Summary
The clinical and surgical management of tumors that invade the orbit can be quite complex but is greatly facilitated by a comprehensive understanding of the orbital anatomy. The orbit can be separated into compartments and can be associated with specific clinical findings. The subsequent clinical work-up and diagnosis can be guided by the clinical findings. Pathologies within the orbit are typically described relative to the orbital cone and in relation to the rectus musculature. Accordingly, the surgical approach is selected that optimizes the opportunity for a complete surgical resection while respecting the orbital anatomy.
28 Tumors of the Orbit
28.1 Introduction
Clinical familiarity with the orbit is important for the comprehensive care of skull base lesions. Pathologies can originate within the orbit and extend into the skull base or, conversely, can invade the orbit from surrounding structures. The complexity of orbital anatomy requires a thorough understanding for determination of the optimal surgical approach for improving outcomes and minimizing complications. Depending on the location of the lesion, surgical approaches may also be performed in conjunction with other surgical services, including ophthalmology and otolaryngology. We present a review of the anatomy, differential diagnosis, and surgical approaches for orbital pathology.
28.2 Osteology
The orbit is a complex bony structure that houses the globe and ocular adnexal tissue (Fig. 28.1). The shape of the orbit can be described in a simplified manner as a pyramid with its apex pointing posteriorly.1 The four sides of the pyramid represent the roof, medial and lateral walls, and floor of the orbit, each having unique landmarks and clinical significance. The anterior face subsumes a roughly rectangular shape formed by the orbital rim (arcus marginalis), which is discontinuous medially to accommodate the lacrimal sac.1 , 2 The periosteum of the face turns roughly 90° to enter the orbit as periorbita and is tightly tethered to the orbital rim.

The orbital roof is formed largely by the frontal bone, with a small contribution made posteriorly by the sphenoid bone. The frontal bone thickens and protrudes anteriorly to form the orbital rim so as to accommodate the frontal sinus. At the superior orbital rim, the supraorbital notch and trochlear foramen allow for passage of the supraorbital and trochlear branches of the frontal division (V1) of the trigeminal nerve, providing sensory innervation to the upper face.3 Laterally, a concave fossa is found just posterior to the orbital rim that accommodates the lacrimal gland. Medially, the fibrocartilaginous trochlea is found 3 to 5 mm posterior to the orbital rim and acts as a pulley for the superior oblique muscle (SOM); careful disinsertion of the trochlea at its osseous insertion (rather than the SOM from the trochlea) is important to prevent injury to the muscle and postoperative diplopia.
The lateral orbital wall is formed by the zygomatic bone anteriorly and the greater wing of the sphenoid posteriorly. Two foramina are also found within the zygomatic bone, the zygomaticofacial and zygomaticotemporal, through which the respective nerves, arteries, and veins pass to provide sensory innervation and vascular supply to the midface. On axial radiographic images, the lateral wall subsumes an appearance of two triangles with their apices meeting at the sphenozygomatic suture; this anatomical landmark is important surgically, as it provides an area of weakness that can be exploited when fashioning a bone flap during lateral orbitotomy. An arterial anastomosis between the middle meningeal and ophthalmic artery is present in about 40% of patients along the frontosphenoidal suture line (foramen meningo-orbitale); control of this artery is important during orbitotomy to prevent vessel retraction and intracranial bleeding.1
The medial wall of the orbit is formed by multiple contributing bones. Most anteriorly, the frontal process of the maxilla forms the orbital rim and the anterior lacrimal crest. The maxilla also contributes to the inferomedial portion of the medial wall, extending toward the orbital apex as a thickening often referred to as the “maxillary strut.” The lacrimal bone is found just posterior to the frontal process, forming the bulk of the lacrimal sac fossa and the posterior lacrimal crest. The lacrimal sac sits within the fossa, whereas the anterior and especially the posterior lacrimal crests provide anchorage points for the medial canthal tendon. The majority of the medial orbital wall is made up of the ethmoid bone, abutting the ethmoid and sphenoid sinuses. This portion of the medial wall is the thinnest of the orbit (lamina papyracea) but might not be the weakest because of the trestlelike support of the underlying ethmoidal air cell septations.4
The anterior and posterior ethmoidal arteries pass through the anterior and posterior canals, respectively. The anterior ethmoidal artery is usually located at the level of the frontoethmoidal suture and the posterior ethmoidal artery at the sphenoethmoidal recess, and in many patients the canals define the level of the skull base (fovea ethmoidalis and cribriform plate). Not only do the locations of ethmoidal canals delimit endonasal landmarks, but they can also be used to define regions of the orbit.5 The bulbar part of the orbit is ventral to the anterior ethmoidals, followed by the retrobulbar portion between the anterior and posterior ethmoidal arteries, and the orbital apex is posterior to the posterior ethmoidal arteries. The medial orbital wall ends at the optic canal, comprising the body and lesser wing of the sphenoid bone, and is typically found 42 mm (range 32–53 mm) posterior to the anterior lacrimal crest.6 As a general rule we use the pneumonic “24, 12, 6”: the anterior ethmoidal artery is 24 mm posterior to the orbital rim, the posterior ethmoidal artery 12 mm behind the anterior ethmoidal, and the optic canal 6 mm posterior to the posterior ethmoidal.
