27 Deformity Prevention and Correction: Complex Clinical Strategies
The component strategies addressed in Chapter 26 are often effective for simple deformities. However, more complex pathologies may require a combination of often complex component strategies. Before a surgeon can apply these component strategies in a complex manner, an understanding of deformity and deformity correction fundamentals is necessary. The indications for deformity correction are numerous. They include neurologic improvement and the prevention of neurologic decline, further deformation, and secondary spinal cord changes. 1
27.1 Fundamentals
Spinal deformities can be divided into three fundamental categories: (1) sagittal plane deformities (e.g., kyphosis, translation); (2) coronal plane deformities (e.g., scoliosis, translation); and (3) axial plane deformities (e.g., rotation; see Chapter 7 and Fig. 27.1a). From a balance perspective, Dubousset 2 described the “cone of balance” or rather the “cone of economical function” (Fig. 27.1b). This cone defines the limit of tilt or “tipping point” parameters, if you will. It describes a range of deformations or postures that can be tolerated without “tipping” or without the expenditure of an exceptional amount of energy to prevent “tipping.” Hence, at the periphery of the cone, outside the stable zone, significant energy is expended to maintain posture—and is often associated with pain.

Treatment strategies for deformities in each of the aforementioned planes differ. Rotational deformities often do not require a separate or isolated attempt at correction. However, many deformities are complex, with a combination of sagittal, coronal, and axial plane components. For example, a lumbar degenerative scoliosis nearly always is associated with a rotational and kyphosis component. It might therefore be more appropriately termed a “degenerative rotatory kyphoscoliosis.” This term implies that a kyphosis is present. In this case, kyphosis is relative. A loss of lordosis, in the aforementioned context, can be thought of as a kyphosis. In many clinical situations, only a loss of lordosis is present. Therefore, only a relative kyphosis (loss of lordosis) exists (Fig. 27.2).

The coupling phenomenon explains in part the rotational and kyphosis components that are secondary to the scoliosis component of a degenerative lumbar curve (see Chapter 2). A scoliotic curvature causes deformation of the spine so that the axis through which most of the axial load is borne (neutral axis) is shifted by spine deformation toward the concave facet joints (Fig. 27.3a). This has been observed in long-term follow-up studies. Degenerative lumbar scoliosis appears to be triggered by disc degeneration, with the loss of segmental lordosis and accompanying segmental wedging in the coronal plane. Such deformities can progress or even uncommonly retrogress. 3 This is termed off-axis deformation. The “new” axis is not loaded in the midsagittal plane, as is the case in a normal spine. It is now loaded off axis. In the lumbar spine, this results in a relative dorsal migration of ventrally situated vertebral bodies with respect to their normal position. This causes a loss of lordosis (relative kyphosis), particularly in the standing position (Fig. 27.3b). 4 , 5 Therefore, both the rotatory and sagittal plane deformations are secondary, at least in part, to the scoliotic component (Fig. 27.3c). In a sense, the affected portion of the spine is swung off axis (out of the sagittal plane) in a manner similar to the swinging of a bucket handle that is held upside down (Fig. 27.3d). With this analogy, the bucket handle swings “off axis,” while the grip rotates with the handle. Therefore, a rotatory scoliosis is manifested because of the off-axis position of the spine. In addition, a loss of lordosis occurs because the bucket handle moves relatively dorsally as it rotates off axis. Hence, the curvature is correctly termed a rotatory kyphoscoliosis.

To bring these off-axis deformations back into alignment, concave side distraction or convex side compression alone will not completely correct the complex off-axis deformity, particularly if facet joint integrity is disrupted by the surgery (Fig. 27.4). Such disruption removes the mechanism by which the rotational deformity occurred in the first place (facet engagement resulting in the coupling phenomenon; see below). It then eliminates the mechanism via which facet engagement could be used to correct the deformity. The surgeon should consider the mechanisms or causes of the deformation and strategies for their reversal during strategic planning and the deformity correction process. 6

A reversal of the mechanisms that caused the deformity is often an appropriate therapeutic strategy. The surgeon must keep in mind, however, that complete deformity correction is not usually necessary in order to alleviate the patient’s symptoms and to “attain and maintain” a nonpathologic relationship between the neural elements and their bony and soft tissue confines. Nevertheless, complex multiple-axis deformities can, at least in part, be corrected by using the derotation maneuver in combination with other strategies. This approach essentially reverses and neutralizes the pathology.
The combination of concave side distraction and convex side compression (see Fig. 27.4) and sagittal plane correction strategies (i.e., the crossed-rod technique and “in situ” rod bending) and the derotation maneuver (Fig. 27.5) can be used, usually in combination, to address the vast majority of spinal deformities (see Fig. 27.4 and Fig. 27.5).

