2 Historical Development of Radiation Modalities for Vestibular Schwannoma
“The time to treat is when the tumor is no bigger than a grain of salt” – Norman Dott1
2.1 Introduction
With earlier diagnosis of vestibular schwannoma (VS) related to the widespread availability of advanced imaging tools such as computed tomography (CT) and magnetic resonance imaging (MRI), the quandary of when and what treatment option to apply remains. Paralleling the advances in earlier detection based on more liberal indications for imaging—asymmetric hearing loss, tinnitus, or equilibrium disorders—the skill set required for surgical removal has advanced. The ability to excise a tumor while sparing existing cranial nerve function improved especially after the introduction of the operating microscope in the 1960s and the 1970s.
During the next 20 years, the consensus management for VS was surgical resection. While results improved with microsurgery, patients continued to be at risk for deafness, facial paralysis, and other cranial neuropathies, in addition to the less common risks of stroke, hemorrhage, and death. Various surgical approaches were refined by neurosurgeons and neurotologists, working alone or as a team, each with commendable rationales—improved facial function with expected deafness via the translabyrinthine approach or improved hearing preservation via the retrosigmoid or middle fossa approaches with somewhat higher risks of facial neuropathy. So what was the rationale and history behind the application of radiation modalities as an alternative to surgical resection?
2.2 The Development of Radiation Modalities
2.2.1 Early Efforts
The pioneering finding that conventional X-rays could detect disorders not apparent on physical examination led to the widespread use of cranial imaging. It was logical to expand the application of radiation to therapeutic needs, especially neoplasia. The energy delivered by orthovoltage X-ray devices quickly led to the finding that the therapeutic window (benefit vs. adverse effects) was relatively narrow. For targets not visible on the surface of the body, radiation delivery to the deeper target also meant that intervening tissues would receive the same dose of radiation. The consideration that faster dividing neoplastic cells would respond more to radiation doses than slow responding normal tissues led to the concept that the target dose needed to be divided into multiple treatment sessions. This so-called fractionation concept was designed to reduce collateral damage but produce preferential neoplastic tissue destruction by repeated hits to the same target.
The safer delivery of radiation to a deep-seated target in the body or skull required the development of higher energy X-ray devices called “linear accelerators.” In addition to fractionation, use of multiple entry ports reduced the potential damage to intervening tissues between the radiation source and the target. In more recent years, the development of linear accelerators equipped with multileaf collimators dramatically improved the delivery of radiation while sparing regional normal tissue. Such devices are often guided by CT or MRI technologies. These developments have greatly improved the therapeutic window for delivery of radiation to deep-seated targets.
2.2.2 Particle Beams
Most radiation devices use gamma rays generated by decay of radioactive sources or X-rays generated by bombarding a tungsten plate with electrons. Alternatively, ionizing radiation can be produced by accelerated charged particles created by cyclotrons. Early pioneers in this effort included Lawrence who worked at the Lawrence Livermore Laboratories at Berkeley, California, in the late 1940s.s. Literatur At that time, patients with pituitary tumors and even those with hormone-sensitive metastatic cancer were treated by pituitary ablation using protons. Work continued at Berkeley with both protons and helium ions in the treatment of both selected tumors and even arteriovenous malformations (AVMs).s. Literatur Similar work was pursued at the Cambridge Cyclotron using protons. Kjellberg by the 1970s had treated a very large number of pituitary and AVM patients with Bragg peak stereotactic radiation done in a single procedure.s. Literatur , s. Literatur Despite relatively rudimentary methods to determine the target location and volume in the pre-CT era, the application of radiation modalities progressed.
2.3 The Early Work of Lars Leksell
Lars Leksell was one of the great pioneering neurosurgeons of the 20th century. Trained as a neurophysiologist and working in the lab of Ragnar Granit, Leksell was the first scientist to describe the gamma motor system for postural motor tone control. This work served as the base of his PhD degree. His neurosurgical education was obtained at the Serafimerlasarettet in Stockholm, Sweden, under the direction of Herbert Olivecrona, the pioneering Scandinavian neurosurgeon in the first half of the 20th century. During his training in the 1930s, Leksell immediately recognized the terrible risks that patients undertook, poor if any anesthesia, brain swelling, stroke, and a high risk of mortality. He was convinced there had to be a better way to access deep regions of the brain more safely.
Leksell completed a fellowship in stereotactic surgery in Philadelphia in 1947, studying with Temple University pioneers Ernest Spiegel and Henry Wycis. By 1949, he had published his first description of an arc-centered stereotactic guiding device.s. Literatur This prototype device was further modified to link an orthovoltage X-ray device to the arc, generating photon beams that could be cross-fired on a cranial target. The term and field of stereotactic radiosurgery (SRS) was borns. Literatur and the first patient with trigeminal neuralgia underwent irradiation of the gasserian ganglion using this prototype device.
