24 Chiari Malformations and Syringomyelia
History
Chiari Malformations
Hans Chiari was a professor of pathology at The University of Prague, Czechoslovakia, and later at the University of Strasbourg. In 1891, his initial work on what would become known as Chiari malformation was published, and 5 years later, he published a classification system that is still in use today.1 In the 1896 publication, he mentioned Cleland and Arnold, who made incidental observations compared with Chiari’s thorough study of the malformation. Arnold’s name was added to the type II malformation to coin the term Arnold-Chiari malformation by Schwalbe and Gredig, in 1907, writing from Arnold’s laboratory in Heidelberg. Unfortunately, this has led to the loose application of the term Arnold-Chiari malformation to both type I and II conditions, and subsequently much confusion. The term Chiari II malformation is clear and the preferred terminology.
Syringomyelia
The original Greek word from which syringomyelia is derived means tubular cavitation of the spinal cord. In 1546, Estienne described an abnormal, fluid-filled space within the spinal cord. It was later described in continuity with the fourth ventricle and labeled syringomyelia by Olivier d’Angers in 1824.2 Abbe and Coley were the first to decompress a syringomyelic cavity, in 1892. But it was only in the 1950s that Gardner postulated the hydrodynamic theory and craniocervical decompression as a surgical method of treating syringomyelia when associated with craniocervical abnormalities.3
Definitions and Classification
Chiari Malformations
Chiari’s classification of these craniocervical malformations is based on the degree of hindbrain herniation and location ( Table 24.1 ). In Chiari I malformation, there is cerebellar tonsillar herniation through the foramen magnum. There may be associated syringomyelia. Other abnormalities that could be present include an elongated aqueduct, a stretched fourth ventricle, and a kinked lower medulla. The upper cervical nerve roots usually project inferiorly, and the posterior fossa is shallow.
Chiari II malformation has many associated congenital abnormalities of the craniospinal axis. It is typically associated with meningomyelocele and hydrocephalus. Apart from the cerebellar tonsils, the cerebellar vermis, fourth ventricle, and medulla herniate through the foramen magnum. In more severe deformities, the pons is also displaced below the foramen magnum. The upper cervical roots project superiorly, the pons is elongated, and the fourth ventricle is slitlike. Usually, the tentorium cerebelli is low lying and hypoplastic, with the superior cerebellum displaced upward through a wide incisura. Other dural abnormalities are hypoplasia and fenestrations in or partial absence of the falx cerebelli, with tight apposition between the cerebral hemispheres. Abnormalities of the skull include lacunae of the skull, erosion of the posteromedial aspect of the petrous pyramid (petrous scalloping), and enlargement of the foramen magnum.
To help differentiate Chiari I from Chiari II malformations, it is generally agreed that cerebellar ectasia in the presence of a neural tube defect, regardless of associated abnormalities or age of onset, constitutes Chiari II malformation.
In Chiari III malformation, there is herniation of the cerebellum and brainstem into a high cervical or sub-occipital meningocele. In Chiari IV malformation, there is cerebellar hypoplasia without cerebellar herniation. These last two categories are rare with limited neurosurgical relevance and will not be discussed further.
The iatrogenic, or acquired Chiari, malformation was not a recognized entity at the time of Chiari’s original classification. It has gained clinical significance and come into the literature as a result of neurosurgical interventions. Cerebellar herniation occurs through the foramen magnum (usually normal in size) due to a change in cerebrospinal fluid (CSF) dynamics from posterior fossa/upper cervical spinal cord surgery or a lumboperitoneal shunt.
Syringomyelia
A cyst involving the central canal and therefore lined with ependyma is known as hydromyelia, whereas a cyst outside the central canal is known as syringomyelia. The differentiation is therefore histological and not relevant in terms of clinical differentiation or management. Both are considered as syringomyelia for this chapter.
Milhorat classified syringomyelia based on autopsy and findings on magnetic resonance imaging (MRI) as communicating, noncommunicating, atrophic, and neo-plastic types ( Table 24.2 ).4 The atrophic and neoplastic types are not relevant to this chapter.
In communicating syringomyelia, there is dilation of the central canal, which is also in continuum with the fourth ventricle. Usually there is hydrocephalus due to obstruction to the CSF pathway distal to the fourth ventricle outlets. The central canal therefore acts like a “fifth ventricle.” Communicating syrinxes are less prone compared with noncommunicating cavities to dissect or rupture paracentrally, and therefore usually asymptomatic or associated with only minor neurological findings. Etiological conditions include postmeningitic and posthemorrhagic hydrocephalus, complex hindbrain anomalies, such as Chiari II malformation and encephalocele, and Dandy-Walker cysts.