27.2 Principles of Deformity Correction
Applying the basic deformation correction component forces (distraction; three-point bending; tension-band fixation; and fixed, nonfixed, and applied moment arm cantilever beam fixation) to the spine, either alone or in combination, provides a variety of mechanisms for simple and complex deformity correction. Five additional factors, however, must also be considered.
First, spine deformations are frequently multisegmental. In these situations, the strategies outlined above must be applied to multiple spinal segments. This complicates the deformity correction process.
Second, the coupling phenomena (see Fig. 27.5 and Fig. 27.6), which results in off-axis complex deformities, often complicates the situation. A motion (or deformation) that occurs along or about an axis of the Cartesian coordinate system often obligates another motion (or deformation) along or about another axis. This is a manifestation of, and in fact defines, the coupling phenomenon. In the coupling phenomenon, a motion along or about an axis of the Cartesian coordinate system obligates another motion along or about another axis. Perhaps the most common and graphic clinical example of this multiplanar phenomenon is degenerative rotatory kyphoscoliosis. Remember, kyphosis is included in this term because of the relative loss of lumbar lordosis (straightening of the lumbar spine). The rotational component of the deformity, as well as the relative kyphosis, is coupled (over several segments) with the scoliosis (see Fig. 27.6). Thus, a correction of this deformity should ideally include a consideration of each of these three deformations. Of note, the orientation of rotation (clockwise vs counterclockwise) is different in the cervical and lumbar regions. With a left cervical bend, the spinous processes rotate to the right. Conversely, with a left lumbar bend, the spinous processes rotate to the left (see Fig. 27.6).

Third, the manner in which the spine bears loads, particularly axial loads, is of significant relevance. The surgeon should consider the load-bearing axis (neutral axis) in this regard (Fig. 27.7a). This axis essentially defines the region of the spine that bears the predominance of the axial load. It should be considered in both the sagittal and coronal planes. This axis cuts corners, as a race car might do while negotiating a serpiginous course. In the normal cervical and lumbar spine, the load-bearing axis is located in the dorsal region of the vertebral bodies. In the normal thoracic spine, it is located in the region of the vertebral bodies (see Fig. 27.7a). This is exaggerated if the spinal contour is exaggerated. For example, in extension, the load-bearing axis passes even farther dorsally in the cervical region, thus explaining why axial loads applied while the spine is extended result in significant dorsal element (facet joint) loading (Fig. 27.7b). The converse is also true (Fig. 27.7c). In patients with scoliosis, the concave facet joints are loaded excessively because the neutral axis is shifted toward the concave facet joints (Fig. 27.7d). It should be obvious that a consideration of the location of the neutral axis is relevant in both normal and pathologic spinal configurations.

Fourth, significant attention must be paid to the concept of spinal (sagittal and coronal) balance (Fig. 27.8). This is most often considered in the sagittal plane (sagittal balance). 4 , 5 , 7 – 9 The spine indeed seeks a balanced posture, with the maintenance of a generous, but not excessive, cervical lordosis, thoracic kyphosis, and lumbar lordosis (Fig. 27.8a). When a plumb line is dropped from the mid-C7 vertebral body in the standing position (sagittal vertical axis, or SVA) in the balanced spine, it falls through the region of the lumbosacral pivot point (dorsal L5–S1 annulus fibrosis region, or slightly dorsal to the sacral promontory; Fig. 27.8b). Other methods have been used to assess balance. 4 , 5 The SVA is assessed objectively by measuring its distance from the sacral promontory. A negative 2 to 4 cm (behind the promontory) is normal. As stated, this is the region of the lumbosacral pivot point, as defined by McCord et al. 10 This line usually moves forward with aging. A significant positive measurement, however, is clearly abnormal. If the normal contour of the spine is disturbed (Fig. 27.8c), compensatory mechanisms that adjust for aberrations in the spinal curvature may ensue. A loss of balance may also result, with the SVA falling in a very abnormal location with respect to the ventral S1 vertebra (Fig. 27.8d). 1 Of note is that the asymptomatic adult lumbar spine is less lordotic than that of adolescents. This corroborates the fact that lordosis naturally diminishes with aging 11 and that the SVA moves forward with aging. Kyphosis tends to occur with aging in the cervical, thoracic, and lumbar regions; hence, aging is kyphogenic. The loss of sagittal alignment and balance is exaggerated in patients with spondylolisthesis, who have a kyphotic lumbosacral angle. Of further note is that the progression of such deformities in patients with isthmic spondylolisthesis is exaggerated those with advanced degenerative changes. In this patient population, the SVA migrates significantly forward.
The center sacral line (CSL) can be used to assess balance in the coronal plane. In a scoliotic curve, the vertebrae bisected most closely by this line are defined as the stable vertebrae in the coronal plane (Fig. 27.8e). 1

Fifth, and finally, a consideration of implant length and location is critical. Implant length must be sufficient to apply the necessary bending moment to the spine. However, it must not be so long that it creates excessive spinal stiffness, over and above that expected. This is of relevance in both the rostral and caudal regions of the implant. In general, for long dorsal implants that are used for overt instability, three to four segmental levels rostral and two to three segmental levels caudal to the focal pathology are appropriately included in the construct (see Chapter 18). This is particularly relevant for hook–rod fixation and less relevant for screw–rod fixation strategies. Ventral thoracic and lumbar screw–rod fixation strategies do not require such length. Screw–rod constructs may be one to three segments shorter at each end. This, however, presents additional challenges, such as screw fracture caused by excessively loading of the screw.
McCord et al defined and characterized the lumbosacral pivot (Fig. 27.9). 10 This point, which is located at the dorsal annulus of the L5–S1 joint, defines the approximate location of the “normal” plumb line and also defines the point beyond which implants should gain the mechanical leverage required to adequately resist angular deformations at this juncture. Implants that pass ventral or caudal to this point provide significant mechanical advantage. 10

For both focal and gradual curves, a consideration of apical and neutral vertebrae (in the sagittal and coronal planes) is extremely important. The apical vertebra is the vertebra that is associated with the greatest segmental angulation at both its rostral and caudal disc interspaces, compared with all the other disc interspaces in the curve. It is located, in general, in the midportion of the curve, at the “horizon” of the curve (Fig. 27.10). The neutral vertebra is the vertebra that is associated with little or no angulation at its rostral and caudal disc spaces. It is the vertebra that is located between curves (Fig. 27.11).


