26 Deformity Prevention and Correction: Component Strategies
This chapter addresses deformity and its correction and prevention. To convey and portray the fundamentals and their clinical techniques, a “building block” approach is undertaken here. First, commonly used strategies are discussed. Next, clinical applications are addressed, with a discussion of deformity-specific principles that build on these commonly used strategies. This is followed by a region-specific approach to deformity prevention and correction. Finally, factors that are not region-specific are addressed.
26.1 Commonly Employed Strategies
One or a combination of two fundamental techniques can be used for deformity correction: (1) implant force and bending moment application that “brings the spine to the implant” and (2) implant force and bending moment application with in vivo implant configuration alteration techniques. A fundamental understanding of these techniques provides the surgeon with a broadened surgical latitude and allows an individualized and customized implant selection process for the patient in each case. Finally, strategies that maintain the acquired correction must be used.
Implant forces, when “bringing the spine to the implant,” can be applied along any of the three axes of the Cartesian coordinate system. They are usually applied in the sagittal plane of the spine (e.g., lordosis or kyphosis correction or prevention). They can also be applied in the coronal plane (e.g., scoliosis correction or prevention; Fig. 26.1).

Implant force and bending moment application by means of in vivo alteration of implant configuration first involves application of the implant to the spine (insertion), followed by adjustment of the implant shape. This is achieved by one or a combination of three fundamental types of implant manipulation: (1) implant contouring, (2) derotation, (3) or the application of an intrinsic implant bending moment (Fig. 26.2).

26.2 Component Strategies for Deformity Prevention and Correction
26.2.1 Bringing the Spine to the Implant
Various techniques can be used to bring the spine to the implant. As mentioned previously, this is accomplished via the application of forces to the spine along one or a combination of the three axes of the Cartesian coordinate system. Forces applied along the long axis of the spine (e.g., distraction) can be used to correct compression deformations, as well as coronal and sagittally oriented translational deformations (Fig. 26.3). Bending moments applied in the sagittal plane are of a three- or four-point bending or applied moment arm cantilever beam type (Fig. 26.4).


Three- or Four-Point Bending Force Application
Three-point bending constructs were discussed in Chapter 17. The forces that they apply to the spine are common and, for the most part, well understood. Three- and four-point bending implants are a classic example of the strategy that “brings the spine to the implant.” They can be applied to reduce subluxations (Fig. 26.5). Crossed-rod techniques can be applied in the sagittal and coronal planes (see below). They are primarily used to correct angular (kyphotic) spine deformities via a three-point bending mechanism applied dorsally. Regardless, three-point bending techniques can be employed dorsally to correct deformity by essentially “bringing the spine to the implant” (Fig. 26.5a).

A ventral approach can be applied in the cervical spine to correct kyphotic deformities via a “bringing the spine to the implant” strategy, as well (Fig. 26.5b). Such a technique provides an advantage for both deformity correction and maintenance of fixation. The latter is achieved via the application of three-point bending forces (see Chapter 27).
Terminal three-point bending techniques can be used to “bring the spine to the implant” as well as to prevent the spine from “falling away from the implant” (Fig. 26.5c). This technique can be applied to any spinal level. It is most commonly used in the cervical region because of the lesser loads accepted by the implant and the relatively insubstantial design of the construct. It is most useful for the prevention or reduction of translational deformation (see Chapter 17).
Note that if a ventral translation deformation is to be corrected or prevented, the long arm of the construct must be situated caudal to the site of translation, whereas if dorsal translation deformation is to be corrected or prevented, the long arm of the construct must be situated rostral to the site of translation (Fig. 26.6).

Four-point bending of the spine, as defined by White and Panjabi, involves loading a long structure (i.e., the spine) with two transverse forces on one side and two on the other (Fig. 26.7a). The bending moment is constant between the two intermediate points of force application if all forces are equal, whereas in three-point bending, the bending moment peaks at the intermediate point of force application (see Chapter 17 and Fig. 26.7a, b). If the forces applied by a three- or four-point bending construct are oriented in the opposite direction, the technique is termed reversed three-point or reversed four-point bending fixation (Fig. 26.8). This technique may be used to reduce lumbar spondylolisthesis.


The forces applied to the various components of an implant must be carefully considered. For example, a screw can be exposed to significant three-point bending forces. This must be considered during the implant design decision-making process (Fig. 26.9).

Crossed-Rod Deformity Correction
The crossed-rod technique is a well-established method of thoracic and lumbar kyphotic deformity correction. It was first used with Harrington distraction rods (Fig. 26.10a); it was later employed more effectively with multisegmental sublaminar wiring (Luque) techniques (Fig. 26.10b). 1 Most recently, it has been most effectively used via the sequential hook insertion (SHI) technique with universal spinal instrumentation systems (Fig. 26.10c). 2 It can also be used to correct coronal plane deformities ( Fig. 26.10d). Creative adjuncts may also be used (Fig. 26.10e). Regardless of the construct type, the technique involves the simultaneous application of deformity reduction forces to the spine by means of moment arms (longitudinal members; i.e., rods). Gradual reduction is thus achieved via a three-point bending force application mechanism (Fig. 26.11).


