intraventricular tumor, and accompanying cerebrospinal fluid (CSF) infections. Dlouhy et al. demonstrated the predictive value of preoperative ventricular bowing for ETV success, but 33% of patients without bowing were also successfully treated with ETV [21]. The bowing may be absent due to a decreased ventricular compliance, e.g. in slit ventricle syndrome.
Moreover, relevant anatomical structures for the ETV approach and their maintained integrity following ETV, such as massa intermedia, mammillary bodies, basilar artery, clivus, and the extent of Liliequist’s membrane, also play an important role in outcome estimation [11].
ETV in adult patients
ETV is an effective treatment option for adult patients with noncommunicating hydrocephalus. Dusick et al. demonstrated an overall long-term shunt independence in 79% of 108 patients with idiopathic aqueductal stenosis, mass lesions causing noncommunicating hydrocephalus, and other miscellaneous obstructive etiologies [2]. These findings corroborate success rates reported in other studies with adult patient subsets – ranging from 66% to 88% [6,22–25]. A recent summary by Sacko et al. reported an overall success rate of 77% in adults with a higher failure risk in hemorrhage-related and idiopathic chronic hydrocephalus than in other types of hydrocephalus [26] (Table 19.1).
Table 19.1 Reported indications for ETV
ETV in children and the role of age and etiology dependence
The roles that (1) age at the time of treatment and (2) etiology of the hydrocephalus play in ETV outcome in children are currently being discussed [8–10,12,27–29]. The following pathophysiological explanations have been offered in light of higher ETV failure rates in children.
The immaturity of arachnoid granulations leads to decreased CSF absorption in newborns, and the open fontanelle with splayed sutures prevents an increase of intracranial pressure of more than 5 mmHg, which would be necessary for a sufficient absorption through the sagittal sinus [30–34]. Cinalli et al. showed that long-term ETV outcomes were the same across children younger and older than 6 months of age, and postulated that this procedure is not contraindicated in this patient group. Furthermore, repeated ETV is a useful management technique for a delayed failure in cases of stoma obstruction [12]. In a recent retrospective study, Costa Val et al. noted that the effectiveness of ETV is more likely due to hydrocephalus etiology rather than age. They found that ETV treatment of aqueductal stenosis had a 90% success rate in comparison with 50% for Chiari-II-related hydrocephalus [35].
On the other hand, many studies have assessed ETV failure rates in very young infants compared with children older than 6–12 months [5,8,10,29,36–41]. Patients under 6 months of age had a 5-fold increase in risk of ETV failure compared with older patients [26]. Beems and Grotenhuis favored the role of etiology for estimating ETV success in children younger than 2 years of age. Though they found an overall success rate of 53% in 66 children younger than 2 years, success varied across etiologies, ranging from 20% to 88% [10]. Siomin et al. found that ETV was not beneficial in premature newborns and patients with IVH-induced hydrocephalus. However, in this multicenter study, the authors reported that secondary ETV following shunt malfunction had a high rate of success in patients harboring a triventricular hydrocephalus [20].
Kombogiorgas and Sgouros reported an overall ETV success rate of 42% in a series of 33 hydrocephalic children with a mean age of 76 months. They concluded that ETV may not be suitable for patients with a complicated shunt history due to previous CSF or shunt infection. CSF leakage following ETV in patients without previous shunt implantation or in children under 2 years of age was strongly associated with ETV failure [42].
The ETV success score (ETVSS) attempts to predict ETV success and failure in the treatment of childhood hydrocephalus by taking age, cause of hydrocephalus, and history of previous shunt placement into account [43].
Warf concluded after a series of 300 consecutive hydrocephalic children (81.3% younger than 1 year of age), usually caused by CSF infection, that ETV can be performed with acceptable results in a developing country in spite of the difficulties regarding shunt dependence [44].
ETV in previously shunted patients
Results pertaining to ETV efficacy in previously shunted patients are conflicting. On the one hand, Hopf et al. reported a success rate of 84% in previously shunted patients, a finding that several studies have corroborated [6,45].
Teo and Jones showed a statistically significant success rate of ETV for previously shunted patients with myelomeningocele (84%) in comparison with patients without previous shunt implantation (29%) [46].
On the other hand, the failure rates of ETV across previously shunted and non-shunted patients were 20.8% and 20.5% in a series of 63 adult patients. The authors pointed out that the history of three or more shunt revisions was a statistically significant risk factor for ETV failure [15]. Kombogiorgas and Sgouros concluded that ETV may not be suitable in young pediatric patients with a complicated shunt history due to previous CSF or shunt infection [42].