Leksell was fascinated with the combination of better brain imaging and guiding devices. During his career, he evaluated conventional radiography, ultrasound, encephalography, CT, and finally MRI. His guiding devices were continually modified to facilitate higher quality imaging with the stereotactic frame in position. After target selection, both open stereotactic procedures and closed skull radiosurgical procedures were possible with the same guiding device.
During the late 1950s and early 1960s, Leksell collaborated with Swedish radiobiologist Börje Larsson to further study whether cross-fired radiation beams could be integrated with his stereotactic guiding devices. In 1951, Leksell published the article in which he described the potential methodology of SRS.s. Literatur Larsson was working at the Gustav Werner Institute in Uppsala, Sweden, where a synchrocyclotron generated proton beams for physics studies. Together they performed an initial series of animal experiments. Goats were the preferred animal because they could thereafter live their natural lives at Larsson’s farm. Following these studies, three patients underwent cross-fired proton radiation (not Bragg peak as performed at the Harvard Cyclotron Unit)s. Literatur , s. Literatur.
The logistics were complex and Leksell and Larsson decided that a smaller more compact 179 source cobalt 60 unit cross-firing gamma rays made more sense. This self-contained technology allowed 4-mm beams to be focused on a small intracranial target. The unit itself could be placed in the local hospital environment. The first prototype Gamma Knife unit was installed in 1968 at the Sophiahemmet Hospital in Stockholm shortly after the first patient was treated in late 1967 just after loading the unit with sources at the nuclear reactor site Studsvik, Sweden. Thus, arrived the world’s first noninvasive neurosurgical operating room. Despite Leksell’s primary interest in functional neurosurgery, especially movement disorders and refractory severe obsessive compulsive and anxiety disorders, the first patient to undergo Gamma Knife radiosurgery had a craniopharyngioma.s. Literatur , s. Literatur
2.4 Application to Vestibular Schwannomas
Leksell himself had little interest in the potential commercial uses of his many inventions and was by nature cautious in the introduction of the new technologies he conceived. Various trainees or collaborators were tasked with individual application of both the prototype Gamma Knife and the second-generation device installed at the Radiumhemmet of the Karolinska Hospital in 1975 (Fig. 2‑1 ). Georg Norén was a disciple of Leksell and was in neurosurgical training at the Karolinska Institute, where Leksell served as professor for many years. After Leksell in 1969 had initiated the study by treating the first three patients, Dr. Norén gradually took over and was given the task of developing single session radiosurgical VS ablation using the prototype device.s. Literatur At that time, the technologies to both diagnose and image the tumor target were rudimentary. In addition, the dose planning generally required that either a single or up to three overlapping isocenters were used to produce a complete and adequately precise dose distribution throughout the tumor. Jürgen Arndt, the first Gamma Knife medical physicist, was actively involved in this early development process. Dose planning initially was done based on partially computer-generated freehand-drawn tumor periphery and isodose configurations and the treatment time was calculated based on a nomogram. Starting in 1979, the display of the radiosurgical dose plan with the isodoses had to be printed at the appropriate magnification and then overlaid on hard copy CT images.

In addition to the imaging obstacles, the stereotactic frame itself did not fit into the aperture of the secondary and tertiary collimators that focused the gamma rays through the slit-shaped collimators of the original prototype Gamma Knife. Innovative solutions included the use of a plaster of Paris and later thermoplastic cast wrapped around the patient’s head (Fig. 2‑1 ). This strategy is strangely reminiscent of current-generation mask immobilization devices.
The first nine patients were imaged using air encephalography or positive contrast cisternography (Fig. 2‑2 ). In 1976, the first stereotactic CT for a VS patient was performed using an EMI scanner with water bag and a special interface without the frame attached during scanning.s. Literatur The introduction of the first CT scanners without the water bag in 1979 facilitated the targeting of the tumor using thin slice axial imaging with the frame attacheds. Literatur (Fig. 2‑3 ). By 1987, more than 180 patients had been treated by radiosurgery.s. Literatur


When the first nine VS patients were treated with the prototype Gamma Knife in the era between 1969 and 1974, nothing was known about optimal single radiation dose for these tumors. Leksell had come to the conclusion that a minimum dose to the tumor periphery of 25 to 35 Gy should be prescribed. There was evidence at the time that peripheral nerves would tolerate even higher doses. Remarkably, only one patient in this series had slight temporary facial hypesthesia and no one developed facial weakness. The tumor control overall was good. The size of the intracranial portion in this series in general was 1 to 2 cm. When the second Gamma Knife was installed in 1975, CT was available for the first time. The tumors actually were treated with a margin dose of 25 to 35 Gy. This resulted in a quite high incidence of trigeminal (18%) and facial (45%) neuropathy, as well as hearing loss. However, the tumor control was good. Starting in 1976, dose was reduced to 16 Gy, which reduced neuropathies other than hearing loss to a zero level. In this series, the size of the intracranial portion of the tumors was up to approximately 2.5 cm. For the first time, the 4-mm collimators were available, specifically designed to cover the intracanalicular portion of VSs.s. Literatur
In 1988, a new Gamma Knife model B with freshly activated sources was installed at the Karolinska Hospital. Using identical dose planning and radiation dose levels, the incidence of facial weakness again increased to 27% (no facial hypesthesia documented).