Finally, the majority of the orbital floor is composed of the maxillary bone medially and the zygoma laterally, with a small contribution posteriorly by the orbital process of the palatine bone. The orbital floor is sloped upward by about 20° from anterior to posterior, an important consideration during fracture repair.1 The inferior orbital fissure (IOF) travels anteriorly at the junction of the inferior and lateral orbital walls and carries the zygomatic branch of V2, the infraorbital branch of V2, the infraorbital artery (a branch of the internal maxillary artery) and vein, and the parasympathetic postganglionic branches from the pterygopalatine ganglion that travel to the lacrimal gland. The IOF ends approximately 24 mm (range 17–29 mm) posterior to the orbital rim and communicates posteriorly with the foramen rotundum.1 The IOF is an important surgical landmark for many endoscopic procedures. In addition, because the IOF neighbors the superior orbital fissure and pterygopalatine fossa, spread of pathology can readily occur in these regions.7 The orbital floor is the most deformable wall when exposed to static loading, which explains the high frequency of fractures.4 When attempting to access the orbital apex surgically, it is important to remember that the floor does not extend to the apex but rather ends at the pterygopalatine fossa.
On imaging, axial view of the midorbit will reveal relatively straightforward normal relationships between the orbital walls; any variation should alert the clinician of possible pathology. The medial orbital walls maintain a roughly parallel configuration. The lateral and medial orbital walls meet at a 45° angle, whereas the lateral orbital walls are roughly 90° apart.1
The superior orbital fissure (SOF) lies at the apex of the orbit and is formed by the lesser and greater wings of the sphenoid, separating the orbital roof from the lateral orbital wall and forming a rough V-shape as it joins the more anteriorly oriented IOF. It provides an opening for many important anatomical structures to pass from the cranium into the orbit. It can be divided into two regions by a ring of fibrous tissue, known as the annulus of Zinn, which envelops structures within the superior orbital fissure as well as the optic nerve and ophthalmic artery, which traverse the optic canal. The four rectus muscles arise from the annulus of Zinn and travel anteriorly to form the intraconal and extraconal spaces of the orbit. The nasociliary, oculomotor, and abducens nerves pass within the annulus of Zinn. The frontal, lacrimal, and trochlear nerves as well as the superior and inferior ophthalmic veins pass outside the annulus of Zinn in the superior orbital fissure.
The optic canal exits the orbit superomedially along the apex of the orbital roof, traveling medially and superiorly at about a 35° angle toward the optic chiasm. The canal is 8 to 12 mm long and abuts the posterior ethmoid and/or sphenoid sinuses, depending on individual anatomy. Notably, in about 40% of individuals, the optic canal may bulge into the adjacent sinus, occasionally surrounded by a pneumatized sinus.8 , 9 A detailed review of all aspects of orbital anatomy can be found in Dutton’s highly regarded atlas on the subject.1
28.3 Regional Pathology and Differential Diagnosis
The differential diagnosis of orbital pathology is broad but can be concisely established based on the location of the pathology within the orbit and structures involved.10 It is important to keep in mind the variability of clinical manifestations depending on the location of the lesion. Rhoton et al described the division of the regional pathologies into three anteroposterior compartments delimited by the anterior and posterior ethmoidal arteries.5 A fourth region where pathology can lie is posterior to the orbit in the region of the optic nerves.