However, studies by O’Brien et al., Cinalli et al., and Mallucci et al. have reported success following secondary ETV in obstructive hydrocephalus after IVH who present with ventriculoperitoneal (VP) shunt malfunction [16,47,48]. Furthermore, O’Brien et al. reported a high ETV success rate in patients with infection-induced obstructive hydrocephalus [16].
ETV in communicating hydrocephalus
ETV has been reported to be an effective treatment for communicating hydrocephalus in certain patients [17]. The precise underlying mechanisms of communicating hydrocephalus remain unknown. Ransohoff et al. suggested a nomenclature with “intraventricular obstructive hydrocephalus” including obstruction of aqueduct and outlet foramina of the fourth ventricle, and “extraventricular obstructive hydrocephalus” [30]. Recently, Rekate investigated the basal cisterns as a source of CSF flow blockage and suggested that all hydrocephalus is to some extent obstructive. Thus, the modified bulk flow theory might explain ETV’s effectiveness in certain types of communicating hydrocephalus [17,49].
The low primary ETV success rate and encouraging secondary ETV results following shunt failure in patients with hemorrhage and/or infection were described as follows. Initially, a communicating hydrocephalus due to the debris in the subarachnoid space and arachnoid granulations occurs and shunting allows for the re-expansion and normalization of the subarachnoid spaces. Aqueductal stenosis may then be acquired during the following course. This seems to explain ETV’s usefulness at a later period and the high failure rate in the initial phase. Thus, the endoscopic approach might be an alternative to be discussed in patients after shunt malfunction and infection [16].
ETV in idiopathic normal pressure hydrocephalus
The first reported patient series using ETV to treat idiopathic normal pressure hydrocephalus (iNPH) was performed by Mitchell and Mathew, and Meier and coworkers [50–52].
Mitchell and Mathew reported improvement in three out of four cases after performing ETV, and suggested that the relief of periventricular tissue stress improved local blood flow.
Meier et al. compared 15 patients who underwent ETV with 60 patients who were shunted and observed that though immediate results were similar, the ETV patients had better long-term outcomes. They additionally suggested that ETV might be particularly beneficial in those patients with increased resistance following the ventricular infusion test but normal lumbar infusion test results. This indicates that obviously patients with both communicating and noncommunicating hydrocephalus have been included in this study. Gangemi et al. found an overall rate of neurological improvement of 72% following ETV in a series of 25 patients with clinical preoperative history of NPH of up to one year [53]. The authors concluded that ETV and shunt implantation offer similar results in patients with iNPH who present with a short duration of symptoms, prevalence of gait disturbance, and slight mental impairment. Furthermore, they suggested that these results indirectly confirm that CSF absorption is at least sufficient in these selected patients. Typical complications of shunting, like subdural hematoma and effusion, are significantly diminished after ETV due to only slight decrease in intracranial pressure (ICP) after ETV.
The following pathophysiological explanations (hydrodynamic concept) may play a role when choosing ETV as a therapeutic option in iNPH.
Communicating hydrocephalus is caused by decreased intracranial compliance which increases systolic pressure and fluid transmission into the brain parenchyma rather than CSF absorption. The increased systolic pressure in the brain distends the brain towards the skull and compresses the periventricular region against the ventricles. These factors lead to a predominant enlargement of the ventricles and narrowing of the subarachnoid space [54]. The parenchyma is chronically damaged due to continuous systolic pulsations and periventricular edema, leading to a decrease of cerebral blood flow. Thus, communicating hydrocephalus is a disorder of intracranial pulsations caused by decreased compliance [55,56]. ETV results in a reduction of the increased systolic pressure by venting ventricular CSF through a stoma, leading to an improvement of the cerebral blood flow and perfusion pressure and abolition of transependymal resorption, whereas the patent aqueduct is too narrow to sufficiently vent the CSF [53,54,57].
Longatti et al. suggested possible factors that could explain good outcomes in 3 of 14 patients with NPH, namely, a partial fourth ventricle outlet obstruction, and a functionally compressed aqueduct [58]. Therefore, a full exploration of the fourth ventricle with flexible endoscopes is suggested to exclude unexpected blockages of the outlet not assessed in MR studies. In addition, the authors postulated the mechanism that intermittent aqueductal squeezing may occur because it is easier for the brainstem to slip upwards through the tentorial notch than the cerebellum thereby leading to a functionally constricted aqueduct. Particular attention should be paid to any slight deformation of the third ventricle floor suggestive of a functional aqueductal stenosis [58].
