2 Intraoperative Neuronavigation
Abstract
Innovations in intraoperative neuronavigation over the past two decades have enabled many of the recent advancements in epilepsy surgery. First utilized to aid with resective surgery planning such as temporal lobectomy or corpus callosotomy, improvements in the image fusion technology and stereotactic accuracy have made intraoperative neuronavigation a critical feature of seizure foci localization and new ablative and neuromodulatory approaches such as laser interstitial thermal therapy and responsive neurostimulation. From frame-based to frameless to robot-assisted stereotaxy, we will review the wide array of techniques of stereotactic neuronavigation that have increased operative efficiency, accuracy, and efficacy of epileptogenic zone localization and surgical treatment of medically refractory epilepsy.
2.1 Introduction
The surgical treatment of epilepsy has long been a mainstay for refractory disease. 1 Treatment of focal seizure disorder via resection was first recorded in 1884 and was closely followed by successful treatment via intraoperative electrical stimulation of the cortex in 1886. Since then, there have been many advancements in epilepsy surgery that have worked to improve the technique and precision associated with neurosurgical treatment. 2 One such advancement is intraoperative neuronavigation, whereby imaging data are utilized to provide real-time anatomic guidance to the surgeon during the procedure. Neuronavigation techniques have been shown to improve surgical outcomes in temporal lobe epilepsy, 3 corpus callosotomy, 4 and other epilepsy surgeries. 5
Neuronavigation has also been instrumental to the development of new approaches for seizure foci localization. Determining the exact location and parameters of the epileptogenic zone is crucial to surgical epilepsy treatment success. One way in which this is done is through the implantation of subdural electrodes that rest on the cortical surface and are tunneled out at the margins of the craniotomy. 6 In this technique, MR imaging studies are utilized for anatomic planning and then are incorporated into image guidance systems to guide the placement of electrodes intraoperatively. After placement, these images are fused with a postimplantation CT scan to anatomically localize exact electrode placement. Video EEG monitoring from the electrodes allows for precise foci localization and resection planning. Stereoelectroencephalogr aphy (SEEG) is another method of diagnostic study of patients with refractory epilepsy which relies on neuronavigation techniques. 7 Electrodes are placed at depth within the hemispheres to allow for greater three-dimensional coverage and more accuracy in epileptogenic foci triangulation. Computer technology aids this procedure in a similar way, allowing for neuroimaging integration before, during, and after the placement of depth electrodes.
Methods of recording include the opportunity for more long-term monitoring and neuromodulation. Responsive neurostimulation (RNS) relies on an implantable device that is used to detect and respond to seizures with electrical stimulation. 8 This programmable system has been shown to detect seizure activity with high sensitivity and effectively stop epileptic activity through responsive electrical bursts. While deep brain stimulation, using continuous electrical stimulation, has successfully treated a myriad of neurological disorders over the last three decades, 9 this novel closed loop system allows for adaptable and efficient treatment of seizures as they arise. 10
These methods of seizure detection rely on neuronavigation in a way that also befits newer, more minimally invasive approaches for seizure foci resection. Open surgical resection can achieve high rates of seizure control but is complicated by a host of unwanted effects. 11 As such, the demand for smaller surgeries has grown. In turn, this has increased the reliance on neuronavigation to accurately localize the epileptogenic zone in order to prevent over-resection. 5 Laser interstitial thermal therapy (LITT) treatment for seizures uses a minimally invasive catheter to cause thermal ablation over a well-demarcated area to increase the precision of therapeutic treatment. 12 While tissue ablation is performed under real-time MRI visualization, modern image guidance systems allow for LITT catheter placement in the operating room or in the MRI suite. 13 LITT is associated with a good side effect profile and is an effective therapeutic option for medically intractable epilepsy.
More than 50 million people suffer from epilepsy worldwide and 20 to 40% of this burden has been deemed medically intractable. 14 Long-term outcomes for these patients depend on effective diagnostics and accurate surgical procedures. As the demand grows, the technology needs to follow suit. Neuronavigation techniques afford surgeons more guidance and a higher degree of reliability than traditional methods. In the following sections, we will explore the opportunities for intraoperative neuronavigation in the surgical treatment of epilepsy.