The most anterior compartment is the bulbar region, which contains the globe. Pathologies in this region can originate in the retina, choroid, sclera, and vitreous body but most frequently arise from the lacrimal gland through posterior spread of eyelid processes. The most common pediatric and adult intraocular malignancies are retinoblastoma and choroidal melanoma, respectively. A variant of idiopathic orbital inflammatory syndrome (IOIS) known as posterior scleritis affects the bulbar region and frequently spills posteriorly into the intraconal orbital fat. Various forms of inflammation (e.g., sarcoidosis, granulomatosis with polyangiitis, IgG4-related orbitopathy) may affect the peribulbar tissue, also extending posteriorly into orbital fat.
The middle compartment, or retrobulbar region, can be further divided into the intraconal and extraconal space. The cone is defined by the rectus muscles. Common intraconal pathologies (Fig. 28.2a,b) include cavernous hemangioma, IOIS, schwannomas, non-Hodgkin’s lymphomas (NHL), solitary fibrous tumors, and metastases (usually breast, prostate, or lung carcinoma), among others. The conal space (essentially the rectus muscles) is often affected by thyroid eye disease, IOIS, and NHL. Extraconal lesions are located external to the rectus muscles and can be further distinguished by their location relative to the periorbita. Lesions deep to the periorbita are considered “intradural” (Fig. 28.2c), and lesions outside the periorbita are extradural (Fig. 28.2d). Common extraconal lesions are dermoid cysts and benign or malignant lacrimal gland masses (e.g., pleomorphic adenoma, adenoid cystic carcinoma, NHL) that mimic salivary gland pathology. Common extradural lesions include mucoceles and subperiosteal abscesses from adjacent paranasal sinuses, as well as bony lesions such as osteomas, fibrous dysplasia, and hyperostotic sphenoid wing meningiomas.
The posterior compartment is the orbital apex. Pathologies affecting the orbital apex include parasellar or sphenoid wing meningiomas, schwannomas, and cavernous hemangiomas.
The optic nerve and its surrounding meningeal sheath may harbor a variety of neoplastic and inflammatory processes, including optic nerve glioma in children and optic nerve sheath meningioma in adults, as well as sarcoidal or lymphomatous infiltration, infection (Lyme’s disease), and demyelinating disease (optic neuritis with or without multiple sclerosis).
Other pathologies can originate from adjacent structures and grow to invade the orbit. Sinonasal malignancies are the most common lesions to secondarily involve the orbit. Tumors originating from the adjacent sphenoid wing include spheno-orbital meningiomas, metastases (especially from the prostate), lymphoproliferative disease (plasmacytoma, multiple myeloma), and histiocytosis X (especially eosinophilic granuloma in adults). The skin may also serve as the origin of subsequent orbital pathology; perineural spread of cutaneous squamous cell carcinoma into the orbit and skull base is an often overlooked condition and a leading cause of orbital exenteration.11 , 12

28.4 Clinical Assessment
Orbital pathology can present with a host of symptoms that vary in severity and duration. In general, pain is associated with infection, inflammation, or malignancy (especially with perineural invasion). Globe dystopia, either axially (exophthalmos) or nonaxially (e.g., hypoglobus, hyperglobus), may be noted. Diplopia may occur but is often absent, especially in slowly progressive, noninflamed, well-tolerated processes such as cavernous hemangioma, sphenoid wing meningioma, and NHL. Likewise, visual loss may be profound secondary to rapidly expansile or compressive lesions (orbital hemorrhage, subperiosteal abscess) or subtle in cases of slowly progressive processes (thyroid eye disease, cavernous hemangioma, sphenoid wing meningioma).
