23 Interbody Constructs
Interbody constructs provide the ability to optimally bear axial loads along the neutral axis without applying a significant bending moment to the spine. This enhances the chance of achieving an arthrodesis because of bone compression–related augmentation of the bone healing–enhancing forces that are “seen” during assumption of the upright posture (Fig. 23.1). It goes without saying, however, that nonautologous interbody fusion materials and substrates have met with varying degrees of success. The documentation required to validate the use of such materials and substrates was, and remains, weak. 1

Interbody devices consist of bone implants, implants other than bone, or a combination. They may be nonabsorbable or absorbable—with fluctuating, intermittent, and fleeting enthusiasm for the latter type shown over the years. 2 They can be used alone or in combination with other implants (cantilever beams, three-point bending or tension-band fixation implants) that may be applied via either a ventral or dorsal approach.
The discussion of interbody constructs in this chapter is region-specific. Because of the common application of cages in the lumbar region, most of the discussion of cages appears in the section on lumbar spine constructs.
23.1 Cervical Spine Constructs
Ventral cervical spine surgery is associated with its own unique challenges and problems. 3 For the most part, though, these challenges have been overcome as experience has accrued. Cervical spine interbody constructs are commonly used today. Bone was the first interbody implant used in the cervical spine. 4 It remains the most frequently used. Following ventral discectomy or corpectomy, autologous iliac crest or allograft bone is often used as a bony spacer or strut. Tricortical iliac crest grafts are usually used. Some have suggested that the crestal portion be placed ventrally, and others that it be placed dorsally. The ventral positioning of the crestal portion provides the greatest cortical bone volume in the region of the anterior column of Denis. This provides a greater advantage for kyphosis prevention by positioning the load-bearing portion of the strut in line with the ventral vertebral body cortex. This takes advantage of the “boundary effect,” which is defined as “the phenomenon of increased axial load-bearing ability associated with the abutment against or gripping of the wall of an inhomogeneous structure (see Chapter 29 and Fig. 23.2a), of which the wall is of greater density and integrity than the inner portions of the structure.” A vertebral body is such a structure. Careful and safe placement of the strut is mandatory. Multiple strategies have been employed to improve safety during strut placement. Such a technique is depicted in Fig. 23.2b–d.

Clinical outcome may be related to sagittal plane alignment. 5 Positioning the crestal portion dorsally, in line with the middle column of Denis, provides good axial load-bearing ability, but perhaps suboptimal ability to prevent kyphosis (Fig. 23.3), and it may assist with attaining and maintaining sagittal alignment. The ventral placement of the crestal portion is biomechanically prudent, particularly when other implant adjuncts are not used. This allows load sharing between the anterior column (interbody strut) and intact posterior column structures (e.g., the facet joints). However, dorsal placement of the crestal portion of the graft may provide advantages when ventral cantilevered implants are used in conjunction (Fig. 23.4). This permits the middle column of Denis and the ventrally placed implant to share the load during the acquisition of fusion. 6 These points have also been studied in the thoracic and lumbar regions. 7 With accompanying long dorsal constructs, though, the location of the axial load-bearing intervertebral component, with respect to ventral–dorsal endplate positioning, does not appear to matter. 8