Hailong and coworkers have shown an overall proportion of 65.6% of excellent/good (≥50% clinical improvement) clinical outcomes after ETV in 32 patients (including 17 patients with iNPH). The authors found a high correlation of Kiefer scores and patient age with favorable outcomes, and determined that the preoperative mental state plays an important role in the prediction of outcome after ETV [17]. Gangemi et al. [59] retrospectively reviewed 110 patients with idiopathic normal pressure hydrocephalus who underwent ETV in four Italian neurosurgical centers. Clinical improvement occurred in 69.1% of patients after 2 years. The authors found no correlation between success rate and patient age, or type of ventricular enlargement (normal or enlarged fourth ventricle). Conversely, the rate of neurological improvement was higher in patients with shorter clinical history, better preoperative neurological score, and clinical onset with gait disturbances. Moreover, sudden intraoperative reappearance of normal cerebral pulsations and significant downward and upward movements of the third ventricular floor after ETV were also correlated with good outcome [59]. A systematic review has been planned to evaluate the possible evidence of ETV in iNPH [60].
In summary, the published series show inconsistent clinical results after ETV for iNPH. In our experience, ETV for iNPH is not successful in the long term. A short-lasting effect is seen similar to the effect after lumbar puncture. It is our impression that the good results of most studies are due to the fact that not only true iNPH was included but also forms of obstructed hydrocephalus with a patent aqueduct which resembled iNPH, for example the extraventricular intracisternal obstructive hydrocephalus described by Kehler and Gliemroth [61]. The hypothesis of (distal and proximal) intracisternal obstructive hydrocephalus may be helpful in patient selection for ETV in cases of so-called “communicating hydrocephalus.” The authors suggested an indication for ETV in cases of proximal intracisternal obstruction with documented downward bulging of the third ventricle floor in MR images [61]. Based on our results, we do not recommend ETV for the treatment of iNPH.
Intraoperative factors influencing the success of ETV
Intraoperative factors, such as hemorrhage, size of stoma, thickness of the third ventricular floor [62], and flow through the stoma, may play a role in ETV success rates. Some studies have found a correlation between success rate and presence of prepontine adhesions, thickened floor of the third ventricle, and small size of stoma in children. Kombogiorgas and Sgouros, however, did not find such a correlation. They evaluated a stoma diameter of over 30% of the distance between posterior clinoid and basilar artery as a possible association with success of ETV in children without previous shunting but pointed out the possible increased risk of basilar artery injury during the creation of a very large stoma [34]. Floating tissue has to be carefully coagulated or resected to avoid a stoma obstruction [7].
Factors leading to the obstruction of the ETV stoma are important for the ETV failure rate: gliotic tissue or arachnoid membranes have been described [7,11,15,63]. Liliequist’s membrane has to be opened as it acts as a barrier of supra- and infratentorial cisterns and may cause a failure of ETV [63,64]. Intraoperative ventriculography may help to evaluate the patency of the stoma and of the intracisternal CSF pathways [65].
Definition of success after ETV and diagnosis of ETV failure
Shunt independence has often been cited as a criterion for the success of an ETV [2,7,14,66]. However, symptomatic improvement has been considered the main criterion. Therefore, the diagnosis of ETV failure is primarily based on clinical and not radiological aspects. It is difficult to define success, for instance, in patients with only minimal clinical improvement after the endoscopic procedure. In many cases, patients present with vague symptoms of possible recurrent hydrocephalus such as headache without specific clinical signs indicating a raised intracranial pressure [14].
The volumes of the third and lateral ventricles are usually reduced after ETV, but up to 20% of patients (especially those with longstanding ventriculomegaly) show little or no changes in ventricular size despite good outcomes [67,68]. The reduction of ventricular volume is much less evident than in imaging following shunt placement. Especially in very young children, ETV rarely results in a major reduction of the ventricles [16].
MRI studies may clearly show the stoma and the flow through the stoma. However, they do not predict the clinical success, as patients may have a combination of obstructive and communicating hydrocephalus. This means that despite a patent stoma with a good flow void the procedure may fail [4]. Furthermore, patients with subtle symptoms and chronic ventriculomegaly may not experience dramatic clinical improvements, or improvements may be delayed [2].
Possible causes of an ETV failure are (re-)obstruction of the stoma due to hemorrhage, debris or scar tissue, not opened membranes within the perimesencephalic cisternal space, decreased absorption due to infection, hemorrhage, or longstanding VP shunt history.
The time of ETV failure is unpredictable and may occur days to years after the operation, leading even to an abrupt and life-threatening deterioration [2,12,14,69].
