26 Electrophysiological Monitoring
Progress in operative techniques has allowed the spine surgeon to treat patients with increasingly complex spinal deformities. Correcting more complex deformities requires sophisticated intraoperative neuromonitoring (IONM) techniques, which facilitate the detection and prevention of potentially devastating iatrogenic neurological injury. This chapter discusses the advantages and limitations of the various IONM tools available to the deformity surgeon.
The reported incidence of spinal-cord injury in scoliosis surgery varies from 0.3 to 1.4%.1–3 Surveying the Scoliosis Research Society (SRS) database, MacEwen et al reported an incidence of spinal-cord injury of 0.72%, with complete paraplegia occurring in 55% of these cases.3 A variety of mechanisms may account for spinal-cord injury during corrective scoliosis surgery. Misdirected wires, hooks, or pedicle screws may cause direct trauma to the cord. The correction of scoliosis may distract the spinal cord and compromise the local blood supply.4 Similarly, occlusion of segmental vessels during anterior procedures may cause ischemia of the cord.5,6 A decreased mean arterial blood pressure (MABP <60 mm Hg) and a low hemoglobin concentration exacerbate these tenuous situations.7
Clinical Tests of Global Spinal Function
Since its description in 1973 by Vauzelle and colleagues, the Stagnara wake-up test has been a widely used tool for assessing neurological function intraoperatively.8 The test involves a temporary reduction in anesthesia during which the patient is instructed by verbal command to move the extremities, starting with the upper extremities and progressing to the lower extremities. Failure to move the lower extremities symmetrically indicates a neurological injury. The benefit of the test is that it is a simple, cost-effective procedure that can be performed in any operating room, and does not require the participation of a specialized team of trained neurophysiologists. Furthermore, it provides a direct measure of global motor function. Although some still consider the wake-up test the “gold standard” for the intraoperative assessment of neurological function, it has significant shortcomings. Potential complications related to the wake-up test include patient recall, accidental extubation, air embolism, pain, construct-rod dislocation, disruption of intravenous and intra-arterial lines, added surgical time (between 30 and 45 minutes), and false-negative and false-positive results.9,10
Hoppenfeld and colleagues described the ankle clonus test in 1997 as a predictor of neurological compromise following scoliosis surgery.11 They reported that the absence of bilateral ankle clonus on emergence of the patient from anesthesia is abnormal and indicates neurological injury. The ankle clonus test is predicated on the normal presence of bilateral ankle clonus upon recovery from general anesthesia as a result of the return of lower-motor-neuron function before the return of inhibitory upper-motor-neuron impulses, leading to a temporary excitatory state. In Hoppenfeld’s review of 1006 patients who underwent spinal arthrodesis and instrumentation for scoliosis, 6 patients had new neurological deficits postoperatively, all of whom had a “positive” ankle clonus test. There were three false-positive findings but no false negatives.9,11 Although this method of monitoring spinal-cord function is cheap, sensitive, and easily performed, some have questioned its specificity in the setting of inhalational anesthesia.12
The major drawback of both the wake-up test and ankle clonus test, however, is their failure to provide a real-time and continuous assessment of spinal-cord integrity. Both tests reflect global spinal integrity and cannot provide a realtime assessment of dorsal sensory or ventral motor cord tracts. This lag between injury and its detection (between 30 and 45 minutes in the wake-up test) may jeopardize the small window of opportunity for intervention, resulting in a transient deficit becoming permanent. Such limitations have served as the impetus for the development of IONM, which provides a real-time (and sometimes instantaneous) indication of spinal-cord integrity in patients undergoing corrective scoliosis surgery. Advances in neurophysiological monitoring of the spinal cord have complemented improvements in corrective spinal instrumentation and design, and together the two facilitate the improved care that can be offered to patients with more complex spinal deformities.
Monitoring of Somatosensory Evoked Potentials
Although the monitoring of somatosensory evoked potentials (SSEPs) was first described almost 70 years ago, its use in clinical practice was first reported by Nash and colleagues in 1977 for detecting impending neurological injury during scoliosis surgery.13,14 Despite the advent of more sophisticated monitoring modalities, monitoring of SSEPs is still considered by some to be the “gold standard” against which all other IONM techniques should be compared.