The definition of the apical and neutral vertebrae (Table 27.1) should be determined by assessing the spine in both the coronal and sagittal planes (Fig. 27.12). In addition to the consideration of overall implant length, as previously discussed, an implant (construct) should usually not terminate at or near an apical vertebra (Fig. 27.13a). An implant, in general, should be long enough to extend to a neutral vertebra (Fig. 27.13b). The apical vertebra is the most critical vertebra in a curve, and as such, it is prone to deformation if a long moment arm (i.e., via an implant and/or fusion) is extended to that level (Fig. 27.13c). This is also true for coronal plane deformities (Fig. 27.13d, e). It is emphasized that both the apices and the neutral regions of a curvature may occur at a disc space, rather than at a vertebra. For simplicity, the vertebra that most closely approximates one of these locales is considered herein as the apical or neutral “location.” It is ideal to extend a fusion to both a neutral AND a stable vertebra (Fig. 27.13f). The stable vertebra is defined as the thoracic or lumbar vertebra that is positioned rostral (or caudal) to a scoliotic curve and that is most closely bisected by the central sacral line, assuming that the pelvis is level.


Angle of trunk inclination (ATI) | with the trunk flexed to the horizontal, the angle between the horizontal and the plane across the back at the greatest elevation of a rib prominence or lumbar prominence, as measured by an inclinometer (scoliometer) |
Apical disc | in a curve, the disc most deviated laterally from the vertical axis of the patient that passes through the sacrum, i.e. from the central sacral line |
Apical vertebra | in a curve, the vertebra most deviated laterally from the vertical axis that passes through the patient’s sacrum, i.e. from the central sacral line |
Apical vertebral/disc lateral deviation (from the global vertical axis) | distance in millimeters from the central sacral line to the midpoint of the apical body/disc (see Note 3). In a decompensated patient, the apical translation should be measured from the central sacral line for the thoracolumbar and lumbar spine, and from the C7 plumb line for the thoracic spine |
Café-au-lait spots | light brown or black irregular areas of skin pigmentation with smooth margins. If 6 or more are 1.5 cm or more in diameter, neurofibromatosis is suggested |
Central sacral line | the vertical line in a frontal radiograph that passes through the center of the sacrum (identified by suitable landmarks, preferably on the first sacral segment) |
Cervical scoliosis | scoliosis having its apex at a point between C1 and the C6-C7 disc |
Cervical-thoracic scoliosis | scoliosis having its apex at C7, T1, or the intervening disc space |
Chest cage asymmetry nonscoliotic | any clinical asymmetry of the posterior chest wall associated with a radiographic Cobb measurement of < 10° |
Chronologic definition of idiopathic scoliosis presentations | infantile scoliosis – presenting from birth through age 2+11 juvenile scoliosis – presenting from age 3 through age 9+11 adolescent scoliosis – presenting from age 10 through age 17+11 adult scoliosis – presenting from age 18 and beyond |
Compensation | the vertical alignment of the midpoint of C7 with the midpoint of the sacrum in the coronal plane (equates with the term coronal balance) i.e. horizontal distance of the C7 midpoint from the central sacral line |
Compensatory curve | a minor curve above or below a major curve that may or may not be structural |
Congenital scoliosis | scoliosis due to congenitally anomalous vertebral development |
Curvature measurement | Cobb method: angle between lines drawn on end plates of the end vertebrae (superior end plate of upper end vertebra; inferior end plate of lower end vertebra) |
Decompensation | compensation greater than a specified threshold value |
Double structural scoliosis | a spine with two structural scolioses |
Double thoracic scoliosis | a double structural scoliosis with the apex of each curve located in the thoracic spine |
End vertebrae | the vertebrae that define the ends of a curve in a frontal or sagittal projection cephalad end vertebra: the first vertebra in the cephalad direction from a curve apex whose superior surface is tilted maximally toward the concavity of the curve caudad end vertebra: the first vertebra in the caudad direction from a curve apex whose inferior surface is tilted maximally toward the concavity of the curve |
Fixed pelvic obliquity | pelvic obliquity which implies a nonidiopathic diagnosis, or an intrapelvic cause |
Gibbus | a localized kyphosis |
Hyperkyphosis | a kyphosis greater than the normal range |
Hypokyphosis | a kyphosis of the thoracic spine less than the normal range |
Hyperlordosis | a lordosis greater than the normal range |
Hypolordosis | a lordosis of the cervical or lumbar spine less than the normal range |
Hysterical scoliosis | a deformity of the spine that develops as a manifestation of a conversion reaction |
Idiopathic scoliosis | defined radiographically as a lateral curvature of the spine greater than or equal to 10° Cobb with rotation, of unknown etiology |
Iliac apophysis | the apophysis along the crest of the ilium |
Inclinometer | an instrument used to measure the angle of trunk cage inclination in the forward bend position |
Inflection vertebra | where curves change direction from convex to concave and vice versa |
Kyphosis | a posterior convex angulation of the spine. (For recommended measurement procedure, see Note 4.) |
Kyphoscoliosis | a nonidiopathic scoliosis associated with an area of true hyperkyphosis |
Lordoscoliosis | a scoliosis associated with an area of lordosis |
Lordosis | an anterior convex angulation of the spine. (For recommended measurement procedure, see Note 4.) |
Lumbar scoliosis | a scoliosis that has its apex at a point between the L1-L2 disc space through the L4-L5 disc space |
Lumbosacral scoliosis | a scoliosis that has its apex at L5 or below |
Major curve | the curve with the largest Cobb measurement on upright long cassette coronal x-ray of the spine |
Minor curve | any curve that does not have the largest Cobb measurement on upright long cassette coronal x-ray of the spine |
Myopathic scoliosis | a scoliosis due to a muscular disorder |
Neuromuscular scoliosis | a scoliosis due to either a neurologic or muscular disorder |
Neuropathic scoliosis | a scoliosis due to a neurologic disorder |
Neutral vertebra | a vertebra without axial rotation (in reference to the most cephalad and caudal vertebrae that are not rotated in a curve) |
Nonstructural curve | a measured curve in the coronal plane in which the Cobb measurement corrects past zero on supine lateral side bending x-ray |