Nonautologous bone alternatives may also be used. The most common of these is the fibula allograft strut graft. Its high modulus of elasticity relative to parent vertebral body bone encourages subsidence. Ideally, the modulus of elasticity of the strut should approximate that of the accepting body (e.g., old endplate). Two factors are significant in this regard: (1) Fibula has a high ratio of cortical to cancellous bone, and (2) the cortical bone component of a graft heals very slowly. Hence, there exists a diminished ability to heal, or at least heal rapidly, when such grafts are employed. This negative attribute can be partially neutralized by the placement of autologous medullary bone in the central (medullary) region of the allograft fibula strut. This provides an enhanced bone-healing capacity component to the already present structural component of the allograft fibula.
The iliac crest graft can be problematic for long bony strut applications because of its curvature. Careful selection of the graft harvest site and meticulous contouring of the graft so that it fits snugly into the vertebral body trough minimize the chance of graft fracture or dislodgement (Fig. 23.5 and Fig. 23.6). This latter technique also aids vascularization and bony ingrowth from the side by the retained portion of the vertebral body walls, which enhances the fusion rate (Fig. 23.7). Most fibula struts cannot take advantage of this phenomenon because of the discrepancy between the diameter of the strut and the diameter of the trough (Fig. 23.8). Finally, long bony struts, particularly fibula, are prone to fracture, albeit rarely. This most commonly occurs at about 4 to 12 months after surgery, when the integrity of the bone graft at the mid-strut level is at a nadir. This is secondary to a time-related diminution of structural integrity caused by resorption. After this time, bone integrity increases because of the vascularization, remodeling, and healing of the intermediate portion of the strut. The latter healing process occurs from both termini of the bone graft, at the vertebral body–allograft junction toward the middle of the graft. The middle of the graft, therefore, is the last to be structurally reconstituted, thus explaining the common occurrence of fractures located in this mid-strut region, even in the face of apparent fusion acquisition at both termini (Fig. 23.9). If screws are placed into the graft, they may further diminish the integrity of the fibula strut. Other factors adversely affect the healing process, as well; smoking, in particular, adversely affects fibular strut graft fusion rates. 9 The aforementioned factors should be taken into consideration during the surgical decision-making process. The advantage of fibula as a construct component can be enhanced in selected cases (e.g., salvage-type situations) via the use of vascularized fibular grafts. 10 Such techniques obviously should be considered in extenuating circumstances.





Both flat-faced (i.e., cylindrical mesh cages) and round-faced (i.e., threaded interbody fusion cages) may be used in the cervical spine. Flat-faced cages present their flat face to the endplate fusion surface, whereas round-faced cages and dowels (e.g., the Cloward cervical technique) present their round face to the endplate fusion surface. Flat-faced cages are commonly used in the cervical region, particularly after single- or multiple-level corpectomies (Fig. 23.10). 11 , 12 Wilke and colleagues, in a human biomechanical study, demonstrated that postoperative neck movements caused subsidence to a greater extent with round-faced cages than with flat-faced cages. 13 However, motion does not appear to affect clinical results. 14 Biomechanical considerations regarding these constructs are discussed in a later section of this chapter, “Lumbar Spine Constructs.”

For long ventral cervical struts, construct integrity is not necessarily positively affected by ventral cantilevered implants (i.e., ventral plates). 15 , 16 Additionally, the surgeon must be cognizant of the effect of solid arthrodesis on adjacent motion segments. Shear strain at adjacent segments has been shown to increase following fusion. 17 Curiously, Payer et al and Thomé et al demonstrated that the implantation of an empty carbon fiber composite or a titanium strut/cage, respectively, after single-level anterior cervical discectomy resulted in a high rate of fusion. 18 , 19 Could it be that simply disrupting the endplate and providing structural stability, without the placement of autograft or other fusion substrate, is sufficient? Other graft substrates have been employed, 20 but allograft remains the gold standard.
23.2 Thoracic Spine Constructs
The thoracic spine does not present particularly unique challenges and problems compared with the cervical and lumbar regions. The often required transthoracic approach necessitates violation of the chest cavity. This poses its own set of risks and challenges. 21 Of note, however, is that the heart-shaped configuration of the thoracic vertebrae in the axial plane, particularly in the upper thoracic region, must be appropriately considered before implant application (Fig. 23.11). Additionally, suboptimal surgical exposure may limit interbody strategies. Dorsal approaches to ventral strut placement provide significant advantage in appropriately selected cases. 22 This has led some to prefer nonoperative alternatives in this region, which have met with clinical and anatomical success in selected cases. 23 , 24 Many surgical alternatives exist, however. 25 Surgical decision making should be carefully considered on the basis of a load-sharing classification scheme (see Chapters 19 and 40). 26