SSEP monitoring represents the averaging of electrical responses to repetitive electrical or mechanical stimulation of a peripheral nerve, most commonly the posterior tibial or peroneal nerves. The spinal cord is the conduit through which the afferent volley travels, largely reflecting the integrity of the dorsal sensory columns of the cord. Signal transduction is mediated by large-diameter myelinated sensory fibers that traverse the peripheral nerve and then enter the spinal cord and ascend through the dorsal columns ( Fig. 26.1 ). After synapsing in the medullary nuclei of the brainstem, the neural signal then crosses the brainstem and enters into the medial lemniscal pathway. After another synapse in the thalamic nuclei, the signal proceeds to the parietal (sensorimotor) cortex. The afferent signal is then recorded either at the level of the spinal cord or, more commonly, the scalp ( Fig. 26.2 ). Monitoring of the upper extremities at the brachial plexus is best done by stimulating SSEPs in the ulnar nerve ( Fig. 26.3 ).15,16 Monitoring of SSEPs in the lower extremities is done by stimulating the posterior tibial nerves or peroneal nerves ( Fig. 26.4 ).17,18
SSEP monitoring has several distinct advantages over the ankle clonus and wake-up tests for spinal-cord integrity. It is highly effective in reducing the rate of neurological injuries below the rate found without IONM; it can detect injuries at the time of their occurrence rather than after the fact; and it can be performed on patients who are neurologically intact and those who are compromised.3,19,20 Although still commonly done in conjunction with the wake-up test, SSEP monitoring can prevent the well-known complications of the wake-up test. Numerous studies have shown the efficacy of SSEP monitoring in reducing the rate of new neurological deficits in surgery for scoliosis.21–23 As compared with the results obtained by MacEwen et al, without intraoperative monitoring was utilized, Nuwer and associates found that SSEP monitoring was effective at reducing the rate of major deficits of new-onset by ~60%.3,20 In their survey of 51,263 scoliosis procedures, the latter investigators reported that SSEP monitoring had a sensitivity of 92%, although it also had a tendency toward giving a relatively high rate of false-positive results. In this large series, the primary predictors of deficits of new-onset were the experience of the neurophysiology team followed by that of the surgeon. Overall, the rate of false-positive results with the use of SSEP monitoring averages 2%, with a reported range of 0 to 7%.24–27 In 1992, the SRS issued a position statement advocating SSEP monitoring as the standard of care for scoliosis surgery.28 A recent survey of 37 members of the Spinal Deformity Study Group of the SRS revealed that the mode of neuromonitoring in surgery for adolescent idiopathic scoliosis (AIS) varied on a case-by-case basis, with SSEP monitoring alone being used in 10% of cases, the wake-up test in 1.1%, and no IONM in 0.6%.29 These results confirm the persistence of wide variation in IONM practice patterns in AIS surgery ( Table 26.1 )



Despite its excellent clinical track record, SSEP monitoring has limitations that have recently become of growing concern among spinal-deformity surgeons.21,30–34 Because SSEP monitoring primarily assesses the integrity of the dorsal sensory columns of the spinal cord, primary motor injuries, usually reflecting ischemia of the ventral motor tracts of the cord from hypotension, distraction, or derotation, may go undetected. Pelosi and co-workers reported a false-negative rate of 2.4% in monitoring of SSEPs, as compared with no false-negatives in monitoring of transcranial electrical motor evoked potentials (tcMEPs).33 Others have similarly reported a failure to detect deficits of new onset with SSEP monitoring.10,21,30–38 In the largest series to date to compare SSEP monitoring with tcMEP monitoring in AIS, Schwartz et al found that SSEP monitoring failed to detect four of seven cases (57%) of new-onset motor deficits, whereas tcMEP monitoring detected all seven cases.34
Type of IONM Utilized | No. of Cases (%) |
No form of IONM | 6 (0.6) |
Wake-up test only | 11 (1.1) |
SSEP only | 100 (10) |
tcMEP only | 6 (0.6) |
Combined SSEP and tcMEP | 436 (44) |
Combined SSEP, tcMEP, and wake-up test | 196 (20) |
SSEP, MEP, and EMG | 233 (24) |
Total | 988 |
Source: Auerbach JD, Diab M, Sanders JO et al. Variability in spinal cord monitoring practice patterns in adolescent idiopathic scoliosis. Proceedings of the 14th Annual International Meeting on Advanced Spine Techniques, Paradise Island, Bahamas, July 2007. Adapted with permission.