Paravertebral height difference | with the trunk flexed to the horizontal, the difference in height (in millimeters) between two points on the posterior rib cage. The two points are identified as (1) the most prominent point (2) a point on the opposite side of the back, at an equal distance from the midline |
Pelvic inclination | deviation of the pelvic outlet from the vertical in the sagittal plane. Measured as an angle between the line from the top of the sacrum to the top of the pubis, and a horizontal line perpendicular to the lateral edge of the standing radiograph |
Pelvic obliquity | angulation of the pelvis from the horizontal in the frontal plane, possibly secondary to a contraction below the pelvis, e.g. of the hip joint. If this angulation is due to a leg length inequality, then the leg lengths should be equalized to create a level pelvis for measurement purposes |
Pelvic axial rotation | rotation of the pelvis in the transverse plane around the vertical axis of the body |
Radiographic plumb line | the vertical line drawn on a radiograph that is used to measure compensation |
Regional apical vertebral translation | distance in millimeters from the line joining the midpoints of the upper and lower end vertebrae of the measured curve to the midpoint of the apical vertebral body or disc |
Rib rotational prominence | the prominence of the ribs best exhibited on forward bending |
Risser sign | in the frontal plane x-ray of the pelvis, the state of ossification of the iliac apophysis used to denote the degree of skeletal maturity: 0-no evidence of ossification of the apophysis; 1–25% excursion; 2–50% excursion; 3–75% excursion; 4–100% excursion; 5-fusion of the apophysis to the iliac crest |
Sacral inclination | the angle between the line along the posterior border of S1 and the vertical lateral edge of a lateral standing radiograph (or between perpendiculars to these lines) |
Sacral obliquity | an angular deviation of the sacrum from the line drawn parallel to a line across the femoral heads on a supine AP view of the sacrum |
Sagittal spinal balance | alignment of the midpoint of the C7 body to the posterior superior corner of the sacrum on an upright long cassette lateral radiograph of the spine |
Scoliosis | a lateral curvature of the spine |
Skeletal age | the age obtained by comparing PA x-ray of the left wrist and hand with the standards of the Gruelich and Pyle atlas |
Stable vertebra | the thoracic or lumbar vertebra cephalad to a lumbar scoliosis that is most closely bisected by a vertically directed central sacral line assuming the pelvis is level |
Structural curve | a measured spinal curve in the coronal plane in which the Cobb measurement fails to correct past zero on supine maximal voluntary lateral side bending x-ray |
Thoracic scoliosis | a scoliosis that has its apex at a point between the T2 vertebral body through the T11-T12 disc |
Thoracogenic scoliosis | spinal curvature attributable to disease or operative trauma in or on the thoracic cage |
Thoracolumbar scoliosis | a scoliosis with its apex at T12, L1, or the intervening T12-L1 disc |
Vertebral axial rotation | transverse plane angulation of a vertebra; one method of measurement is with the Perdriolle technique (in degrees) |
Vertebral tilt | vertebral angulation to the horizontal in the coronal plane, measured from specified landmarks on the vertebra in a standing radiograph (normally lines drawn on the upper or lower end plate) |
Source: Used with permission of the Scoliosis Research Society: SRS Terminology Committee and Working Group on Spinal Classification Revised Glossary of Terms by the SRS Working Group on 3-D Classification and SRS Terminology Committee, March 2000. Note 1: Drawing curve (Cobb) measurements. The major curve should be measured first. It is normally measured from the superior end plate of the upper end vertebra to the inferior end plate of the lower end vertebra. Minor curves above or below the major curve will utilize the same upper or lower inflection vertebra. If the end plates cannot be visualized, then the inferior portion of the pedicles should be utilized as landmarks. In congenital curves, it may be necessary to draw a perpendicular line to the lateral aspect of the vertebral body to determine the upper and lower end vertebra tilt. Note 2: Radiographic plumb line. The preferred technique involves drawing a vertical line up from the midpoint of the sacrum and measuring the distance from this line to the C7 vertebral body center in millimeters. The alternate technique involves drawing a vertical line or dropping a plumb line from the C7 spinous process down to the sacrum, with the distance to the midpoint of the sacrum measured in millimeters. In both instances, it is assumed that the vertical edge of the radiograph is a true vertical. Note 3: The midpoint of a vertebra body/disc is determined by drawing a cross (X) in the body/disc. Draw a line from the upper left corner to the lower right of the body/disc and a line from the upper right to the lower left of the body/disc. The intersection is the midpoint. Note 4: The recommended measurement of thoracic kyphosis from a lateral radiograph is the angle between the superior end plate of the highest measurable thoracic vertebra, usually T2 or T3, and the inferior end plate of T12. The recommended measurement of lumbar lordosis from a lateral radiograph is the angle between the superior end plate of L1 and the superior end plate of S1. |
Finally, the cervicothoracic and thoracolumbar junction regions are prone to deformity and deformity progression if implants are placed to, but not beyond, these regions (Fig. 27.14a, b). Despite the fact that these regions usually encompass a neutral vertebra in the sagittal plane, the disc spaces adjacent to these neutral vertebrae are not parallel to the floor in the standing position. This angulation causes translation and angular stresses (component vectors) to be applied. This is particularly true in the cervicothoracic region, in which the angles are greater and the vertebrae smaller. Longer constructs may therefore be required. Hence, in addition to the caution applied to terminating long constructs in these regions, stabilization and fusion procedures should be considered as an adjunct to laminectomy in the cervicothoracic region. Of note, the cervicothoracic junction is a true junction. It is associated with an abrupt change in morphology—with the addition of ribs at T1 being of particular note. The thoracolumbar region is more of a “zone” within which there exists a very gradual loss of rib stabilization (via floating and then no ribs) and a gradual change in vertebral size. Therefore, use of the thoracolumbar junction as a “site for implant termination” is rather nebulous and precarious. Much, however, is yet to be learned regarding the nuances of instrumentation termination in the thoracolumbar junction (zone).