Management of ETV failure
If the primary ETV fails to improve the patient’s symptoms, the patency of the stoma has to be checked. If MR imaging shows that the stoma is obstructed, a redo ETV should be performed if during the first surgery no negative predictors such as severe arachnoid scarring of the prepontine cistern were observed. The chance of a successful outcome after a re-ETV in our series was 80% [70]. If the stoma is patent and MR imaging shows a flow void signal, the hydrocephalus is obviously not only obstructive but has a CSF resorption component. Then, shunting is usually necessary to treat the hydrocephalus successfully. Our experience corresponds with reports in the literature. In a series of 20 patients, a re-ETV was shown to be safe with a success rate of 65% [71]. Additionally, Koch et al. concluded that ETV has to be considered as a sufficient treatment option after an initial successful ETV in cases of later stoma occlusion [72].
Advantages/disadvantages of ETV compared to VP shunt placement
The main advantage of ETV is that no foreign material has to be inserted. This decreases the risks of infection and malfunction – namely, obstruction or disconnection. Studies confirm that the risk of infection following a neuroendoscopic operation is lower than with shunting (approximately 0–2% vs. 5–10%) [16,73,74]. ETV’s main disadvantage is that it is not effective in most types of communicating hydrocephalus. Unfortunately, no tests are currently available to accurately predict the success of an ETV. Shunt placement is an effective therapy for obstructive and communicating hydrocephalus in all age groups, but carries the risk of infection and/or malfunction of 25–40% in the first year after shunt placement and the risk remains at 4–5% each year thereafter [16,74–76]. Long-term data show a revision rate of 81% in 12 years [77]. Triventricular hydrocephalus treatment with ETV has been shown to have a success rate of 72% over 6 years [12]. Other types of hydrocephalus treated with ETV are reported to have less successful outcomes [19,47]. Slit ventricles and diminished reabsorption due to peritoneal adhesions may present difficulties for shunted patients but do not pose any problems for ETV patients.
Preoperative planning and anatomic eligibility for ETV
ETV success and complication avoidance during/following the operative procedure depends on precise preoperative planning, and is usually based on MR imaging. Certain aspects have to be considered when planning an ETV (see Table 19.2).
Table 19.2 MR findings to be considered preoperatively
First, the ventricular size must allow handling of the endoscope without an increased risk to the surrounding structures. Furthermore, the width of the foramen of Monro must be estimated to allow the passage of the endoscope. Because it is the bottleneck of the approach to the third ventricle, the foramen will essentially influence the choice of endoscope used in the procedure. An endoscope with a diameter larger than the foramen of Monro may cause damage to surrounding anatomical structures like the fornix, veins, and choroid plexus. Smaller endoscopes are recommended particularly for newborns and young children. If narrow, it is useful to view the foramina of Monro on coronal and sagittal MR images to determine the optimal approach [16].
Furthermore, the perimesencephalic cisternal anatomy should be carefully evaluated. Patients in whom the brainstem and distal basilar artery were judged to be too close to the clivus (in general 2 mm space) have not been considered to be eligible for an ETV [2]. On the other hand, however, the prepontine space will be widened due to the normalization of the supratentorial pressure after drainage of the CSF. We agree with O’Brien et al. who suggested that there is rarely a contraindication to perform an ETV [16].
Navigation systems help to plan the trajectory in patients without significantly enlarged ventricles. Moreover, navigation is helpful if anatomic landmarks on the floor of the third ventricle are distorted – a situation that could lead to decreased or absent orientation. Chen and Nakaji have suggested after trajectory views of 53 patients that the optimal entry point for ETV is 30.1 ± 7 mm perpendicular to the midline and 8.9 ± 14.1 mm behind the coronal suture. Furthermore, they stated that the entry point is highly variable and image guidance should be used in all patients whenever available [78].
Kehler has introduced a grading system to classify eligible patients for ETV on a 5-point scale: (1) presence of imaging evidence of obstruction, (2) degree of bulging of the third ventricular floor, and (3) the presence of progression in preoperative symptoms within the 12 months preceding the ETV. This standardized grading system predicts the outcome of ETV and helps in decision-making for ETV versus VP shunting [79]. Another grading system, the ETV success score (ETVSS), includes age, cause of hydrocephalus, and history of previous shunt placement to model ETV success and failure in the treatment of childhood hydrocephalus [43]. However, to date, no test can precisely predict the success in an individual patient.
An externalization of the shunt 48 to 72 hours before the operation under strict ICP monitoring can help to enlarge the ventricles in patients with shunt-related slit ventricles [80].