Abbreviations: IONM, intraoperative neuromonitoring; EMG, electromyography; SSEP, somatosensory evoked potential; tcMEP, transcranial electrical motor evoked potential
Besides its weaker sensitivity in detecting impending neurological injury, monitoring of SSEPs shows a well-documented delay in detecting ischemic injury as compared with monitoring of tcMEPs. Schwartz and associates showed that the average time to detection of an intraoperative insult with SSEP monitoring lagged behind that with tcMEP monitoring by an average of 5 minutes, with the lag ranging from instantaneous to 10 minutes.34 This relative delay in detecting ischemia with SSEP monitoring has also been reported in the literature on thoracoabdominal aneurysm (TAA), and as a result, the technique has been abandoned for the prevention of neurological injury in surgery for TAA.35,37 There are two potential explanations for the delay in detecting ischemia with SSEP monitoring: (1) monitoring primarily of the dorsal sensory columns allows ischemia of the ventral cord and motor tract only to be inferred rather than measured directly, in contrast to monitoring of tcMEPs, which correlates directly with the blood supply to the ventral cord; and (2) unlike tcMEP impulses, SSEP impulses relay afferent sensory signals nonsynaptically, which, because of the relative resistance of axonal conduction to ischemia, may result in a lag between the onset of an ischemic injury and detection of the causative ischemic event.35,39 When these factors are taken together, they show that SSEP monitoring alone is incapable of direct monitoring of vascular blood flow to the ventral cord in real time, and therefore at best serves as an indirect measure of the global ventral-cord blood supply.
With the improved sensitivity afforded by the monitoring of tcMEPs, a residual role of SSEP monitoring of the spinal cord during AIS surgery may be to confirm the integrity of the cord in the setting of unchanged tcMEPs.33,34 One critical and unique contribution of SSEP monitoring, however, is in monitoring of blood flow to the brachial plexus during the prone positioning of patients.40 Prolonged or inappropriate prone positioning is an increasingly recognized source of iatrogenic neurological injury to both extremities in scoliosis surgery. With the arm in abduction and with increasing axillary pressure, brachial plexopathy can occur. Typically, ulnarnerve SSEPs are recorded immediately after positioning of the arm in the flexed, abducted position. The point prevalence of positional brachial plexopathy reported in recent studies has ranged from 3.6 to 15%, depending on the criteria used for a significant change.15,16,40 A reduction in SSEP amplitude of 30% indicates an impending injury and should prompt the surgical team to reposition the patient’s arm15,16,41 In 18 arms for which there was an intraoperative SSEP alert, repositioning resulted in a nearly immediate return of ulnar-nerve SSEP tracings to baseline and in normal neurological function upon awakening of the patient ( Fig. 26.1 ).16 Another study demonstrated that SSEP monitoring was 78% sensitive in detecting sensory deficits in the upper extremity, 100% sensitive in detecting combined sensory and motor deficits, and 98.5% specific in predicting a normal neurological status postoperatively.40 For routine AIS surgery, the continued use of SSEP monitoring is recommended because of its confirmatory role in the setting of negative tcMEPs, for providing information on the dorsal sensory cord, and for its ability to monitor the brachial plexus during prone positioning of the patient.

Monitoring of Transcranial Electrical Motor Evoked Potentials
Transcranial electrical stimulation of the motor cortex generates an electrical impulse that descends the corticospinal tract (CST) and, at the distal end of the signal volley, enters the peripheral muscle in which this electrical impulse, or tcMEP, is ultimately recorded. Beginning with a transcranial electrical stimulus that delivers a brief (50 μsec), high-voltage (250 to 500 V) train of anodal pulses (two to seven pulses, with an interstimulus interval of 1 to 5 msec), a neuroelectric signal is generated ( Fig. 26.5 ). Axons in the CST mediate the electrical impulse, which travels from the cortex through the internal capsule to the caudal medulla, where the fibers of the CST decussate and form the lateral CST. The signal then descends the CST in the lateral and anterior funiculi of the spinal cord. Upon entering the gray matter of the spinal cord, the axons of the CST interact with the interneurons of the spinal cord and synapse with α? motor neurons. The subsequent electrical responses are recorded at peripheral muscles, most commonly the anterior tibialis, although recording at the abductor hallucis, iliopsoas, quadriceps, and foot flexors has been described ( Fig. 26.6 ).42 The first dorsal interosseous muscle is also monitored as a control, to assess whether or not an intraoperative tcMEP alert represents global spinal-cord dysfunction (reflected by reductions in the amplitude of tcMEPs in both the upper and lower extremities) or localized injury (in which only tcMEPs in the lower extremity are affected). Once baseline signals are obtained, there are no absolute standards about what constitutes an alert. Some authors advocate ≥50% decrease in unilateral or bilateral tcMEP amplitude as the criterion for an alert, whereas others use an 80% decrease in amplitude.2,33 In our institution, a tcMEP alert is defined as a sustainable decrease in tcMEP amplitude of 65%.