Interbody struts rely, to one degree or another, on endplate integrity; however, this concept has been challenged. 27 Ventral screw–rod and screw–plate constructs provide a significant advantage regarding the restoration of stiffness compared with dorsal short-segment fixation strategies. 28 Deformity progression (kyphosis), however, may be problematic regardless of the technique employed.
Interbody acrylic is applied perhaps most commonly in the thoracic spine. 29 With this technique, with or without wire reinforcement (Fig. 23.12), the surgeon must be aware of the gaps created at the acrylic–bone juncture. These gaps are created in part by blood, which is present at the time of acrylic application (Fig. 23.13). They can be avoided to some degree by using the chest tube technique described by Errico and Cooper 29 and others. 30 This strategy causes the acrylic to be tightly approximated to the vertebral body because of the pressurization strategy used (Fig. 23.14). Care must be taken to avoid extravasation and neural element impingement (Fig. 23.15) and thermal injury of neural elements. The latter is prevented by copious irrigation during the exothermic polymerization reaction. Percutaneous vertebroplasty with acrylic has been used for osteoporotic compression fractures (pathologic fractures related to osteoporosis) with success. 31 A strategy for creating a hollow cylindrical polymethylmethacrylate strut for interbody reconstruction has been devised. 32 This is applicable in the cervical and thoracic regions. Alternatives to acrylic are, or will become, available. 33 – 37




23.3 Lumbar Spine Constructs
Because of recent enthusiasm regarding lumbar interbody fusion, this surgical strategy, particularly the use of threaded interbody fusion cages (TIFCs), is given special consideration in this section. Other lumbar interbody strategies are also discussed and compared with dorsal strategies.
There is a significant need for effective strategies that minimize the incidence of failed lumbar spinal operations. Many factors have been studied in this regard, including fusion. 38 – 43 One should, however, be careful regarding the determination of the operative indication for lumbar fusion. Some diagnostic tools, such as discography, are controversial. Therefore, they must be used and interpreted cautiously. 41 , 44 , 45 It is in this vein that interbody fusion techniques provide many theoretical and proven advantages over dorsal onlay grafting techniques. These include (1) a decreased incidence of pseudarthrosis, (2) an accelerated rate of fusion acquisition, and (3) an increased axial load-bearing ability.
In the lumbar spine, additional extenuating circumstances may neutralize some or all of the aforementioned advantages, not the least of which includes the technical pitfalls associated with the operative procedure. 46 Other factors include the fact that the lumbar spine bears substantial axial, torsional, and translational loads. The latter is particularly relevant in the lower lumbar spine (lumbosacral junction), in which axial load force vectors may cause resultant translational and angular force vectors (Fig. 23.16).