MRI: flow dynamics measurement
CSF flow can qualitatively be described as the flow-void signal on T2-weighted images. CSF flow is best appreciated in narrow areas within the ventricular system such as aqueduct or after third ventriculostomy within the stoma site. To evaluate the dynamics of the CSF flow, the technique of cardiac-gated cine phase-contrast MR imaging has been increasingly used over the past 15 years. This extremely sensitive imaging technique allows the visualization and quantification of CSF flow noninvasively, even in slow flow and, therefore, allows considerable information on the physiology of the normal CSF circulation. Furthermore, information about flow disturbances is possible in certain pathological circumstances: communicating and obstructive hydrocephalus, Chiari malformation, cystic lesions, and cervical stenosis. Cine MR imaging has been recommended for evaluating third ventriculostomy patency [81–83]. For this measurement, technical considerations include: a high field MR unit (1.5T or greater) with a circular polarized head-array coil and a gradient system capable of acquiring standard axial (5 mm slice thickness) T1-weighted images. CSF flow can be visualized with flow sensitive T2-weighted images, e.g. T2-weighted turbo inversion-recovery spin-echo sequence (TE 4300 ms, TE 60 ms, and 2 mm slice thickness). For phase-contrast MR imaging, a two-dimensional fast low-angle shot (FLASH) T1-sequence can be used. Velocity encoding should be set at 6–10 cm/s. The imaging plane should be placed in the middle of the ventriculostomy or the aqueduct and slice thickness should be 4 mm. For flow quantification, the axial plane with through-plane velocity encoding in the craniocaudal direction, and for qualitative assessment, the sagittal plane with in-plane velocity encoding in the craniocaudal direction can be used [84].
Surgical technique of ETV, avoidance of complications
We use the LOTTA® (Karl Storz GmbH & Co. KG, Tuttlingen, Germany) rod-lens ventriculoscopic system which includes a larger (Standard LOTTA) and a smaller (Little LOTTA) ventriculoscope (Figures 19.1 and 19.2) [85]. For ETV, we usually use the smaller scope (diameter 3.6 mm). We prefer rigid rod-lens scopes with HD cameras because of the excellent image quality and ease of guidance. Flexible scopes are rarely used. The endoscope can either be guided “freehand” or fixed using a holding device (e.g. Point Setter pneumatic arm, Mitaka Co., Tokyo, Japan), which is, in our opinion, preferable.
Figure 19.1 (a) LOTTA ventriculoscope, sheath and trocar. (b) Tip of the endoscope including lens, working and irrigation channels.
Figure 19.2 (a) Endoscope fixed with the pneumatic holding arm allowing a bimanual manipulation of the instruments. (b) Bimanual dissection with two instruments inserted through the main working channel (middle) and one side channel that alternatively can be used for irrigation.
We always administer antibiotic prophylaxis (cefuroxime) as a single shot intravenous medication around 30 minutes before skin incision. The head of the supine positioned patient should be anteflexed 30° to avoid CSF loss. The burr hole is placed in front of the coronal suture and about 2.5 cm paramedian [86]. Usually, we place the entry point on the right side. If the right foramen of Monro or the right ventricle is significantly smaller than on the left side, we make the approach on the left side. Depending on the need for further procedures (e.g. tumor biopsy, inspection of the aqueduct, septostomy), the burr hole must be placed more anteriorly, posteriorly, or laterally. It is essential to plan the trajectory before the skin incision. In most cases of hydrocephalus, the ventricles are clearly dilated and allow an uneventful puncture of the frontal horn.
We only use navigation in patients in whom we expect anatomical variations which make orientation difficult, such as ventriculitis, or to approach narrow ventricles.
After having placed the burr hole, the dura is coagulated and incised. The cortex is coagulated and the ventricle punctured bluntly using a Cushing needle to confirm the trajectory. A small amount of CSF is taken for laboratory examination. The endoscopic sheath is introduced with the aid of the trocar into the frontal horn. Usually, the frontal horn is reached at a depth of 4–5 cm. Then, the trocar is removed and the endoscope introduced. Excessively deep introduction of the endoscope or endoscopic sheath into the lateral ventricle may cause thalamic contusion or midbrain injury. Tiny hemorrhages always disappear within minutes with continuous irrigation.
The choroid plexus, the veins, and the foramen of Monro are then identified and the endoscope is carefully advanced through the foramen into the third ventricle. The fornix structures should not be touched. Therefore, the endoscope is moved on the top of the plexus and the plexus is gently pressed down if the interventricular foramen is narrow. It is also essential to see and control the edges of the endoscopic sheath (endoscope pulled back into the trocar) during movements within the ventricle, especially when passing the foramen of Monro. Limitations of a very narrow foramen are obvious. Once the trajectory to the floor of the third ventricle is not optimal, maneuvering the device within the foramen is difficult and may cause an injury to the fornix.