27.3 Spinal Pelvic Parameters
A consideration of the sacropelvis in any discussion of spinal deformity is imperative. The anatomical and pathologic nuances of this region are substantial and critical to an understanding of deformity and its appropriate correction. To begin simply, one may best consider the sacropelvis as a vertebra. Dubousset, in fact, considered it as such and termed it the pelvic vertebra. 2 The pelvic vertebra may be angled or tilted dorsally or ventrally. The angle of the pelvis, or rather the pelvic vertebra, affects the entirety of the spine. The tilt of the pelvis (or pelvic vertebra) affects the more rostral segments in a manner similar to a chain reaction, as do alterations of spinal alignment at any level (Fig. 27.15). In this regard, Smith et al observed an improvement of cervical alignment after the correction of global sagittal balance following pedicle subtraction osteotomy. 12 Others have demonstrated the importance of considering sagittal balance for cervical deformity and deformity correction. 13 In asymptomatic adults, the neutral upright sagittal balance is maintained, with a significant variation in the undulation of the lordotic and kyphotic regional curves. 14 The majority of asymptomatic adults are able to maintain their normal sagittal alignment as they age. Loss of lumbar lordosis is the most common etiology for loss of such alignment. Surgeons should act accordingly when performing lumbar spine surgery. 15

Of final note here is that spinopelvic parameters vary among ethnic groups. 16 This should be taken into consideration in the clinical arena.
Glassman et al observed that as the plumb line shifts forward, disability increases and quality of life decreases. 17 This can be affected by the tilt of the pelvic vertebra and the effects of congenital and aging-related parameters. Blondel et al similarly emphasized the importance of complete sagittal plane deformity correction if the optimization of health-related quality of life is the goal. 18 Similarly, uncorrected sagittal plane imbalance predisposes to symptomatic instrumentation failure. 19 Hence, deformity entails disability and adversely affects quality of life, as well as the success of surgical procedures. Obviously, prior surgery can have a profound effect on sagittal alignment.
The sagittal alignment of the spine is critical regarding both the determination of surgical strategy and quality of life and disability. 20 , 21 It therefore becomes imperative to understand the etiologic factors and underlying parameters that affect the curvature of the spine. 22 – 27 In order to enhance understanding, each parameter is presented individually, and then the parameters are considered collectively.
27.3.1 Pelvic Incidence
Pelvic incidence is defined as the angle between a line joining the middle of the sacral end plate to the center of the femoral head (femoral head axis) and a line drawn perpendicular to the sacral end plate (Fig. 27.16). It is a unique parameter that is specific for each individual. It does not change with position or alterations of spatial orientation. The greater the pelvic incidence, the greater the pelvic tilt and the greater the lumbar lordosis, thoracic kyphosis, and cervical lordosis. The pelvic incidence has been shown to be greater (significant pelvic tilt) in patients with spondylolisthesis. 25 A small pelvic incidence is associated with minimal pelvic tilt and a relative loss of lumbar lordosis, thoracic kyphosis, and cervical lordosis. Pelvic incidence helps define an individual’s natural lumbar lordosis (see below).