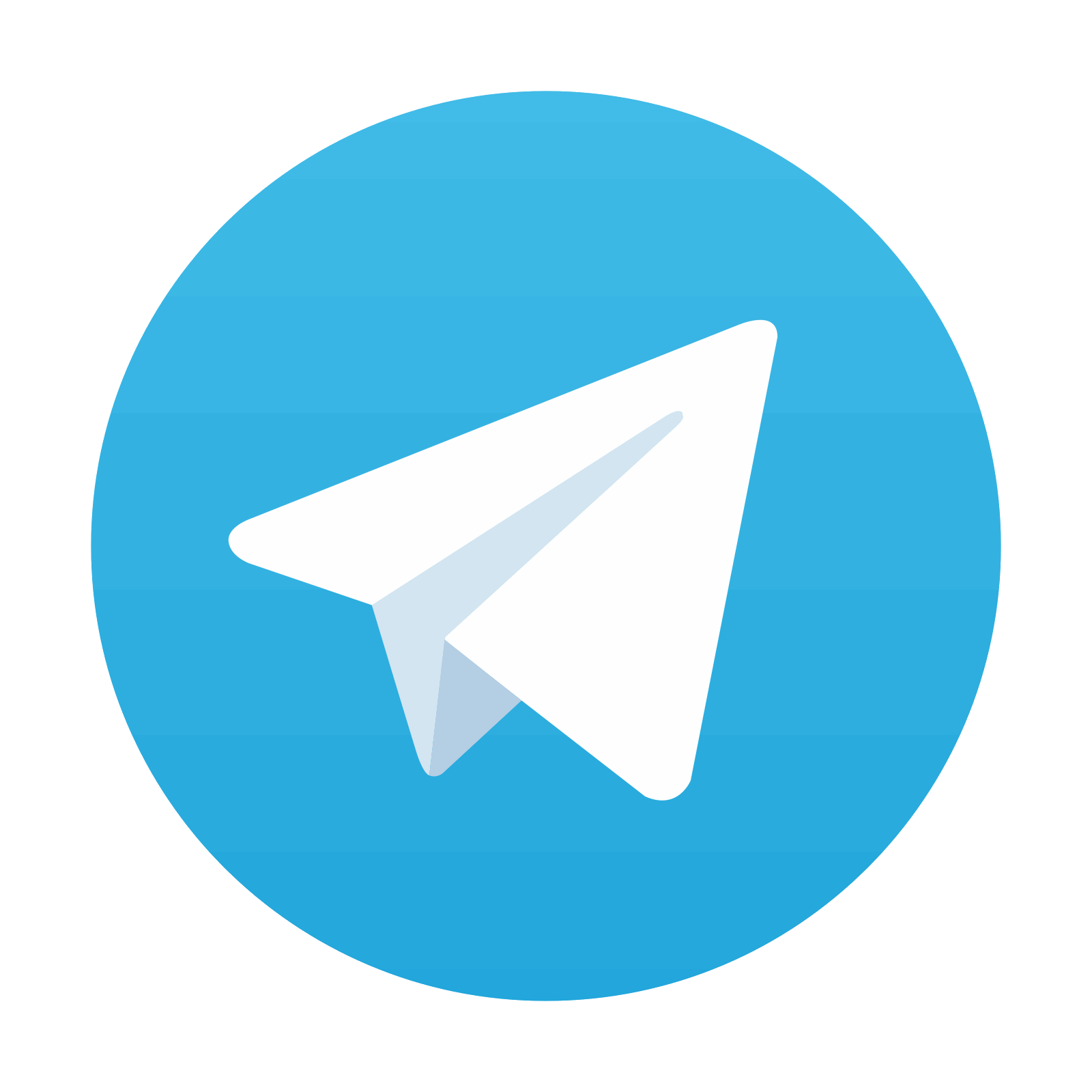
Stay updated, free articles. Join our Telegram channel

Full access? Get Clinical Tree
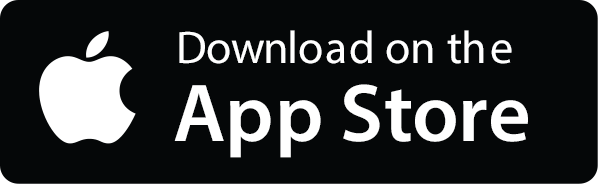
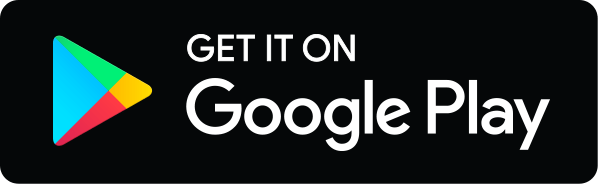