Meanwhile, when the very first series of nine patients returned for follow-up, they underwent CT and later MRI. This imaging was meticulously compared to the earlier air and positive contrast studies. This revealed that these tumors had been treated with a peripheral dose in the range of 10 to 15 Gy. This explained the good tumor response paired with the almost nonexistent incidence of neuropathies. Based on these findings, Norén decided (Leksell had passed away in January 1986) to reduce the standard minimum peripheral tumor dose to 12 Gy prescribed to 50%. The 71 tumors treated between 1989 and 1990 demonstrated growth control (shrinkage or stable size) in 93% of cases.s. Literatur Three tumors were retreated with Gamma Knife giving a total rate of growth control after radiosurgery of 97%.
Hearing levels were much harder to maintain and virtually all patients experienced hearing impairment over time. Overall, in this early experience, 20 of 180 patients had delayed tumor growth, 11 of who required delayed surgical removal and CSF diversion by shunting was required in 10%.s. Literatur Norén’s early work confirmed the potential future role that radiosurgery might achieve. He established new outcome benchmarks for VS management: life preservation, morbidity reduction, tumor growth prevention, and improved long-term cranial nerve outcomes.s. Literatur , s. Literatur
2.4.1 The Expansion of the Role of Gamma Knife Radiosurgery for Vestibular Schwannoma
In 1987, the first 201 source Gamma Knife (unit no. 5) was installed at Presbyterian University Hospital in Pittsburgh.s. Literatur Among the earliest patients treated were those with VS. These patients wished to avoid the potential morbidity of microsurgical resection or had serious medical comorbidities precluding open surgery. All patients had enlarging symptomatic tumors confirmed by CT. It became clear in the first few years that the margin dose prescription suggested by the early Swedish experience resulted in too high a risk of new but usually transient facial and/or trigeminal neuropathy as well as inadequate hearing preservation rates. A margin dose de-escalation study commenced. Subsequent experience sought to define the therapeutic window—low cranial nerve complications plus long-term tumor control. During this dose optimizing era from 1987 to 1995, many other technological features emerged to further improve Gamma Knife SRS treatment. These advances included the first use of MRI to target the tumor (1991); improved multiple isocenter medical physics planning (first using KULA and then conversion to early generations of Leksell GammaPlan); and earlier detection and treatment of patients when tumor volumes were somewhat smaller. In addition, the installation of updated Gamma Knife technologies also contributed to ever improving outcomes. The U unit design gave way to the B unit, which facilitated reloading. The C unit incorporated true robotic positioning of the isocenters. The larger aperture, fully robotic Perfexion Gamma Knife was installed in Pittsburgh in 2006.
By 2017, almost 2,000 VS patients had undergone Gamma Knife SRS at the University of Pittsburgh Medical Center. Other worldwide centers reported large patient experience, including the Gamma Knife program at the Hôspital de la Timone in Marseille, France, where more than 3,000 VS patients underwent radiosurgery.s. Literatur According to the tracking of patient volumes by the Leksell Gamma Knife Society, worldwide experience by 2016 had exceeded 100,000 VS patients. This experience was conducted using virtually the same paradigm at more than 300 worldwide centers: stereotactic frame application, high definition targeting most often with MRI, highly conformal and selective dose planning using advanced high-speed image-integrated computers (dose prescriptions typically of 11–13 Gy at the tumor margin), and single-session delivery of the therapeutic radiation—wheels-in to wheels-out in a single day.
