29 Radiosurgery for Medically Refractory Epilepsy
Abstract
Although conventional open resection remains the mainstay for focus ablation in medically refractory cases, the search for less invasive, less costly, and safer procedures to treat focal epilepsy is justifiable. The obliteration of the seizure focus with noninvasive approaches has reached level of evidence in randomized trials with well-defined results and complication rates. The goal of radiosurgery is ablation of the focus and modulation of adjacent circuitry. DNA modification, provoking chromosomal instability which leads to cell modification or death by hindering correct mitosis, represents the radiobiological principle in radiosurgery. Besides cell death that may occur in the epileptic focus, therefore halting low-threshold neurons from firing, modification of cell function in the area may take place as well. This may occur by glial proliferation, microvascular environment modification, scar formation, and neurotransmitters neoformation and imbalances. Although treatments with classical parameters are hampered by the high incidence of complications linked to radiation-induced edema when treating hippocampal sclerosis, more restrictive indication criteria and development of new protocols based on other treatment paradigms may keep efficacy and reduce morbidity for the technique. More encouraging results were found in the treatment of other causes of epilepsy. The resolution of seizures caused by the presence of arteriovenous malformations after radiosurgery brought the enthusiasm of treating the focus of the seizure with the technique. The same can be said about seizures in patients with cerebral cavernous malformation. Many studies using Gamma Knife as an option to treat hypothalamic hamartoma were published over the last years Radiosurgery has also been used in different types of focal epilepsy as a complement for surgery or when the patient refuses resection.
29.1 Introduction
The burgeoning development of anatomical and functional neuroimaging settled in the dawn of the 21st century changed the landscape of epilepsy surgery. It led to the possibility of noninvasive focus definition and the development of a number of minimally invasive procedures. 1 , 2 , 3 Exquisite stereotactic surgery hinged on these images, 4 , 5 coupled with techniques of navigation and visualization of target during surgery, brought the enthusiasm for seizure focus ablation with laser and radiofrequency. These novel approaches are in phase of development. Even more enticing is the obliteration of the seizure focus with noninvasive approaches, such as high-frequency focus ultrasound and radiosurgery. 2 , 6 The latter, already tried since the 1980s, 7 has reached level of evidence in randomized trials with well-defined results and complication rates. 1 Herein, we discuss the applications of radiosurgery for treatment of focal epilepsy, with emphasis in comparison with conventional surgical techniques. This approach, initiated in the 1980s by Barcia-Salorio and collaborators, has evolved slowly with the improvement of the radiosurgery techniques, 7 , 8 , 9 the definition of the epileptic focus with functional imaging, and safer diagnostic invasive approaches. Stereoelectroencephalography (sEEG) has further confirmed the imaging findings, bringing confidence to the use of noninvasive ablation of the seizure focus. Presently, radiosurgery is poised to progressively complement and compete with the traditional resection techniques.
29.2 Developments Permitting Noninvasive Techniques
Facilitation of diagnosis, decreased demand for invasion, and the scope of surgical procedures make the benefits of surgery available to the needy patient population remaining aside of the normal society flow by their frequent epileptic fits. This is the goal of specialized teams treating severe epileptic patients.
Well-formed multidisciplinary teams of doctors, psychologists, physical and occupational therapists, and truly qualified epilepsy experts are scarce. There are few centers in the world dedicated to these patients due to costs and complexity of their management, diagnosis, and surgical procedures demanded. Several multicenter controlled trials determined that when the focus is well identified, 10 complete control of the epileptic seizures occurred in 30 to 90% of the cases, 11 and in 65% of the patients in more rigorous randomized clinical trials. 12
Modern surgical series demonstrated that complications related to the investigation and surgery necessary for the treatment of refractory patients are not negligible. 11 , 13 These include stroke, postsurgical bleeding, blood loss, and infection. They also limit the wider use of open surgery. 14 , 15 Additionally, important limitations for resective surgery include potential neurocognitive impairment when the focus is in the language-dominant hemisphere; acute social adjustment reactions during the recovery period, and societal adjustment in the long-term follow-up. 16 , 17 , 18 , 19 Although conventional open resection remains the mainstay for ablation of the seizure focus in medically refractory cases, as recommend by the American Academy of Neurology, 20 the search for less invasive, less costly, and safer procedures to treat focal epilepsy is justifiable.
When the seizure focus, i.e., the precise neuronal group firing to initiate the spread of ominous electric current throughout the brain, is identified and medical therapy fails to control repetitive seizures, thus hampering the quality of life of the adult or the normal development of a child, surgery becomes the only option. It aims to remove the focus or halt the seizure spread by blocking its pathways of dissemination. 21 Precise definition of the focus is not a simple task, frequently requiring invasive procedures. Even the safe stereotactic techniques used in sEEG carry morbidity and mortality. 22 Radiosurgery became an important tool to manage refractory surgery due to these limitations of the conventional surgical approaches.