Clinical assessment begins with evaluation of the afferent system for signs of optic nerve dysfunction.13 Visual acuity with the Snellen chart and pupillary reaction to light are two methods of determining function of the afferent system. Confrontation fields can also be performed monocularly in all four peripheral and central quadrants with finger counting. Note that not all afferent visual parameters need be abnormal. As an example, a patient may have 20/20 central acuity and still harbor a significant optic neuropathy.
The efferent system should also be evaluated. A cardinal rule to remember when evaluating the efferent system is that pupils, extraocular motility, and eyelid position are related: when one is abnormal, it is extremely important to carefully check the other two. Anisocoria may be either physiologic or nonphysiologic. Physiologic anisocoria can be seen in up to 20% of normal individuals but is typically mild and seen in dim light.13 Nonphysiologic anisocoria may result from abnormalities of the sympathetic and parasympathetic innervation to the iris musculature but may also occur following intraocular surgery (e.g., cataract extraction).
Extraocular motility testing should be performed to look for full and smooth pursuits (slow, tracking movements) and saccades (rapid movements toward an eccentric target) in the four principle gazes, as well as oblique gazes.14 If either eye has a limitation of gaze, then forced duction and force generation testing with forceps should be performed to evaluate for a restrictive or paretic process, respectively. If the patient complains of diplopia, it is of paramount importance to ascertain whether the diplopia is monocular or binocular.13 Monocular diplopia is rarely caused by neurologic disease and typically connotes an abnormality of the eye (corneal or lens aberration, macular abnormality, etc.), whereas binocular diplopia points to an ocular misalignment.
The third parameter for evaluation of the efferent system is eyelid position. Palpebral apertures size should be measured as well for any signs of ptosis or eyelid retraction. Of note, in addition to the upper eyelid ptosis, patients who have Horner’s syndrome may also have asymmetry of lower eyelid position, with the affected lower eyelid positioned higher than the unaffected side in what is called “reverse ptosis.”
Slit lamp examination may reveal signs of conjunctival chemosis or hyperemia, indicating a possible orbital process. Arterialization of the conjunctival veins suggests an arteriovenous malformation of the orbit or skull base; this finding is most frequently encountered in a carotid–cavernous fistula.
Dilated funduscopic examination should be performed to evaluate the optic nerve, retina, and retinal vascular tree. Unilateral optic disc swelling suggests a localized orbital or optic nerve process, whereas bilateral disc swelling often indicates increased intracranial pressure and requires urgent neuroimaging.13 , 14 Optic disc pallor may be present in a variety of pathologies but usually indicates that the process is at least 4 weeks old. Chorioretinal folds are highly suggestive of an orbital (usually intraconal) process.
One additional useful technology for orbital and skull base disease is optical coherence tomography (OCT), which provides detailed information on the topography of the optic nerve and macula, supplying the clinician with objective measurements of a variety of parameters, including nerve fiber layer thickness. In addition, preoperative OCT has been shown to have prognostic implication in patients who have preoperative visual loss and who are undergoing transnasal hypophysectomy.15 , 16
In general, lesions closer to the orbital apex wreak greater havoc on the afferent and efferent visual system than those found in the anterior orbit. Optic neuropathy and concomitant multiple sensory and motor cranial neuropathies are seen frequently with lesions of the orbital apex, cavernous sinus, and adjacent areas of the skull base.
28.5 Diagnostic Imaging
The two imaging modalities that are critical for evaluation of orbital pathology are CT and MRI studies of the orbits. Sequences used for routine imaging of the brain do not provide the same detail as dedicated orbital studies.17 Multidetector CT is a rapid, readily available study that is highly useful in emergency or posttraumatic situations and when bony detail is necessary. CT of the orbit has distinct advantages over that of the brain, mainly because the fat in the orbit is relatively radiolucent on CT, providing excellent detail of nonlipomatous normal anatomy and pathologic processes. However, this detail diminishes significantly at the orbital apex and skull base.14 When possible, intravenous contrast should be used, although this is unnecessary in cases of orbital trauma or in thyroid eye disease.