27.3.2 Pelvic Tilt
Pelvic tilt is a measure of the degree of pelvic retroversion. It is the angle between a vertical reference line drawn from the center of the femoral head (femoral head axis) and a line drawn from the femoral head (femoral head axis) to the midpoint of the sacral end plate (Fig. 27.17). As opposed to pelvic incidence, pelvic tilt is affected by position. It is a compensatory parameter. Pelvic tilt normally ranges from 10 to 25 degrees. If these parameters are exceeded (e.g., a pelvic tilt greater than 25 degrees), the patient may be at the limit of his or her ability to sagittally compensate. 23 Hence, pelvic tilt correlates with energy expended to maintain an upright posture.

27.3.3 Sacral Slope
Sacral slope is defined as the angle between a line drawn along the sacral end plate and the horizontal (Fig. 27.18). Similar to pelvic tilt, sacral slope is affected by position. It is, likewise, a compensatory parameter. Normal sacral slope ranges from 30 to 50 degrees. If a steep sacral slope is present, anterior lumbar interbody fusion (ALIF) may be contraindicated, although not absolutely. 28 This is so because of the steep angle of the lumbosacral junction, which exposes an interbody device to extreme shear forces.

27.3.4 Spinal Pelvic Parameters Considered Collectively
The pelvic incidence is equal to the pelvic tilt plus the sacral slope (Pelvic Incidence == Pelvic Tilt ++ Sacral Slope). This simply represents a geometric calculation or phenomenon (Fig. 27.19). Clinical relevance, however, is achieved when the aforementioned parameters are considered in the context of an overall deformity. To assist with this consideration, a general rule of thumb is helpful:
A simple restoration of sagittal alignment alone may not always represent the optimal treatment strategy when reconstructive spine surgery is being considered. Osteotomies can be used to achieve this, but if the patient is maximally compensated, as assessed by the observation of a pelvic tilt greater than 25 degrees and a decreased sacral slope, the patient’s ability to extend after the procedure remains limited. An overcorrection may be appropriate in such a patient, thus leaving the patient with a reserve, if you will, for extending the spine. Schwab et al suggest that patients with large spinopelvic deformities should perhaps be overcorrected via more extensive osteotomies or additional corrective procedures beyond standard corrective osteotomies in order to avoid undercorrection. 29
Individuals with a large pelvic incidence tend to have a relatively horizontal sacrum (large sacral slope), with an accompanying significant lumbar lordosis, thoracic kyphosis, and cervical lordosis. The converse is also true (Fig. 27.20).


Pelvic incidence and pelvic tilt essentially “define” the ability of an individual to compensate for sagittal imbalance. An individual with relatively normal spinal curvatures (lumbar lordosis, thoracic kyphosis, and cervical lordosis) may be grossly out of balance if the pelvic tilt is small, resulting in a large sacral slope. Another individual with a large pelvic tilt (> 25 degrees) may have a normal sagittal alignment and balance but be at the edge of compensation. Pelvic tilt is, indeed, a compensatory parameter. Hence, if a superimposed loss of sagittal balance occurred in the latter scenario as a consequence of progressive degenerative changes and a deformity correction procedure were to be performed, overcorrection might be in order to provide a reserve via a decrease in pelvic tilt (at the expense of an increased sacral slope) to a more normal range (< 25 degrees; Fig. 27.21). The prediction of postoperative sagittal alignment preoperatively is key. 30 An understanding of the complex interplay between alignment and spinal pelvic parameters is critical. 2 , 22 , 23 , 25 Ames et al have provided a very informative in-depth review of the deformity correction decision-making process and the impact of spinopelvic alignment on this process. 31 Such variables may also affect the success of long fusions to L5 and help determine the indications for ending such fusions at L5 or extending them to the sacrum or the pelvis. 32

Postoperative spinal pelvic parameters such as, most notably, a large pelvic incidence and small lumbar lordotic angle are associated with a greater risk for adjacent segment stresses and degenerative changes. 33 – 39 Specifically regarding the lumbar lordotic angle, the maintenance of lumbar lordosis following fusion results in a diminished incidence of adjacent segment stresses and instability in comparison with a kyphotic spine. 34 , 35
27.4 Clinically Relevant Deformity Classification Schemes
It is important to consider the fundamental categories in which complex deformities fall. Category-specific treatment strategies (within reason) can then be devised.
Complex spine deformities can be divided into distinct categories. King and colleagues divided coronal plane deformities into five categories (Fig. 27.22). 1 , 40 These deformities are multiplanar, as is their degenerative counterpart (i.e., degenerative rotatory kyphoscoliosis). The management of these complex deformities differs, depending on the curve type and other patient-specific characteristics. In addition, treatment is often controversial.

Other methods have been used for the definition of complex spine deformities. Their utility is enhanced by a meticulous definition of the deformity, such as the scheme described by Lenke et al. 41 , 42 This scheme places strong emphasis on the position of the lumbar apical vertebra with respect to the CSL, both before and after surgery. 41 , 42 It has been validated clinically (Fig. 27.23). 43 Table 27.2, Table 27.3, Table 27.4, Table 27.5, and Table 27.6 portray objective guidelines for using this scheme in operative decision making.