Furthermore, complete lumbar discectomy (complete disc evacuation), as well as corpectomy, is difficult to achieve in this region from a dorsal approach. Overly aggressive endplate débridement may result in the loss of integrity of this substantial component of axial load bearing (the endplate); insufficient débridement may result in the preparation of an inadequate graft bed and nonunion (pseudarthrosis).
This portion of the chapter focuses on interbody strategies for lumbar and lumbosacral fixation. The next chapter (Chapter 24) focuses on more complex, predominantly dorsal strategies. Interbody strategies, for the most part, are employed as stand-alone strategies only when overt instability is not present. The strategies discussed in Chapter 24 are used when significant (overt) instability is present or when stability or spinal integrity is at risk.
23.3.1 Interbody Fusion Strategies
Dorsal Lumbar Interbody Fusion Strategies
Optimal disc space preparation for interbody fusion is difficult to achieve, particularly from a dorsal approach. Cloward popularized an approach in which this, as well as interbody fusion, could be achieved; he termed it posterior lumbar interbody fusion (PLIF). 47 Other positive reports followed. 48 – 51 Cloward emphasized careful endplate preparation, meticulous graft preparation, and the attainment of a high surface area of contact between the endplate and the bone graft (Fig. 23.17). 47 He did not use spinal implants other than bone. Others, however, were unable to achieve equivalent results. Hence, techniques and implants that facilitated discectomy and endplate débridement were developed. Others recommended the use of spinal implants, such as pedicle fixation, to enhance the clinical results of PLIF, 52 , 53 although the utility of this combination was questioned by still others. 54 A review of reported surgical techniques reveals that a failure to comply with the original tenets of Cloward, particularly regarding surface area of contact between the bone graft and endplate, may at least in part explain the variation in surgical results. Excessive loads, coupled with a suboptimal surface area of contact between the bone graft and the vertebral body, resulted in the use of a “spacer” that was suboptimally effective, and that could not adequately resist the axial and resultant translational and angular force vectors. Of significance in this regard is the fact that the chance of subsidence of an interbody bone graft into a vertebral body is inversely proportional to the cross-sectional area of contact (Fig. 23.17a). 55 This is consistent with Cloward’s technique and teachings, 47 and it has contributed, at least in part, to the observed high failure rates of interbody lumbar fusions. In summary, both the cross-sectional area and the relationship of the strut to the margin of the endplate significantly affect subsidence. 56 Hence, in addition to a large surface area of contact being important, the location of contact is also important. Struts with lateral or peripheral (perimeter) endplate contact are associated with greater axial load bearing and subsidence-resisting ability than centrally placed struts. This is simply a manifestation of the boundary effect (Fig. 23.17b, c). This likely explains why transforaminal lumbar interbody fusion (TLIF) has been shown to be associated with fewer complications and better fusion rates than PLIF or anterior lumbar interbody fusion (ALIF) approaches. The latter two techniques usually involve the more central placement of struts than does the TLIF technique (see Chapter 12). 57 Regardless, it appears that circumferential lumbar spinal fusion (ventral plus dorsal fixation) provides superior clinical results compared with ventral fusion alone. 58 Femoral ring allograft strategies and variants with ventral screw fixation may improve ventral fixation, but not to the extent that circumferential fixation does. 59 With stand-alone techniques, the position of the patient during surgery (i.e., posture) does not affect postoperative lumbar lordosis. 60 This is not unexpected, in that in the absence of rigid dorsal or ventral fixation, the configuration of the spine “adjusts” after surgery, regardless of the patient’s posture at the time of surgery.

Others have considered both the mechanical (structural support) and biological functions (bone graft for bony fusion) of the “traditional PLIF” concept by employing rectangular cages that increase the surface area of contact of bone while providing structural support with the cage. 61
Ventral Lumbar Interbody Fusion Strategies
ALIF techniques have gained in popularity, including “minimally invasive” and laparoscopic approaches. 62 – 64 This was in part because of difficulties associated with the PLIF procedure. 65 However, complications with the surgical approach and a similar pseudarthrosis rate (compared with PLIF reports) initially plagued the ALIF procedure, as well. Therefore, it was often employed in combination with dorsal spinal implants (e.g., pedicle screw fixation). 66
Of interest is that allograft performs as well as, if not better than, autograft in ALIF procedures. 67 The selection of allograft type and source is controversial. The fibula and femoral ring have been compared in this regard. 68
Comparison with Lateral Intertransverse Fusion
With lateral intertransverse fusion, the fusion mass is placed at a significant distance from the instantaneous axis of rotation (IAR) and the neutral axis (Fig. 23.18). The amount of flexibility that persists following such an operation may be the cause of clinical failures, even in the face of a solid fusion. 69 The obligatory lateral soft tissue retraction and associated soft tissue injury are perhaps a causative factor regarding the loss of lumbar lordosis and chronic back pain associated with uninstrumented fusion, and the disappointing incidence of chronic back pain following both instrumented and uninstrumented fusions.