In noncommunicating syringomyelia, there is either dilation of the central canal, which does not communicate with the fourth ventricle, or a separate extracanalicular syrinx that arises in the spinal cord parenchyma and does not communicate with the central canal or fourth ventricle (primary parenchymal cavitation as a result of spinal cord injury due to trauma, ischemia/infection, or spontaneous intramedullary hemorrhage).
Noncommunicating central canal dilatation is associated with obstruction to the CSF pathways at or below the level of the foramen magnum, the most common etiology by far being the Chiari I malformation. Other etiological factors are basilar invagination, spinal arachnoiditis, extramedullary compressions, tethered cord, and acquired tonsillar herniation.
Unlike a communicating syrinx, these syrinxes often dissect into the spinal cord parenchyma, usually to the dorsolateral quadrant of the spinal cord, and can extend to the pial surface to communicate with the subarachnoid space. Also, unlike a communicating syrinx, they are often symptomatic with neurological findings correlating to the anatomy of cavitation demonstrated on MRI.
Etiology and Pathogenesis
Chiari I Malformations
Chiari described hindbrain anomalies in infants and pre-term births.1 Therefore, subsequent descriptions of tonsillar herniation in adults assumed the condition to be present since birth. A difficult labor can cause excessive molding of the skull, thereby pressing the brain into the foramen magnum, with anoxia, hemorrhage, and hydro-cephalus setting the stage for chronic tonsillar herniation. There have been reports of familial aggregation of Chiari malformation and/or syringomyelia, implying an underlying genetic basis, with an autosomal dominant and predominant autosomal recessive inheritance pattern. Morphometric studies of the posterior cranial fossa have shown that the posterior fossa is small due to an underdeveloped occipital bone. Because the posterior fossa contents are of normal size, they are “squeezed” by the underdeveloped occipital enchondrium, with a secondary downward shift of the brain and upward shift of the cerebellar tentorium. Basilar invagination due to a more severely underdeveloped occipital enchondrium results in worsening of the overcrowded posterior cranial fossa.5,6 There also may be a role of craniospinal CSF pressure gradient in the pathogenesis of Chiari I malformations.
Chiari II Malformations
The dysraphic condition occurring in the setting of Chiari II malformations has been proposed to arise from many causes, including the arrest of normal closure of the neural tube, overgrowth during neurulation, reopening of a closed neural tube, secondary to altered CSF dynamics, and maternal vitamin B6 (thiamine) levels. McLone proposed a unifying theory to explain the development of Chiari II malformations. Due to failed neurulation, a dorsal myeloschisis is created, leading to excessive ventricular CSF drainage through the defect. The ventricular system is therefore unable to distend normally and does not exert its normal inductive Effect of pressure and volume on the surrounding mesenchyme and endochondral bone formation. This results in a small posterior fossa, and the developing cerebellum and brainstem herniate through the incisura and foramen magnum. As opposed to Gardner’s unifying theory, in which hydrocephalus is felt to initiate tonsillar and brainstem herniation, McLone suggested that hydrocephalus arises as a result of the hind-brain herniation. CSF outflow can be impaired by “collapse-induced” developmental abnormalities (blockage) at the cerebral aqueduct, obstruction of the outlets of the fourth ventricle, or obstruction at the level of the incisura. It is also possible that the abnormally low CSF pressure, as a result of overdrainage, may have an adverse Effect on developing arachnoid granulations, leading to hydrocephalus, especially after closure of the neural tube defect.
Acquired Chiari Malformations
Two theories have been proposed to explain the development of acquired Chiari malformations.
Cephalocranial Disproportion Theory
Shunting procedures, common to all such cases, can be associated with the arrest of skull growth. The cranial contents grow faster than the surrounding cranium, and skull growth is again stimulated as the brain continues to grow and fill the space previously occupied by the ventricular system, but not before a transient rise in intracranial pressure produces herniation of the cerebellar tonsils through the foramen magnum.7
Craniospinal Pressure Gradient Theory
According to this theory, the artificially created pressure gradient due to the relatively lower intraspinal pressure caused by the spinal shunting or drain is the driving force for the tonsillar descent. For acquired Chiari malformations, the conversion of a lumbar shunt to a ventricular shunt may eliminate the downward craniospinal pressure gradient, allowing the reversal of the tonsillar descent or at least arresting further migration.
Syringomyelia
Early Theories on Pathogenesis of Syringomyelia
Gardner’s and other earlier theories on the pathogenesis of syringomyelia assumed that there was a communication in all cases between the fourth ventricle and the syrinx. These early theories therefore focused on the transmission of pressure from the intracranial space into the central canal, via the fourth ventricle.