Coronal (side bend) | Sagittal (upright) | Axial (side bend) | |
Proximal thoracic | ≥ 25 degrees | T2–T5 ≥ +20 degrees | |
Main thoracic | ≥ 25 degrees | T10–L2 ≥ +20 degrees | |
Thoracolumbar–lumbar | ≥ 25 degrees | T10–L2 ≥ +20 degrees | < Nash-Moe |
No. | PT | MT | TL/L | Curve type |
1 | NS | S(M) | NS | Main thoracic |
2 | S | S(M) | NS | Double thoracic |
3 | NS | S(M) | S | Double major |
4 | S | S(M) | S | Triple major |
5 | NS | NS | S(M) | Thoracolumbar/lumbar |
6 | NS | S | S(M*) | Thoracolumbar/lumbar–main thoracic |
M, major (largest curve); M*, TL/L curve > MT by 10 degrees; MT, main thoracic; NS, surgical nonstructural; PT, proximal thoracic; S, surgical structural; TL/L, thoracolumbar/lumbar. Source: After Lenke et al. 41 , 42 |
PT |
|
MT |
|
TL/L |
|
MT, main thoracic; PT, proximal thoracic; TL/L, thoracolumbar/lumbar. |
Curve type | Structural regions | Regions by arthrodesis | Approach |
1. MT | MT | MT | PSF or ASF |
2. DT | PT, MT | PT, MT | PSF or ASF |
3. DM | MT, TL/L | MT–TL/L | PSF |
4. TM | PT, MT, TL/L | PT, MT, TL/L | PSF |
5. TL/L | TL/L | TL/L | ASF or PSF |
6. TL/L–MT | TL/L, MT | TL/L, MT | PSF |
ASF, anterior spinal fixation; DT, double thoracic; DM, double major; MT, main thoracic; NS, surgical nonstructural; PSF, posterior spinal fixation; PT , proximal thoracic; S, surgical structural; TL/L, thoracolumbar/lumbar; TM, thoracic major. |
Type | Description | Etiology | Problem located in the spine | Problem located beyond the spine |
Type I | Primary degenerative scoliosis (de novo scoliosis), mostly lumbar or thoracolumbar; curve apex at L2–3 or L4 most frequently | Asymmetric disc degeneration and facet joint degeneration | + | |
Type II | Progressive idiopathic scoliosis of the lumbar and/or thoracolumbar spine (e.g., double major curve) | Idiopathic scoliosis present since adolescence or childhood, progression due to mechanical reasons or bony and/or degenerative changes | + | ? |
Type III(a) | Secondary adult scoliosis, mostly thoracolumbar or lumbar/lumbosacral | Secondary to an adjacent thoracic or thoracolumbar curve of idiopathic, neuromuscular, or congenital origin Obliquity of the pelvis due to leg length discrepancy or hip pathology with secondary lumbar/thoracolumbar curve Lumbosacral transitional anomaly | + | + |
Type III(b) | Deformity progression mostly due to bone weakness (e.g., osteoporotic fracture with secondary deformity) | Metabolic bone disease, osteoporosis | + | + |
Source: From Aebi, 44 with permission of Springer-Verlag. |
Coronal curve types | Sagittal modifiers | |
T: Thoracic only with lumbar curve < 30 degrees | PI minus LL | 0: within 10 degrees +: moderate 10–20 degrees ++: marked > 20 degrees |