Despite its documented efficacy regarding arthrodesis acquisition, pedicle screw fixation for lumbar fusion became less popular in the 1990s, predominantly because of medical, legal, and related concerns. Hence, during this era, spine surgeons needed an easier and safer way of dealing with the lumbar fusion “problem.”
Perhaps the solution was interbody fusion. The elevation of disc space height via distraction requires that this elevation be maintained via the interbody strut employed. Solely dorsal techniques take advantage of the relatively stable state associated with the already collapsed disc interspace height in patients with advanced motion segment degeneration. Supporting this notion is the study of Kaito et al. 70 They observed that excessive distraction of the L4–L5 disc space during PLIF surgery was associated with a greater incidence of adjacent-segment degeneration. Contrary to this notion, however, are the findings of Schuler et al, who demonstrated that the best clinical outcomes following lumbar interbody fusion were in patients with less disc interspace height preoperatively. 71 It is hoped that time will provide the answer via clinical and basic science research. 72
23.3.2 Fusion Cages
Flat-Faced Fusion Cages
Flat-faced fusion cages expose the accepting fusion bed (i.e., the endplate region) to a flat surface rather than a round surface, like that of the TIFC; see the following (Fig. 23.19). Flat-face cages may be round, square, or rectangular at the end (Fig. 23.20), and they may have a parallel or trapezoidal shape in the sagittal plane to conform to the shape of the disc interspace (Fig. 23.21). They may have a high modulus of elasticity (e.g., stainless steel or titanium alloys), a relatively low modulus of elasticity (e.g., bone), or an intermediate modulus of elasticity (e.g., carbon fiber, polyether ether ketone [PEEK], or femoral ring allograft). The latter much more closely approximates the modulus of elasticity of the endplate and medullary bone. This theoretically reduces the chance of implant subsidence, which, however, may very well be irrelevant. 73 Allograft bone, though, has been shown to outperform expandable cages from a stiffness perspective. 74



Some flat-faced cages provide a greater surface area of contact with the accepting fusion bed than others, but flat-faced cages almost always provide more surface area than round-faced cages (TIFCs; Fig. 23.22a, b). 75 , 76 The greater surface area of contact, however, results in less bone-on-bone contact. This obviously deserves careful consideration. In this regard, larger-diameter titanium mesh cages provide significantly greater augmentation of implant–bone interface strength than do smaller=diameter cages (Fig. 23.22c). The addition of an internal end ring (Fig. 23.22d) provides a substantial further increase in interface strength. 77 However, it results in less bone–bone contact for fusion enhancement. Finite element modeling studies have demonstrated this, as well. In addition, differences in the elastic moduli of cages (i.e., differences in material stiffness) do not appear to affect load transfer to the cancellous core. 73 Contrary to such observations, however, are the findings of van Dijk et al, who noted that bioabsorbable implants enhanced interbody fusion compared with titanium implants, 78 and of others, who noted superb clinical results with devices whose modulus of elasticity approximated that of cortical bone. 79 Regardless of the findings of van Dijk et al, still others have observed that the incidence of cage migration and nonunion is greater with bioabsorbable implants. 80 All such factors and confusing findings must be considered during the decision-making process. 81 Of final note regarding migration. interbody flat-faced cages with endplate spikes significantly augment rigidity in tests of biomechanical flexibility. 82 Such testing strategies, however, may not always provide clinically relevant results. 83

Bone mineral density also affects the biomechanics of the strut–endplate region. 84 Flat-faced cages provide a significant advantage regarding the prevention of angular deformation at the cage–endplate interface (e.g., compared with interbody struts or TIFCs; Fig. 23.23). This, combined with their significant surface contact with the endplate, makes flat-faced cages a viable alternative to strut fixation with iliac crest, rib, or acrylic constructs, particularly with regard to longer constructs (Fig. 23.24). 85 , 86 Of note, the angle of insertion does not appear to affect the limitation of motion and stability of the construct—that is, ventrolateral and ventral techniques are equivalent from a biomechanical perspective. 87