Gardner’s Hydrodynamic Theory
Prior to Gardner’s theory put forth in the late 1950s, the popular belief was that syringomyelia was the result of cystic degeneration in nests of glial proliferation, scattered throughout the spinal cord. Gardner postulated that in the presence of obstruction to the foramina of Luschka and Magendie, there would be exaggerated ventricular pulsations from the choroid plexus.8 At 6 to 8 weeks of the embryonic period, there could be failure to develop permeability to CSF flow in the roof of the fourth ventricle. The subsequent hydrocephalus was felt to cause posterior displacement of the primitive transverse sinus and herniation of the hindbrain and cerebellum through the foramen magnum. Because of obstruction at the foramina of Luschka and Magendie, the “water-hammer” Effect of the choroid plexus–driven CSF flow through the obex caused dilation of the central canal and subsequent syringomyelia. Therefore, Gardner proposed relieving outflow obstruction through lysis of arachnoidal adhesions and treatment of the syrinx by plugging the obex. However, Gardner’s theory cannot explain syrinx formation in most cases where there is no hydrocephalus, and the fourth ventricle outlet is patent.
Craniospinal Pressure Dissociation Theory
This theory was proposed by Cameron and later modified by Williams.8–10 Tonsillar herniation caused obstruction to the caudal flow of CSF, from the cranium at the foramen magnum. This resulted in a craniospinal pressure gradient, causing CSF to flow from the fourth ventricle into the central canal, with subsequent syrinx formation. differences from Gardner’s theory are outlined in Table 24.3.
“Slosh” and “Suck” Effects
Unlike Gardner’s theory, syrinx expansion was felt to be the result of increases in intrathoracic pressure and not intracranial pressure. Sneezing/coughing caused expansion of the epidural spinal veins and moved CSF from the spinal canal into the cranial subarachnoid spaces through the foramen magnum. In this process, the syrinx was compressed, and its fluid was pushed up and down the central canal, expanding the syrinx cavity (“slosh” Effect). At the end of the Valsalva maneuver, the tonsillar herniation prevented the return of CSF from the cranial cavity into the spinal subarachnoid space. Therefore, the pressure gradient (higher intracranial to lower intraspinal) continued to be maintained, forcing further CSF from the fourth ventricle, through the obex, and into the central canal, leading to further syrinx expansion (“suck” Effect). This hypothesis is supported by gradients in excess of 100 mmHg, documented after coughing episodes.9
“Milking” Action at the Level of the Foramen Magnum
Du Boulay10 showed that in response to cerebral hemisphere expansion due to arterial inflow during systole, there were periodic movements of CSF in the basal cisterns. He suggested that in the presence of tonsillar herniation, these movements resulted in a ball-valve plugging of the foramen magnum by the cerebellar tonsils. Therefore, during systole, the tonsillar impaction resulted in CSF from the basal cisterns being diverted into the fourth ventricle and the central canal. During diastole, retraction of the tonsils and brainstem resulted in a “milking” action at the foramen magnum, causing further movement of the CSF along the central canal. Such a repetitive activity, over time, could lead to the formation and expansion of a syringomyelia. During coughing, a large upward displacement of CSF throughout the spinal axis and up to 50% reduction in the anteroposterior diameter of the thecal sac occurred; therefore, an increase in spinal venous pressure was felt to be the main factor in syrinx expansion following a Valsalva maneuver.
Theories Not Relying on Communication between the Fourth Ventricle and the Central Canal for the Formation and Expansion of the Syrinx
Ball and Dayan’s Theory
Ball and Dayan found that in the walls of syrinx cavities were prominent small arteries and veins, as well as dilated perivascular spaces around the cavities.11 They felt the dilated perivascular spaces were likely to represent enlarged Virchow-Robin spaces. When India ink was injected into the lumen of the syrinx, it was found to spread out around blood vessels and reach the subarachnoid space. The authors therefore postulated that, with obstruction at the foramen magnum, syringomyelia could develop as a result of CSF dissecting into the spinal cord through dilated Virchow-Robin spaces.
Rennels et al.12 demonstrated that horseradish peroxidase in the CSF penetrates rapidly and deeply into the Virchow-Robin spaces at various sites of the central nervous system, and the penetration is dependent on systolic pulsations in the CSF. Stoodley and coworkers subsequently confirmed these observations.
Oldfield’s Theory
Oldfield and coworkers further expanded this theory.13 According to their mechanism, during cardiac systole, there is a pistonlike downward movement of the cerebellar tonsils at the foramen magnum onto the partially entrapped spinal CSF. The resultant spinal subarachnoid heightened systolic CSF pressure and increased pulse pressure cause bulk movement of the fluid from the subarachnoid CSF to the spinal cord, through the perivascular spaces, causing formation of a syrinx. This action also compresses the cord and causes pulsatile flow in the syrinx, leading to expansion of the syrinx.