The neurophysiological mechanism of signal transduction is probably responsible for the preferential sensitivity of tcMEP over SSEP monitoring in the detection of motor deficits during scoliosis surgery. Although SSEPs in the lower extremity provide information about the sensory dorsal columns, they do not reflect the integrity of the CSTs. The sensory dorsal columns consist of nonsynaptic axonal white matter, which is inherently more resistant to ischemia than is gray matter.39,43 On the other hand, motor neurons in the anterior horn of the gray matter of the spinal cord have a high metabolic rate and are exquisitely vulnerable to ischemic changes.35,39 TcMEPs, which are mediated by synapses in the ventral spinal cord, are thus highly sensitive to localized ischemic changes affecting the motor cells and interneurons of the anterior horn of the spinal cord. This is of particular clinical relevance because the vascular supply to the ventral motor pathways is less redundant than that to the posterior sensory columns, making the ventral motor tracts highly vulnerable to cord ischemia. During scoliosis surgery, hypotension and corrective maneuvers such as distraction and derotation may further compromise the local blood supply to the spinal cord.10,30,33 On the basis of its sensitivity to ischemic changes affecting the ventral cord, tcMEP monitoring serves as a real-time neurophysiological indicator of impending injury to the ventral motor tract ( Table 26.2 ).31,34,35,38
Cause of Intraoperative Alert (n = 38) | |
Distraction/derotation | 16/38 (42%) |
Hypotension only (MABP <60 mm Hg) | 9/38 (24%) |
Passing/tightening of sublaminar wires | 5/38 (13%) |
Thoracic hook | 4/38 (11%) |
Segmental vessel clamping | 3/38 (8%) |
Thoracic pedicle screw | 1/38 (3%) |
Source: Schwartz DM, Auerbach JD, Dormans JP et al. Neurophysiological detection of impending spinal cord injury during scoliosis surgery. J Bone Joint Surg Am 2007;89:2440–9. Adapted with permission.
Abbreviation: MABP, mean arterial blood pressure
Numerous studies document the efficacy of tcMEP monitoring for detecting impending neurological injury during scoliosis surgery.2,33–36,38,41,44 Schwartz et al reported the largest series of scoliosis surgeries in which tcMEP monitoring was used.34 They retrospectively reviewed the intraoperative records of 1121 patients with AIS. All four participating pediatric spine centers adhered strictly to optimal anesthesia protocols and used the same team of highly trained, experienced neurophysiologists. Seventeen patients experienced depression of tcMEPs >65% (alert level) without any changes in SSEPs. Seven patients (0.6%) in the 1121-patient cohort experienced transient motor deficits, all of which were identified during surgery and were met with appropriate and timely intervention. No patient sustained a permanent neurological deficit, possibly because of the rapid detection of neurological compromise and subsequent appropriate intervention at a point when the impending injury was still reversible. All neurological deficits resolved within 90 days, and most (six of nine cases) resolved within 7 days.34 Other studies have confirmed the efficacy of tcMEP monitoring during scoliosis surgery.2,33,38
The issue of false-positives in IONM deserves special mention. A perceived limitation of spinal-cord monitoring is the relatively high rate of false-positive readings in some series. As a result of an IONM alert, the team response (i.e., a pause in surgery, raising of the patient’s systemic blood pressure, reduction in the degree of spinal correction, administration of steroids, or removal of hardware) when the postoperative neurological examination is normal can be frustrating and perhaps perceived as unwarranted, and may be a source of significant risk to the patient. Traditionally, the outcome variable that is assessed in evaluating the efficacy of spinal-cord monitoring is whether or not the patient awakens with a neurological deficit of new onset (a true positive). According to these criteria, a false-positive alert is therefore defined as an intraoperative alert in which a patient has a normal neurological examination upon awakening. We would argue, however, that an intraoperative neurophysiological alert, especially in tcMEP monitoring, indicates a true physiological event affecting the spinal cord (i.e., ischemia), and has the potential to become a neurological deficit if not addressed acutely. The bases for this are numerous animal and clinical studies demonstrating the relationship between spinal-cord ischemia (a true physiological event), a significant tcMEP alert, and the onset of new neurological injuries.35,45,46 A recent study showed that up to 74% of tcMEP alerts may be attributable to a mechanism of spinal-cord ischemia arising from systemic hypotension, distraction, or derotation maneuvers, in 32% of which patients awakened with a neurological injury of new onset.34 It can therefore be concluded that the ischemic cord is “at risk” for permanent injury and that a tcMEP alert provides an opportunity to correct a threatening neurological deficit.31,33,34,38 Although it is difficult to prove in a controlled study, false-positive tcMEP readings are likely to represent a true positive made “false” by appropriate and timely intervention.