The mammillary body and the infundibular recess are identified. The typical point for fenestration of the third ventricular floor is the midpoint of a triangle formed by the mammillary bodies and the infundibular recess, just posterior to the infundibular blush. Usually, this point is halfway between mammillary bodies and infundibular recess, just behind the clivus. However, the anatomic landmarks may be distorted. Sometimes, the floor of the third ventricle is thick and non-translucent, e.g. in the event of acute hydrocephalus or after chronic inflammation. If the basilar artery is not seen through the floor, the position of the basilar artery and the distance to the mammillary bodies and clivus is obtained from MR imaging. We prefer to perforate the floor bluntly using a closed “Decq” forceps. During this step, the basilar artery and perforators are at the greatest risk for injury and, therefore, we do not advise fenestrating nearer to the mammillary bodies than the triangle midpoint described above. The perforation is then enlarged by inflating the balloon of a 3-French-Fogarty catheter. The catheter should be inflated with saline instead of air to prevent the “pop-up” phenomenon and assure a slow and constant enlargement of the balloon. Usually, pulsation of the floor can be observed after the fenestration has been achieved. The premesencephalic and prepontine cisternal space has to be inspected to confirm that the subarachnoid space has been reached and a patent premesencephalic and prepontine subarachnoid space is present. The basilar artery should be seen (Figure 19.3a–e). If there are arachnoid membranes which might interfere with CSF flow, such as Liliequist’s membrane, these membranes have to be opened too. Sometimes, several membranes have to be cut with scissors. To enhance the safety of this procedure, the endoscopic sheath of the Little LOTTA scope is inserted through the ventriculostomy into the prepontine cistern. Then, under good visual control, the membranes are cut with the sharp-pointed scissors. If intraoperative hemorrhage occurs, it will be important to maintain the position of the endoscope and to irrigate (lactated Ringer’s solution at 37°C) to improve the visualization. Especially in difficult procedures where long irrigation and substitution of the CSF is needed, this fact is noticeable, because the alternative use of saline solution may lead to prolonged awakening after surgery, hyperventilation, and transient confusion of the patient as observed by the authors. Rarely, the use of the bipolar rod or forceps is necessary. Usually, minor hemorrhages stop after a few minutes of irrigation. Mild bleeds of the rim of the fenestrated membrane may be controlled simply by leaving the dilated Fogarty balloon within the stoma for a minute for compression. While irrigating, the surgeon has to make sure that the outflow canal for the irrigation fluid is not closed to avoid a critical increase of ICP. Additionally, air bubbles causing a diminished view have to be avoided during the irrigation.
Figure 19.3 Endoscopic third ventriculostomy. (a) View through the foramen of Monro (CP, choroid plexus; F, fornix). (b) Floor of the third ventricle (BA, basilar artery; M, mammillary bodies; C, clivus; IR, infundibular recess). (c) Blunt perforation of the floor of the third ventricle with the Decq forceps. (d) Dilation of the stoma by inflating the balloon of the Fogarty catheter. (e) Sufficient open stoma after ETV. (f) Inspection of the prepontine cistern showing the basilar artery.
After a sufficient ETV has been achieved, the endoscope is withdrawn from the third ventricle into the lateral ventricle and the foramen is inspected. While the endoscope is removed from the brain, the cortical puncture channel is visualized for potential active bleeding. In most cases, the dura is not closed. We simply place a piece of Gelfoam® in the burr hole. The galea is sutured and the skin is closed with an atraumatic running suture. In premature newborns and newborns, we try to achieve a water-tight dural closure because of the higher risk of CSF fistula. We suture the dura, if possible the periosteum too, and place a piece of Tachosil® (Nycomed, Konstanz, Germany) over the suture line. We particularly mention not to use autologous bone dust to close the bony defect. For cosmetic reasons, a titanium burr hole plate can be used to cover the burr hole.
Usually, we do not place a reservoir after having performed an ETV. However, Aquilina and coworkers recommend the routine placement of a ventricular reservoir after ETV due to the fact of unpredictable timing of a possible failure and its unexpected, abrupt, and life-threatening course [73]. It allows emergency therapeutic CSF aspiration which assists in the diagnosis of ETV failure, and intrathecal drug administration without an increased operative risk. For cosmetic reasons, a circular depression in the outer table of the cranium can be made to countersink the burr hole reservoir [73].