Many flat-faced cages and struts are derived from allograft bone. They provide varying amounts of endplate contact and surface area of contact for the autologous bone placed within them. Hence, they vary in efficacy. 88
Finally, the heated debate over multiple discectomies versus corpectomy for the management of cervical spondylotic myelopathy continues. 89 The mechanics and clinical outcomes have been addressed in numerous venues and publications. Andaluz et al have presented a good case for corpectomy. 89
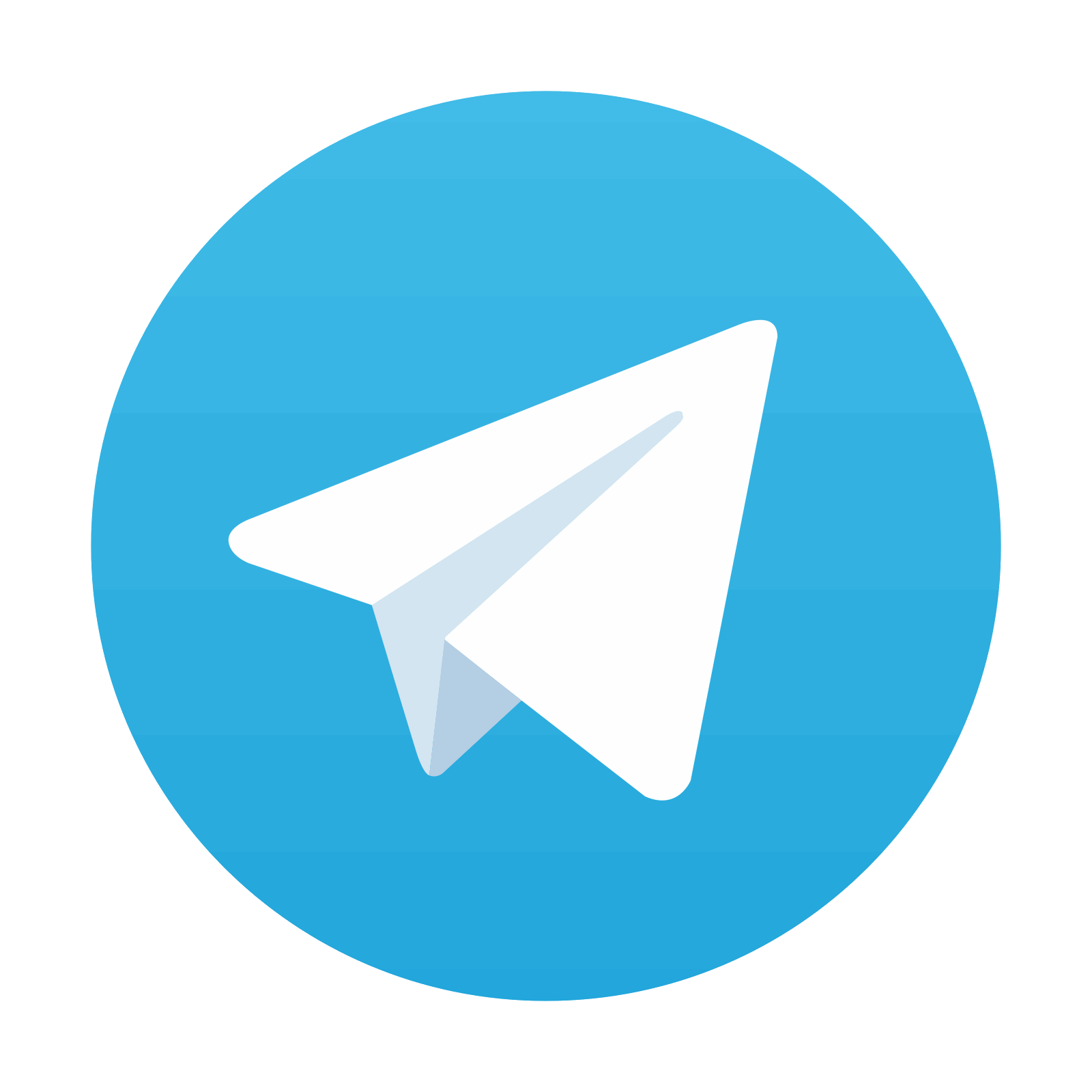
Stay updated, free articles. Join our Telegram channel

Full access? Get Clinical Tree
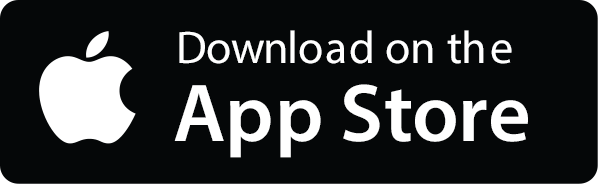
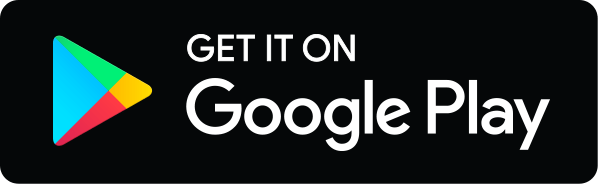