Contrast-enhanced MRI with fat saturation (fat suppression) is critical in providing the soft tissue definition necessary to delineate the location and extent of an orbital lesion, especially at the orbital apex and skull base.14 , 17 Lack of fat saturation and contrast greatly diminish the efficacy of orbital MRI, mainly because in contradistinction to CT, the hyperintensity of the fat on T1-weighted images overwhelms normal anatomy and pathologic processes.
CT and MR angiography play an important role in certain orbital pathologies when there is a suspicion of abnormal vasculature. The three most common clinical situations include Horner’s syndrome (possible internal carotid artery dissection), oculomotor nerve palsy (posterior communicating artery aneurysm), and suspected carotid cavernous fistula (admittedly, CT angiography and MR angiography may not visualize small caliber vascular abnormalities). Color Doppler ultrasonography is useful in a variety of suspected embolic or vascular processes of the orbit, including evaluation for a carotid–cavernous fistula. Finally, four-vessel cerebral angiography remains the gold standard in the work-up of neuro-orbital vascular disease. Although the cerebral angiogram may be more invasive, it also allows the possibility of concurrent endovascular intervention.
28.6 Preoperative Preparation
The goal of surgery should be clearly delineated, especially when a pathology involves the orbital apex or skull base. Because of the density and complexity of important efferent and afferent neural structures in this area, significant potential risks exist, and these should be reviewed with the patient in detail. Any patient undergoing orbitotomy must be warned about the possibility of partial or complete iatrogenic visual loss, most of which has no effective treatment. Visual loss in orbital surgery is unpredictable and may occur secondary to intraoperative manipulation or compression of the globe and/or optic nerve, vasospasm of the ophthalmic and/or central retinal arteries, or laceration of the globe and/or optic nerve. The risk of visual loss is higher with surgery in the orbital apex.18 , 19 External ophthalmoplegia with symptomatic diplopia and ptosis is a common sequela of orbital surgery and is almost guaranteed following orbital apical surgery. Much or all of the external ophthalmoplegia will resolve spontaneously over weeks to months, but the patient is often quite symptomatic in the interim. Pupillary abnormalities are less common and less symptomatic, but the patient should be warned about this possibility.
28.7 Surgical Technique
The location of the lesion will dictate the surgical approach. Additional considerations are the surgical goals with biopsy, decompression, or resection. The surgical approaches to the orbit can be broadly divided into two categories: transcranial extraorbital and transorbital extracranial. In general, the transcranial route is indicated for posteriorly placed lesions in the orbit (orbital apex and optic canal) or if there is infiltration into the skull base. Tumors in the anterior orbit that are superficial can often be reached extracranially. Each of the approaches is described in more detail hereafter.
28.7.1 Extraorbital Transcranial Approaches
The transcranial surgical approaches to the orbit may be arbitrarily divided into two types based on whether the superior orbital rim is removed when exposing the orbital lesion. Early approaches involved removal of a frontal or frontotemporal bone flap, with preservation of the supraorbital rim, and opening of the orbit behind the rim.10 , 16 , 17 , 18 , 19 , 20 , 21 , s. Literatur The transcranial approaches to the orbit use exposures of the orbital roof and lateral wall that are very common to neurosurgeons. We offer a brief description of these approaches within the context of treating lesions of the orbit.
A standard frontotemporal craniotomy can provide optimal access to the optic canal and orbital apex through the orbital roof, as well as the SOF and IOF and the cavernous sinus. It is important to drill and flatten the sphenoid wing so as to facilitate access to the anterior cranial fossa floor, which corresponds to the superior border of the orbit. The meningo-orbital band is incised to relax the frontal and temporal dura and to minimize retraction. The floor of the anterior fossa can then be drilled with a diamond bit. Care must be taken to maintain the periorbital plane. The periorbita is then opened in a semilunar fashion. At this point, three routes can be taken to access the lesion relative to the optic nerve. The medial route is between the SOM and the superior rectus/levator complex. The central route requires splitting of the superior rectus/levator complex. The lateral route is directed between the superior rectus/levator complex and the lateral rectus muscle. Blunt dissection with cotton-tipped applicators and neurosurgical cottonoids is used to access the specific route. The motor nerves (III, IV, and VI) are often encountered via the superior approach and should be gently reflected to minimize iatrogenic injury. The oculomotor nerve branches are typically robust and easily identified and lie within the intraconal space. In contrast, the trochlear nerve travels from the superior orbital fissure in the extraconal space and is the smallest cranial nerve; it is frequently injured during orbital dissection, especially with the superomedial approach.