Reported complications
The rate of complications varies between 0% and 31.2% with an overall complication rate of 8.5% according to a literature review by Bouras and Sgouros [87]. The technique as described in detail within this chapter covers different intraoperative steps that have to be completed very carefully to avoid damage to the intra- and paraventricular structures.
Herniation syndromes following increased ICP due to a closed endoscope outflow channel have been observed and result in a transient decrease of consciousness and permanent confusion. Although unusual, it has also been reported that in a patient with very narrow foramina of Monro who underwent ETV because of hydrocephalus related to aqueductal stenosis, bilateral occlusion of the foramina of Monro had occurred 30 months after ETV [88].
Inflating the balloon within the ambient cistern and then pulling the balloon through the tuber cinereum may risk incorporating a branch of the posterior circulation [89]. Major subarachnoid hemorrhages due to basilar artery injuries and its branches may be underreported, but happen and frequently lead to death [90]. Apart from vascular injuries, transient and permanent damage to the oculomotor nerve has been reported [91].
Furthermore, hormonal disturbances following ETV are closely related to hypothalamic injuries. It is possible that an eccentric stoma creation is responsible for the traumatization of the hypothalamus [87]. Transient diabetes insipidus has been frequently observed [23,24,91]. Also, abnormalities of prolactin levels, postoperative hyperkalemia, and inappropriate secretion of antidiuretic hormone after ETV have been reported [92–94].
During the postoperative period, CSF leaks may occur due to inappropriate skin closure or increased intracranial pressure in 1.61% [87]. Occasionally, it is an early sign of a failure of ETV. Infectious complications such as meningitis or ventriculitis are seen rarely but have to be treated with antibiotics [87,91].
Although subarachnoid hemorrhage after ETV has already been mentioned as a deadly complication, due to injury of the basilar artery or its branches, other intracranial hemorrhages such as intraventricular, subdural, or epidural hematomas can also be found. Subdural hematomas may develop because of a decrease of the intracranial pressure after ETV related to the CSF drainage during the procedure itself or as a result of tiny cortical bleeds into the subdural space due to the corticotomy [89,95,96].
Sudden late deterioration is a rare but probably underestimated complication in patients after ETV. The largest series was reported by Drake et al. and included 16 children with a mean age of 7.6 years [97]. The time to ETV failure ranged from 5 weeks to 7.8 years. Thirteen patients died due to a closed stoma as verified at the autopsy. We concur with other colleagues that the mechanism of a closure of a stoma is likely influenced by multiple factors such as a poor fenestration of the floor of the third ventricle, prepontine remnants of arachnoid membranes, or CSF absorptive problems by arachnoid granulations especially seen in cases of infection or hemorrhage [89]. Bone dust used for filling the burr hole may migrate via the cortical channel through the ventricles and occlude the aqueduct or stoma resulting in an acute increase in ICP [98].
Postoperative care and follow-up
Most failures occur early, but long-term follow-up is vital as mentioned above. In our institution, all patients receive MR imaging with flow studies 1 day postoperatively to assess the patency of the stoma (Figure 19.4). Measurement of ICP during the immediate postoperative course can be performed but is not standard in our ETV protocol [99,100].
Figure 19.4 MR images show a typical patient with hydrocephalus due to aqueductal stenosis. (a, CISS sequence) and (b, IRTSE) demonstrate the preoperative state with enlargement of the lateral and third ventricle with a typical bulging of the floor of the third ventricle (arrow, image a) and the widened prestenotic aqueduct without a flow within the lumen (arrow, image b). Postoperative images after successful ETV reveal a decrease of the size of the ventricles and the elevated floor of the third ventricle with the fenestration site (arrow, image c). The typical flow void can be seen within the stoma between the third ventricle and the prepontine cistern (arrow, image d).
Usually, we recommend a clinical examination 10 to 14 days after discharge. The next follow-up examination including MR imaging with flow studies is performed 3 months after surgery and then annually with an uneventful course.
Summary
ETV has been established as the therapy of choice for obstructive hydrocephalus and is considered to be straightforward and safe. At this point, there is insufficient evidence to suggest that ETV is also effective in communicating hydrocephalus. Because our experience with about 300 ETVs showed that ETV frequently failed as a treatment for communicating hydrocephalus, we do not recommend ETV in these cases. The preferred initial treatment of premature hemorrhagic hydrocephalus is still VP shunt placement. The same is true for iNPH.
ETV patients should have follow-up checks similar to shunt patients. They and/or caregivers should be advised that rapid deterioration with the need for emergency admission can occur at any time.