Electromyography
Intraoperative evaluation of the integrity of the pedicle and pedicle-screw position before and after screw placement is a three-step procedure, involving: (1) manual palpation of the ventral, medial, lateral, superior, and inferior pedicle walls with a pedicle probe or feeler; (2) intraoperative imaging47; and (3) electromyographic (EMG) stimulation.
In more traditional hook or hybrid constructs, EMG monitoring is used only during lumbar or lower-thoracic pedicle-screw placement. More recently, however, thoracic pedicle-screw constructs have become more common as numerous clinical and biomechanical studies have shown improved rotational correction, improved fixation, and reduced loss of correction with their use as compared with hook or sublaminar wire constructs.48–51 Given the smaller pedicle diameter in the thoracic spine, the variable pedicle angle, and the proximity of the great vessels, thoracic spinal nerve roots, and spinal cord, the risk of a pedicle breach and injury of adjacent structures is greater than in the lumbar spine.52–55 Consequently, there is growing interest in studying the capabilities of EMG monitoring as a means of improving patient safety during the placement of both lumbar and thoracic pedicle screws.52,53
Earlier cadaveric studies revealed a rate of 12.5 to 54.7% of thoracic cortical violation with thoracic pedicle-screw placement56–60 More recently, a cadaveric study in which the freehand technique was used to place thoracic pedicle screws along the anatomic axis demonstrated a 97% rate of success in screw placement with less than 1 mm of pedicle-wall violation. In addition, 87.5% of the screws inserted were fully contained within the pedicle.57 In the largest clinical study of its kind to date, Lehman and coworkers used postoperative computed tomography (CT) to evaluate the thoracic positioning of of 1023 pedicle screws placed in 60 patients with spinal deformity through the freehand technique. They reported a 91.2% rate of success, without any neurological, vascular, or visceral complications.61 Reported clinical sequelae of erroneous pedicle-screw placement include incidental durotomies, nerve-root irritation, pedicle fracture, and leakage of cerebrospinal fluid (CSF).52,53,62
EMG monitoring is done by placing subdermal needle electrodes into the muscle groups innervated by the spinal nerves relevant to a surgical procedure. For the cervical and lumbar spine, well-established EMG recording sites that correspond with the nerve roots at risk have been described ( Table 26.3 ). For monitoring thoracic pedicle-screw placement in the upper spine (T2 to T6), electrodes are placed at the corresponding intercostal spaces at the nipple line, and compound muscle action potentials (CMAPs; electrical potentials evoked by stimulation of the motor nerve innervating a specific group of muscles) in the intercostal musculature are assessed. For monitoring the placement of screws in the lower thoracic spine (T7 to T12) according to the method described by Shi and coworkers, paired electrodes are placed along the nipple line at evenly spaced intervals between the lower margin of the 10th rib and the iliac ridge ( Fig. 26.7 ).53 In most instances of monitoring of lower thoracic screw placement, CMAP activity is assessed in the rectus abdominus musculature.52,63
Source: Padberg AM. Electrophysiology. In: DeWald RL, Arlet V, Carl A et al, eds. Spinal Deformities: The Comprehensive Text. New York: Thieme, 2004:135–48. Adapted with permission.