The borders of the lesion are defined circumferentially, and the tumor is either excised en bloc or decompressed internally. If possible, the tumor is carefully dissected off the surrounding structures and removed. The extraocular muscles are often bluntly retracted during tumor dissection, which must be done with care in the orbital apex, because the motor nerves innervate each rectus muscle on its internal aspect at the junction of the posterior third and anterior two-thirds of the muscle. The orbital roof is reconstructed with a collagen sponge and a malleable titanium plate, taking care to avoid iatrogenic compression of the orbital apex and trapping of the muscles. The supraorbital osteotomy via an eyelid or eyebrow incision offers a less invasive approach to access the floor of the anterior cranial fossa, and specifically the retrobulbar area and orbital apex. The contralateral optic nerve and canal are more difficult to visualize. The size of the frontal sinus must also be considered, as a large and more lateral sinus extension may be better suited for a frontotemporal craniotomy to facilitate access for frontal sinus reconstruction or cranialization. An incision along the inferior or superior border of the eyebrow may also be used and is extended medially up to the supraorbital notch.
The periorbita is cut with a 15-blade or Bovie cautery along the orbital roof, and this dissection proceeds posteriorly and subperiosteally along the orbital roof, laterally to the zygoma, medially to the supraorbital notch, and superiorly along the frontal bone. The temporalis is dissected to expose the keyhole. The soft tissue is retracted with fish hooks. For the eyelid and eyebrow approaches, a bur hole is placed at the keyhole to expose the frontal dura and periorbita. A frontal craniotomy with orbital osteotomy is performed from this bur hole, starting superiorly over the frontal bone medial to the supraorbital notch. Next the orbital roof is drilled from the bur hole while protecting the periorbita. Finally the zygoma is detached inferior to the frontozygomatic suture. The anterior fossa floor can then be thinned to permit access to the superior orbit. These approaches permit access to the superior and lateral orbit from the retrobulbar region to the orbital apex, optic canal, and superior orbital fissure. The periorbita is opened with a no. 12 Bard-Parker sickle blade. Blunt dissection allows for identification of the lateral rectus and superior rectus/levator complex. The tumor can then be resected using microsurgical techniques. The orbital wall is reconstructed with a malleable titanium plate. The frontal sinus can be packed with iodine-soaked Gelfoam if the opening is small; otherwise it will need to be cranialized and obliterated with pericranium. The craniotomy bone is then reapproximated with low-profile titanium plates at the bur hole, zygoma, and frontal areas. The galea and skin are closed with 2–0 Vicryl and 4–0 Monocryl subcuticular sutures, respectively.
A subfrontal craniotomy provides circumferential access to the orbit from medial to superior to lateral walls, from bilateral retrobulbar areas to orbital apex. Possible modifications include an extended subfrontal craniotomy, which includes a bilateral orbital osteotomy where the bilateral keyholes are connected to transect the superior orbital rims along with the roofs of the orbits.