Publications about outcomes after Gamma Knife SRS were produced at an impressive rate, so that by 2016 more than 300 publications related to outcomes of Gamma Knife radiosurgery for VS were available (Fig. 2‑4 ). Based on these scientific publications and many national and international forums, the value of radiosurgery as a primary management of VS was established. Long-term tumor control rates of more than 95%, facial neuropathy rates of less than 1%, and hearing preservation rates in 50 to 90% of patients (depending on preoperative tumor volume and hearing status) became an expected outcome for patients undergoing Gamma Knife SRS.s. Literatur

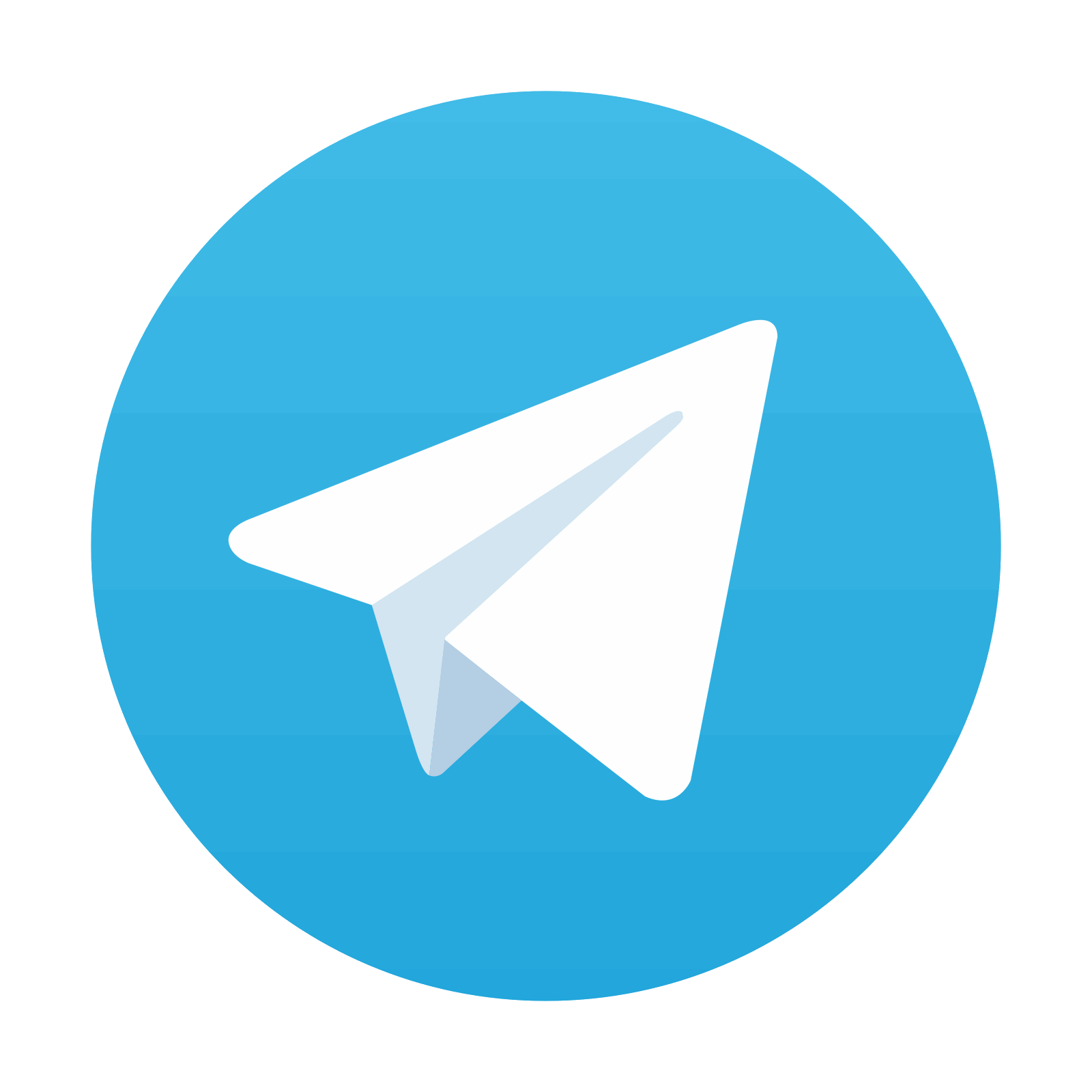
Stay updated, free articles. Join our Telegram channel

Full access? Get Clinical Tree
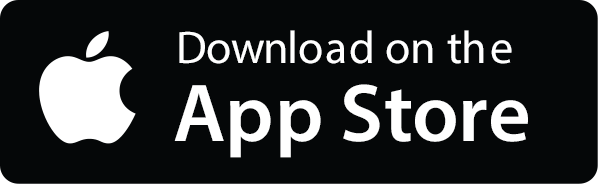
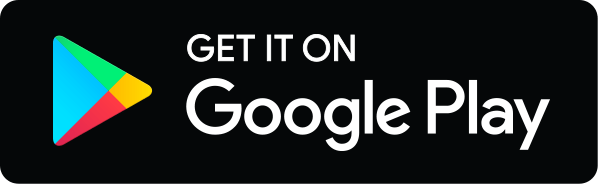