The regimen in EMG monitoring consists of two components: (1) monitroring of spontaneous EMGs (spEMGs); and (2) monitoring of stimulated EMGs (stEMGs). Monitoring of spEMGs involves the continuous acquisition of data from spontaneous muscle activitys at rest. With chronic nerve-root compression, impulse-train activity is the most common pattern seen in spEMG recordings. More acute changes in spEMG activity may manifest in the form of burst or train activity resulting from mechanical stretching, retraction, or sudden compression of a nerve root. The major contribution of spEMG monitoring is its ability to instantly notify the surgical team about a nerve-root insult. It does not, however, provide information about the conductive capacity of the nerve root after insult or injury. The frequency of postoperative neurological injuries following spEMG alerts is low, indicating a low specificity for this technique.63,64

stEMG monitoring involves the electrical stimulation of a pedicle channel or screw and the recording of the stimulation threshold needed to trigger a CMAP.63 The stimulating probe is placed into the pedicle-screw channel or on the surface of the pedicle screw, and a threshold potential for stimulation of a CMAP is recorded. The rationale behind stEMG monitoring is that the electrical stimulus required to activate the neighboring nerve root is proportional to the electrical impedance characteristics of the pedicular bone. If the pedicle is intact, the greater resistance to current flow would result in a high threshold for screw stimulation (i.e., >15 mA). If there is violation of the pedicle, however, the resistance to current flow is reduced and the screw-stimulation threshold will be lower (i.e., <3mA), indicating damage.41,52,53,65 Studies have confirmed a significant correlation between low pedicle-screw stimulation thresholds and misdirected placement of lumbar pedicle screws52,53,62,66,67
It is important to maintain proper technique when performing stEMG to avoid false recorded values. Steps should be taken in every case to: (1) ensure that no soft tissue is in contact with the EMG probe, which can artificially increase the stimulation threshold (i.e., give a false-negative result); and (2) stimulate circumferentially inside the pedicle and vertebral body, being aware that in patients with osteoporosis or poor bone quality a low impedance value (from diminished resistance to the applied electrical current) can be seen despite the pedicle being intact (i.e., a false-positive result)68; (3) apply the cathode stimulator probe to the hexagonal screw port or directly to the shank of the pedicle screw, and not to the mobile crown, to avoid a false-negative EMG result ( Figs. 26.8 , 26.9 ).69 Anderson et al recently reported that polyaxial pedicle screws can have high electrical resistances between their mobile crowns and shanks, and may therefore fail to produce a response during stEMG testing despite the presence of a pedicle breach.
The clinical results with triggered EMG for monitoring the placement of lumbosacral pedicle screws have been excellent.41,52,66,70 Overall, the negative predictive value of lumbar pedicle-screw monitoring with stEMG has been 98% when the impedance values have been ≥11 mA.53 If the threshold of stimulation is low (<6 to 7 mA), a pedicle breach is more likely. Impedance values between 7 and 11 mA represent a possible pedicle breach.66,67 In results resembling those in the lumbar spine, Shi et al recently reported that stEMG for monitoring pedicle-screw placement in the thoracic spine had a negative predictive value of 98% when stimulation thresholds were >11 mA.53 Raynor and associates reported that all screws with stimulation thresholds >6 mA were safely implanted, without breaching of the medial pedicle wall. For screws with a stimulation threshold of <6 mA, however, Raynor et al recommend concomitant evaluation of the “average” of all other screw-stimulation thresholds in a given patient, and suspicion of a pedicle-wall breach only if the threshold is 60 to 65% below the patient’s average threshold.52 In a later study, Raynor et al analyzed the EMG results for the placement of 4857 lumbar pedicle screws, and established 8.0 mA as a threshold above which the stimulated screw was very likely to be present in an intact pedicle (a 0.33% false-negative rate). At lower thresholds of stimulation, Raynor and colleagues recommended removal of the relevant screw and repeated palpation to evaluate for a pedicle breach.71 Certainly the higher the threshold of EMG stimulation the less likely a pedicle is to have been breached, but using a higher threshold of stimulation as the criterion for repeat palpation carries the risk of increaseing the frequency of false-positive results and unnecessary repeat palpations.