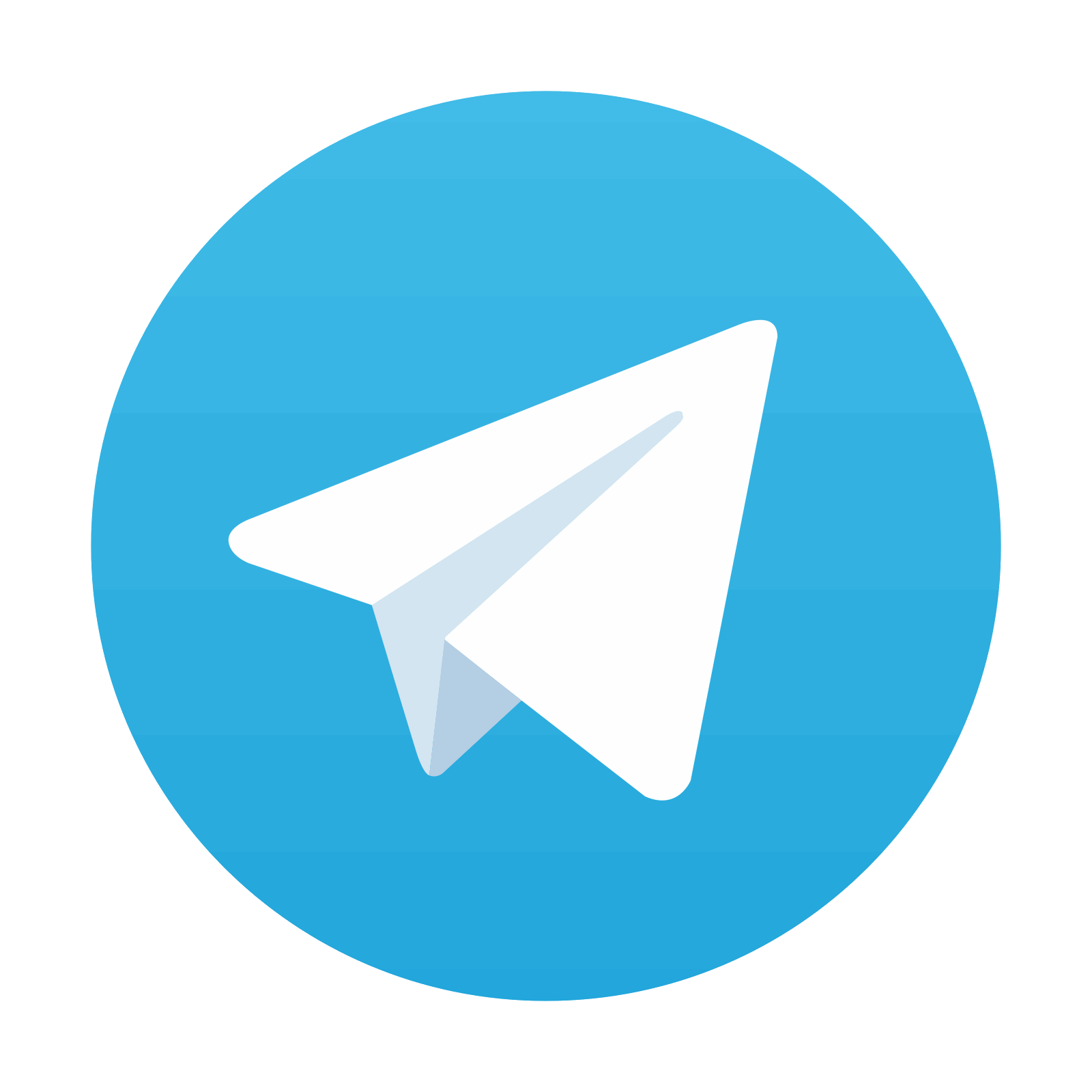
Stay updated, free articles. Join our Telegram channel

Full access? Get Clinical Tree
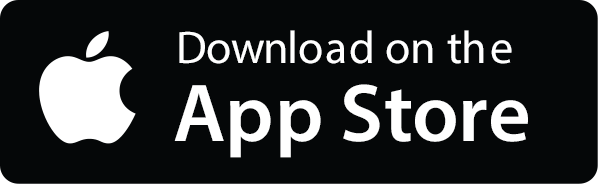
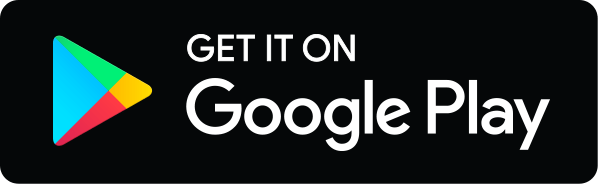