2.2 Frame Based
The usage of skull-fixed stereotactic frames is one of the most commonly utilized methods for neuronavigation and provides procedures with a high level of accuracy. 15 There are several different types of frames available, which can be adjusted along x, y, and z coordinates to localize the surgical target. Some of the most popular models are arc based, including the Leksell Frame and the Integra CRW. These require the operator to first identify the best axial slice for entry and planning targets along the moveable arc. 16 In order to conduct higher accuracy procedures, imaging can be conducted in relation to one of these frames, after it has been semipermanently adhered to the patient’s skull. 17 There are several different compatible workflows for presurgical imaging that vary by surgeon preferences and level of comfort, as well as what imaging modalities are available at each institution. Frame attachment is typically completed at some point preoperatively, usually on the day of surgery, in an awake patient. The surgeon will position the frame while facing the patient and mark four projected screw entry sites on the scalp. 18 At this point, local anesthetic is injected into each of the sites and careful measurements are made to gauge depth prior to screw insertion and tightening. After the four pins are in place, the frame is tightened to adequately secure it to the patient’s head. The patient can then proceed to imaging. Advances in CT scanning and image fusion have allowed for the fusion of preoperative anatomic planning MRIs, completed prior to frame placement, and a verification volumetric CT scan, performed after frame attachment on the day of surgery. 19 These are fused and uploaded to the computer for intraoperative guidance. For asleep procedures, this allows for frame placement and image fusion to take place after induction to avoid the discomfort of affixing the frame on an awake patient. Typically, an additional intraoperative verification scan can be completed via either intraoperative CT 19 or MRI-based methods. 20 However, more recently, frame developers have created localizers that allow for three-dimensional MR imaging with computer reconstruction after the placement of the frame. 21 In this scenario, the attachment of a MRI frame localizer is required prior to imaging. 22 This then allows for more accurate reading and meshing of the two scans via electronic fusion, functioning in much the same way as a preoperative CT scan.
The incorporation of these frame-based techniques has dramatically improved electrode placement for both DBS and epilepsy, as verified by postsurgical imaging. 23 SEEG is another area of application, where frame-based stereotaxy allows for improved placement and access to deep cortical structures. 24 As previously described, SEEG involves intracranial electrode placement in order to monitor seizure activity and triangulate foci. In a comprehensive review of placement accuracy, frame-based systems were shown to be superior to frameless methods. 25 The mean entry point error was 1.43 mm (95% confidence interval [CI]: 1.35–1.51) for frame-based models and 2.45 mm (95% CI: 0.39–4.51) for frameless. LITT methods have also relied on frame-based modeling, whereby the point of interest for the laser ablation is calculated in relation to the stereotactic frame. With recent advances, this procedure can be performed using a real-time MRI system for neuronavigation. 26 The secure frame placement allows for full control and aides the computer in determining the relative location of structures. While this has proven to be an effective strategy for localization, there are some limitations. The frame structure itself can be unwieldy, and the application prior to imaging and surgery results in a longer procedure for both patient and surgical team. 27 Additionally, there is some patient discomfort associated with frame attachment in the awake patient prior to induction. One large downside is that the frame and four pins limit cranial access and provide decreased entry points for the placement of additional electrodes. 28 As computer calibration and accuracy improves, methods that do not rely on a physical frame device are gaining popularity.
2.3 Frameless
Frameless systems provide real-time anatomic information through various imaging technologies that eliminate the need for a fixed frame. 29 This category encompasses several different technologies, including modified stereotactic arcs, arm-based devices (with and without aiming facilities), and skull-affixed devices 30 (▶Fig. 2.1). These systems use sensors and imaging data to create computer-generated models of where the surgical instruments lie in relation to anatomical structures. This is commonly used to help plan craniotomies, because it accurately provides information about general anatomic location. 31 This can be useful for both open resections of the epileptogenic zone and for electrode placement, as in SEEG or RNS. The different methods of frameless registration each have individual technical considerations. 30 Modified stereotactic arcs use a stable head holder attached to an adjustable coordinate platform containing a single armed yoke. This creates a similar plane and point of entry as the previously discussed frame-based systems. Arm-based devices include retractors, which lack aiming facilities, and jointed arms, which can have aiming devices. Retractors can be attached to either the head or the operating table and aligned with the planned trajectory. Jointed arms can be connected in much the same way but can also include a rotating ball head within a lockable bearing or multiple joint heads. These can be paired with aiming devices made by the respective companies, in order to facilitate the surgical trajectory. Skull-affixed devices are usually mounted within a burr hole or the skull after the surgery begins and the trajectory is determined. The levels of target error vary between these systems, as shown in ▶Table 2.1.