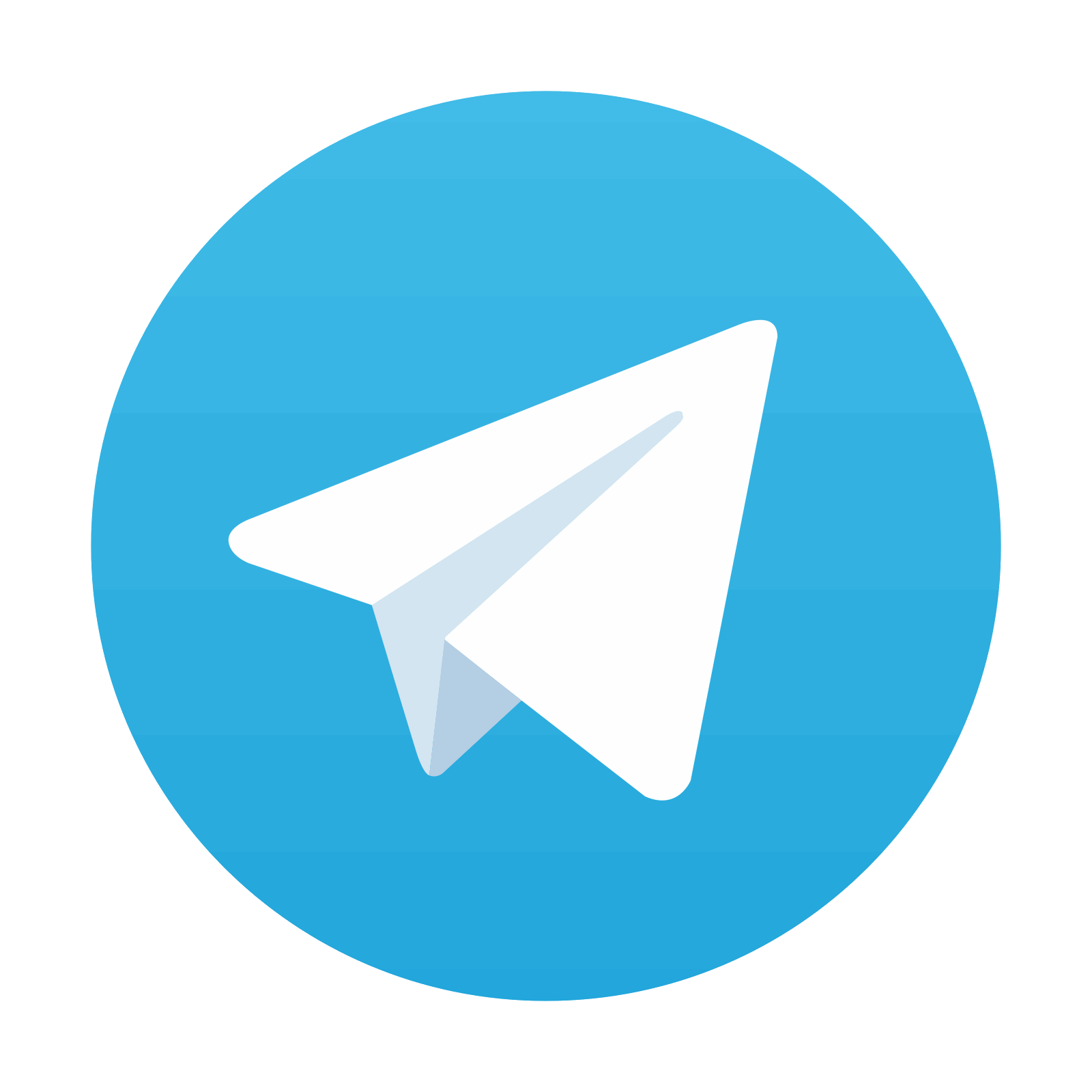
Stay updated, free articles. Join our Telegram channel

Full access? Get Clinical Tree
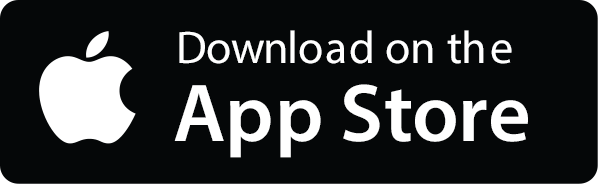
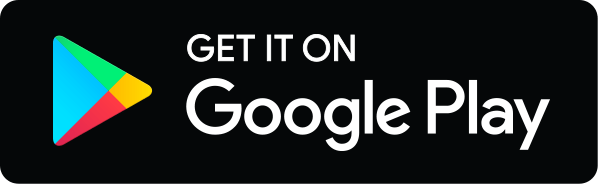