References
















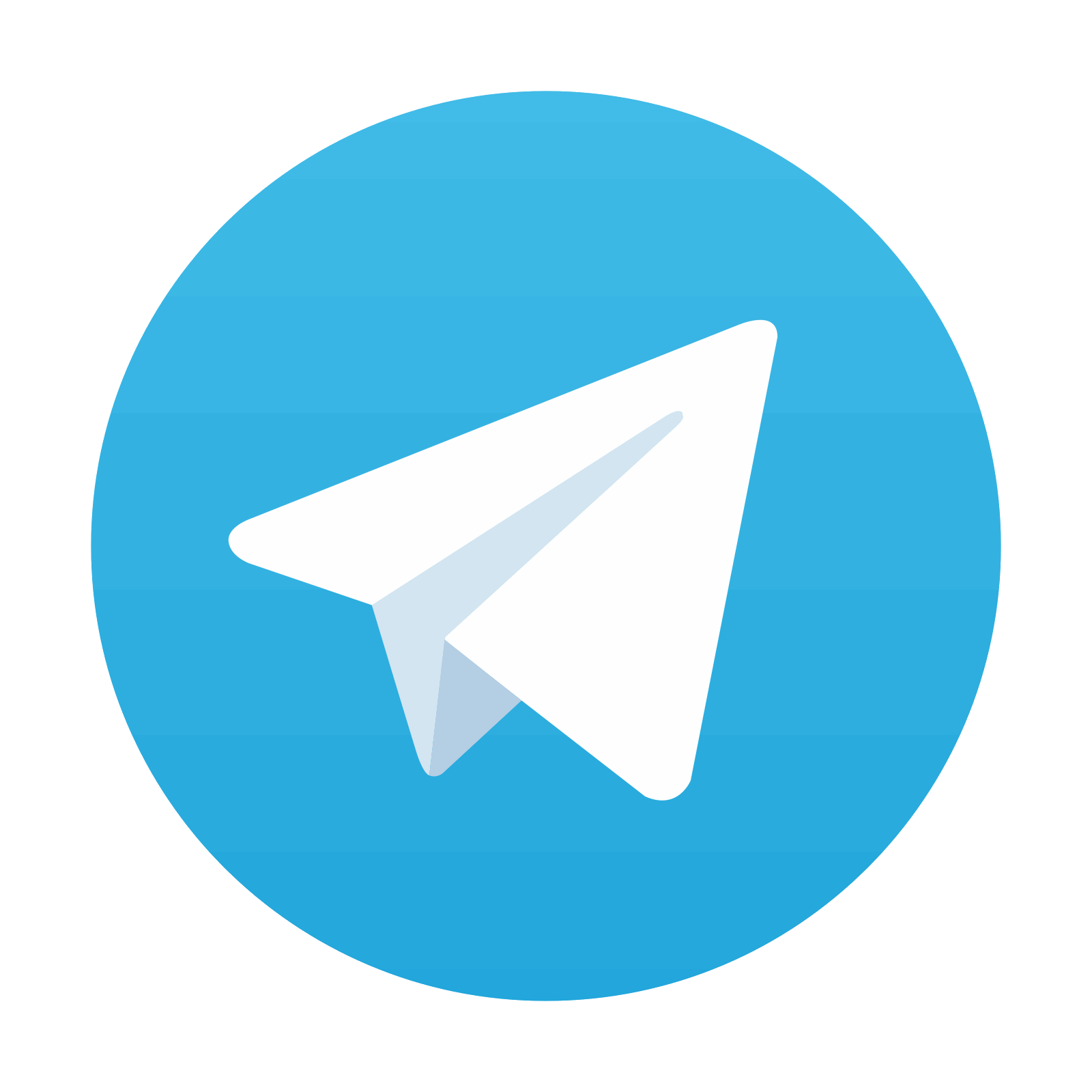
Stay updated, free articles. Join our Telegram channel

Full access? Get Clinical Tree
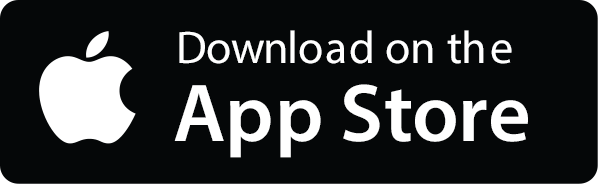
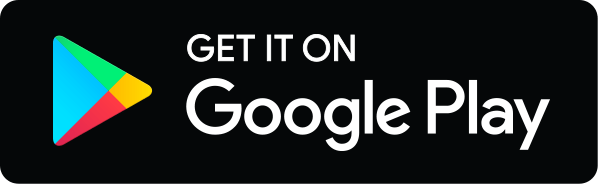