Frameless device | Mean target error ranges | Range |
Modified stereotactic arcs | n/a | Within 2.5 mm |
Arm-based devices Without aiming With aiming facilities EasyTaxis Vertek | 1.5 mm 4.4–5.4 mm 3–4 mm | 1.0–2.1 mm 1.2–8.1 mm 0–7 mm |
Skull-affixed devices Snapper Nexframe Starfix | 1.5 mm 0.5–3.2 mm 1.99–2.8 mm | 0.6–3.4 mm 0.2–6.4 mm 0.14–4.1 mm |
Source: Adapted from Widmann et al. 30 |
Skull fiducial markers have proven to provide the most reliable system calibration in frameless methods. 32 In a theoretical model similar to frame application, markers are applied to the skull prior to imaging and used to calibrate the computer modeling during intraoperative imaging. This method has proven to be equal in accuracy to frame-based systems and may result in increased number of contact placement due to the greater freedom of device positioning. 33 Frameless neuronavigation may also decrease the need for re-resections. 34 As compared to conventional surgery, frameless neuronavigation improved the rate of re-resections by 16.5%. However, some factors are more difficult to control in a frameless system. In addition to the logistical and mechanical properties of the system, accuracy in image-guided surgery is reliant upon avoidance of patient movement during imaging and the fixed placement of registered points. In these domains, frameless systems have been criticized in the past for being less accurate. 35 However, reviews have determined that the submillimeter differences in accuracy between these systems do not translate into significant differences in clinical outcomes. 36
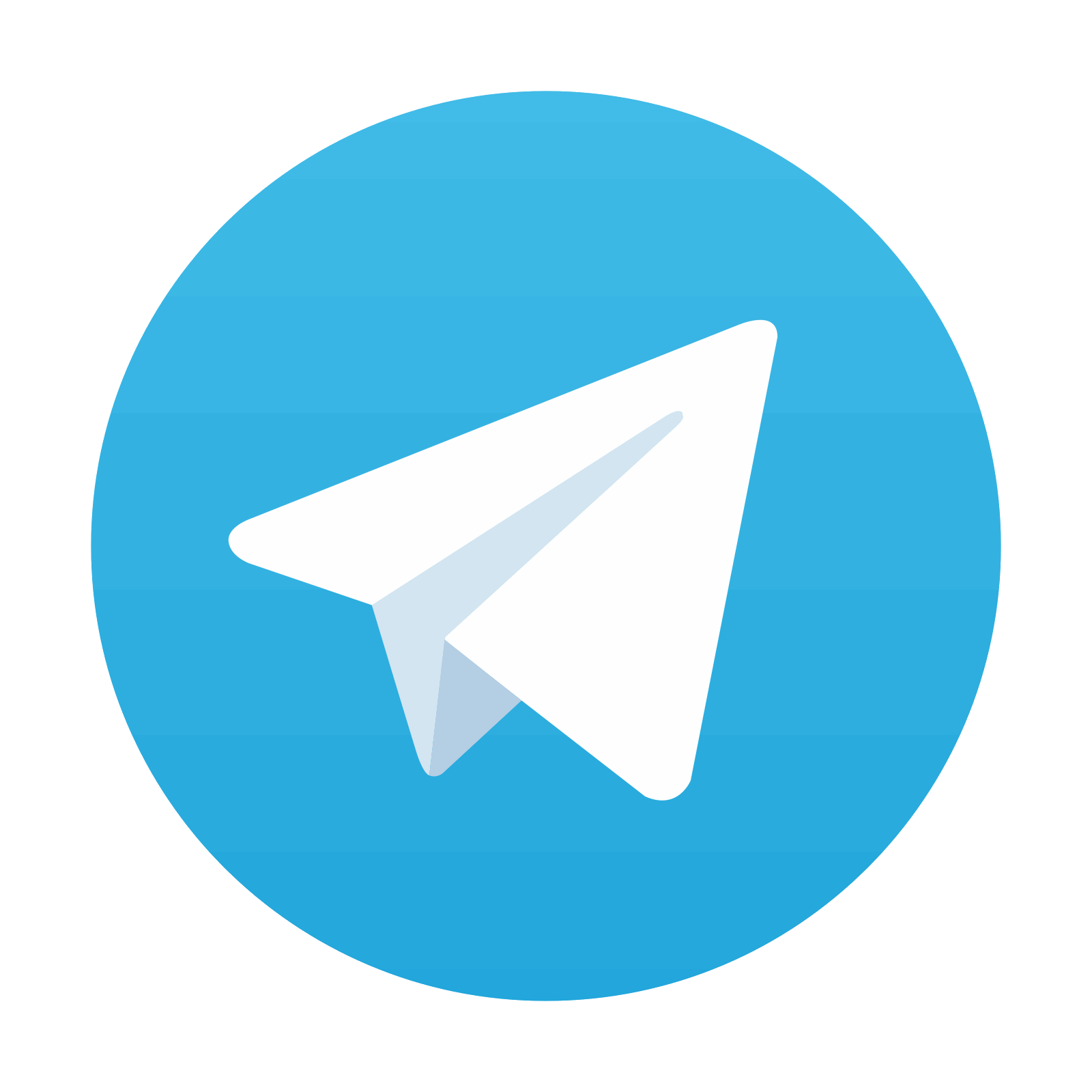
Stay updated, free articles. Join our Telegram channel

Full access? Get Clinical Tree
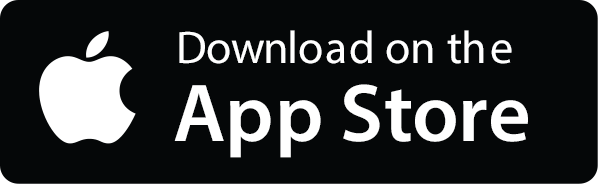
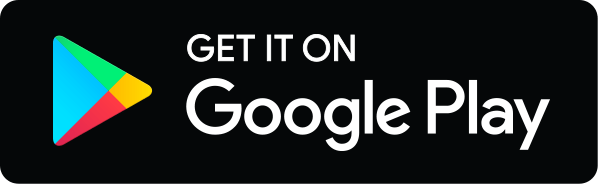