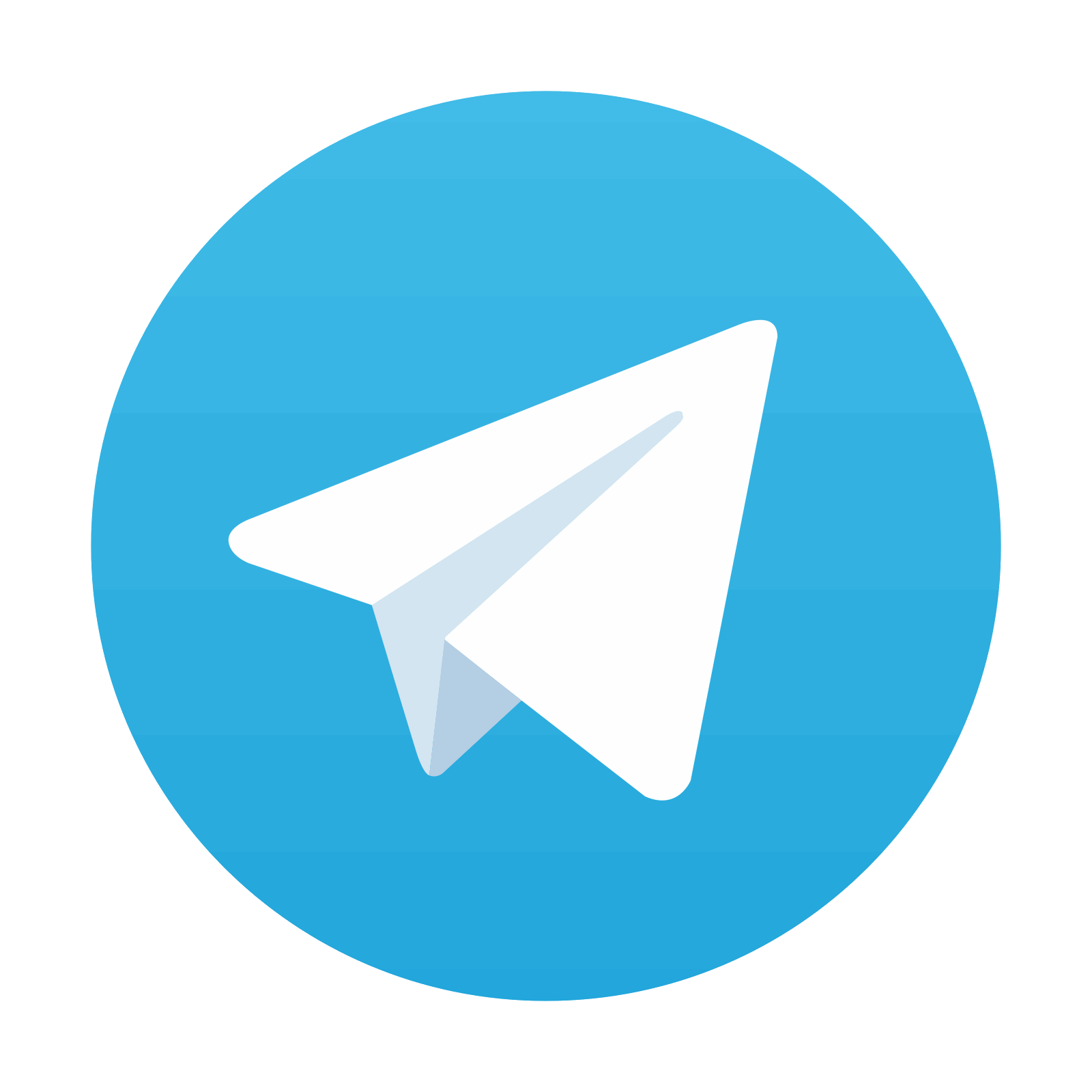
Stay updated, free articles. Join our Telegram channel

Full access? Get Clinical Tree
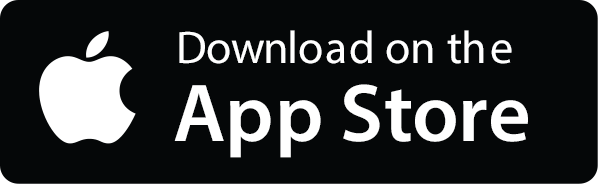
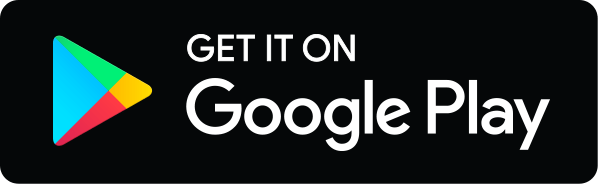