L: TL/L only with thoracic curve < 30 degrees | Global alignment | 0: SVA < 4 cm +: SVA 4–9.5 cm ++: SVA > 9.5 cm |
D: Double curve with T and TL/L curves > 30 degrees | PT | 0: PT < 20 degrees +: 20–30 degrees ++: PT > 30 degrees |
N: No major coronal deformity with all coronal curves < 30 degrees | ||
LL, lumbar lordosis; PI , pelvic incidence; PT, pelvic tilt; SVA, sagittal vertical axis; T, thoracic; TL/L, thoracolumbar/lumbar. Source: From Schwab et al, 45 with permission of Lippincott Williams & Wilkins. Curve type T: Patients with a thoracic major curve of greater than 30 degrees (apical level of T9 or higher) are classified with curve type T. Curve type L: Patients with a lumbar or thoracolumbar major curve of greater than 30 degrees (apical level of T10 or lower) are classified with curve type L. Curve type D: Patients with a double major curve, with each curve greater than 30 degrees, are classified with curve type D. Curve type N: Patients with no coronal curve greater than 30 degrees (i.e., no major coronal deformity) are classified with curve type N (normal). |
The aforementioned deformity classification schemes were designed for pediatric and adolescent scoliosis. They were useful predominantly for determining the levels of fusion and fusion termini. Adult and degenerative deformities are not always served well by these schemes. Three separate additional classification schemes have been devised to address adult deformities: the schemes of Aebi, 44 Schwab et al, 45 and the Scoliosis Research Society. The Aebi scheme divides deformities into four types, based on etiology (see Table 27.6). 44
Schwab et al introduced a scheme that derived classification criteria from a prospective study of a large patient population. 45 They categorized patients into five groups, based on the location of the apex of the major curve, and further subclassified them on the basis of two modifiers: degree of lumbar lordosis and subluxation. Finally, a third modifier, global balance, was added (Table 27.7). 46 – 49
Most deformities are associated with a primary and a secondary (compensatory) component. The secondary component is indeed compensatory if it occurs as a response to the primary curve. This is a manifestation of the attempt by the spine and the nervous system to “right” themselves in order to achieve balance. The aforementioned schemes are included herein for completeness, The surgeon should use these schemes to categorize patients in such a manner as to facilitate the determination of clinical strategy.
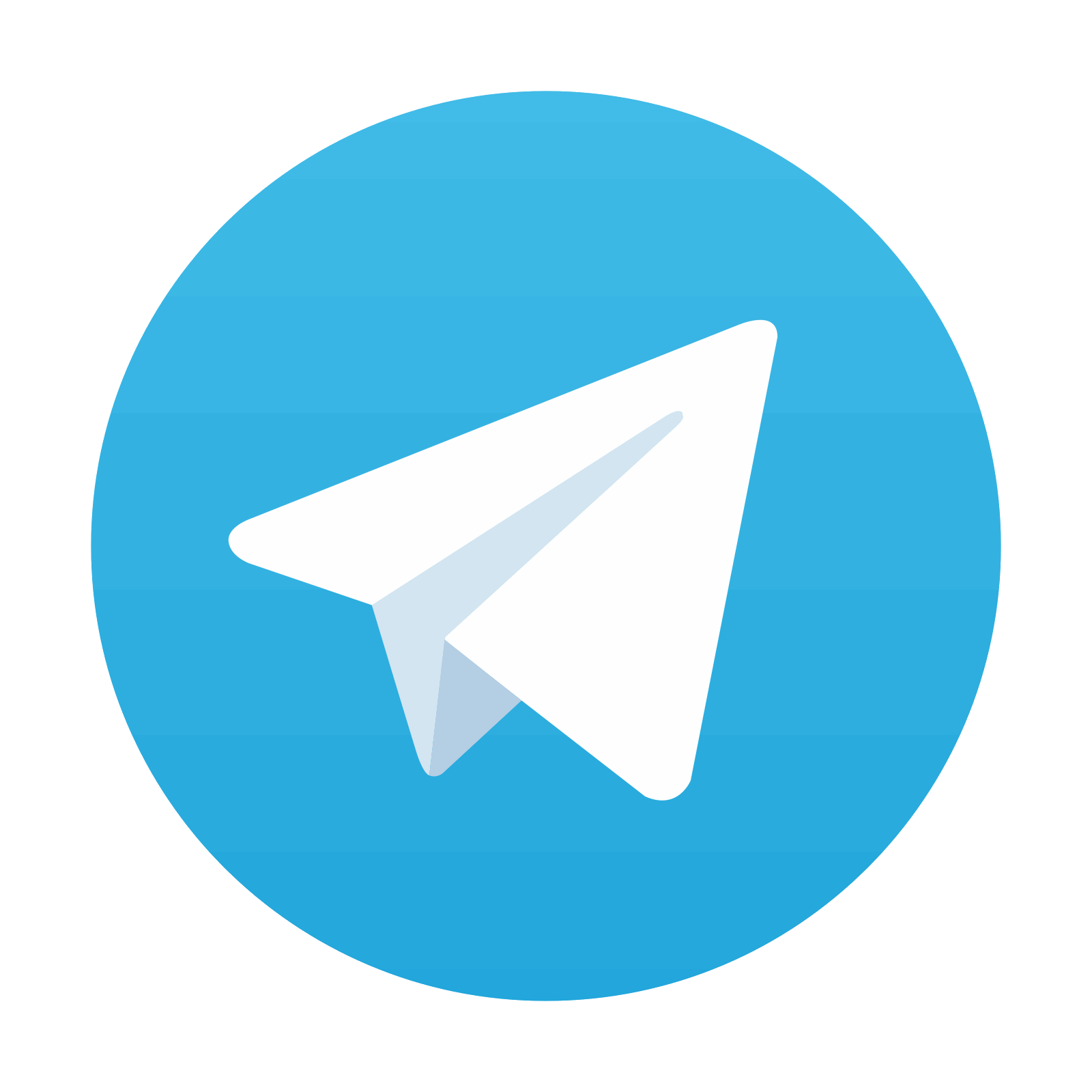
Stay updated, free articles. Join our Telegram channel

Full access? Get Clinical Tree
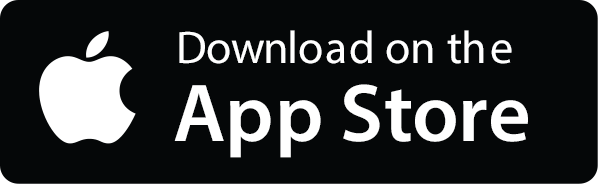
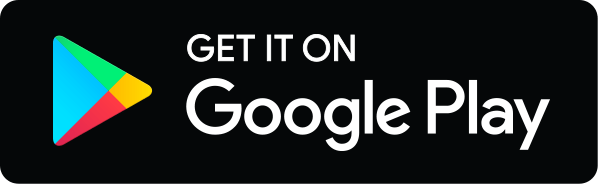