29.3 Basic Principles of Radiobiology
The goal of radiosurgery is ablation of the focus and modulation of adjacent circuitry. Different types of lesions generating seizures can be treated with radiosurgery, such as tumor, vascular malformation, hippocampal sclerosis (HS), hypothalamic hamartoma (HH), focal cortical dysplasia, and others. 23 , 24 , 25 , 26 DNA modification, provoking chromosomal instability which leads to cell modification or death by hindering correct mitosis, represents the radiobiological principle in radiosurgery. Effectiveness is related to tissue’s ability of repairing damage or replication of errors culminating in cell function modification or death. 27 , 28 Response timing can be divided in two groups: early and later responders. Precocious responses are seen in tissues with higher cellular turnover, such as skin and mucous membranes, which show response in a few days to weeks, due to rapid accumulation of mutations. Late responders show efficacious responses months to years after treatment, due to the lower rate of cell turnover. Brain tissue is one of the most important examples of late responders. 29 Therefore, one would expect that the response after treatment with radiosurgery of patients with epilepsy would be delayed.
Another aspect associated with the response pattern in patients treated with radiosurgery is the type of functional arrangement. Tissues with cells arranged in sequence (i.e., unit to unit), such as the spinal cord, are affected with lower radiation doses due to the fact that a one-unit destruction leads to circuitry interruption. Tissues with a parallel organization, like the brain, need more radiation so that many cells would be destroyed with the objective of stopping local and regional activity. 30 This bears an important role in the disconnective approach to halt seizures in the brain. The objective of radiosurgery is the destruction of one or multiples target cell populations with minimal effect over neighboring tissues. The most accepted model of tissue response to radiation is the linear quadratic model. 31 , 32 , 33 , 34
The use of high single dose radiation with high precision and accuracy can preserve the surrounding healthy tissue around the seizure focus, obviating the need for fractioning the dose. It is represented graphically as paused descending curves (each fraction looks like a “shoulder” in the graphic). Radiosurgery, contrary to conventional fractionated dosing, causes irreversible damage to the target (acute downward curve on the irradiated cells survival). 29 , 35 This is important when the tissue receiving radiosurgery should be ablated, as is the case of neoplasia. However, when the desired effect is only to block neuronal firing, as in the case of an epileptic focus, lower and safer single dose may suffice. We have shown that nonnecrotizing radiation doses can be used to increase the seizure threshold above 150%. 36 This is the case when radiosurgery is used to treat HH, as described below.
The classical effects of radiation obtained in patients with tumors may not apply to the treatment of epilepsy; there is a lack of response when conventional fractions of radiation are applied to the seizure focus. The efficacy of fractionation doses in the treatment of neoplasia is based on the 4R’s proposed by Steel in 1989 (repair, repopulation, reassortment, and reoxygenation). After at least 4 to 6 hours between doses, healthy cells irradiated with sublethal doses initiate mechanisms of adjustment of this damage, i.e., repair tending to replace the damaged cells in the area (repopulation). At the same time, fragile neoplastic cells become more radiosensitive to the effects of the next dose (reassortment) and free radicals (generated by changes in blood flow in the irradiated region—reoxygenation) proceed to damage the DNA of these cells and also contribute to radiosensitivity. 29 , 30 , 32 , 37 , 38 Although effective for treatment of a variety of tumors, this biology is likely not the one taking place when treating nondividing cells, as are the firing neurons populating the seizure focus.
29.4 Vascular Effects and Seizure Control
Vascular endothelium inflammation is also associated with the radiosurgery local effect. Vascular structures present in the irradiated tissue (▶Fig. 29.1) will show apoptosis in about 20 hours after irradiation and later will be replaced by fibro-blasts, leading to a microvascular disorder. This reaction leads to microvascular obliteration. This radiobiological characteristic is highly associated with the therapeutic effects in tumors and vascular malformations. 38 , 39 , 40 , 41 , 42 , 43 , 44

Indeed, it was the resolution of seizures caused by the presence of arteriovenous malformations (AVMs) after radiosurgery that brought about the enthusiasm of treating the seizure focus with the technique. Of the 59 AVMs patients admitted with seizures at the Karolinska Institute in Sweden, 11 (18.6%) became seizure-free and were receiving no anticonvulsant medication and 30 pa- tients (50.8%) became seizure-free under anticonvulsant medication, reaching a 69.4% remarkable seizure control rate. 45 This spearheaded the Karolinska group to study the effects of radiosurgery in the seizure focus. 46 , 47
29.5 Effects of Radiation in the Epileptic Focus
Besides cell death that may occur in the epileptic focus, therefore halting low-threshold neurons from firing, modification of cell function in the area may take place as well. This may occur by glial proliferation, microvascular environment modification, scar formation, and neurotransmitters neoformation and imbalances. 48 These modifications of local epileptic group of cells are well exemplified by the effects of radiosurgery in the epileptogenic tissue surrounding cavernous angioma. The risk of seizures in patients harboring cerebral cavernous malformations (CMs) is reported to range from zero to 14% in those not initially presenting with seizures. 49 , 50 , 51 Surgical resection is indicated in cases of drug-refractory seizures, and is the intervention of choice for resolution of seizures. 52
When resection is not possible, radiosurgery is an acceptable approach. Lévêque et al studied 49 patients with cortical or subcortical CM and severe long-term drug-resistant epilepsy. They treated these patients with a peripheral dose of 19.17 Gy delivered by a Gamma Knife (GK). Patients had a mean duration of epilepsy of 7.5 years. The mean frequency of seizures in the group was 6.9 per month. Seizure control was very encouraging, as 53% of the patients became seizure-free, while a significant decrease in the number of seizures was observed in 20% of the patients. This effect appeared to be due to neuromodulation of the epileptogenic iron-impregnated tissue surrounding the CM. 53
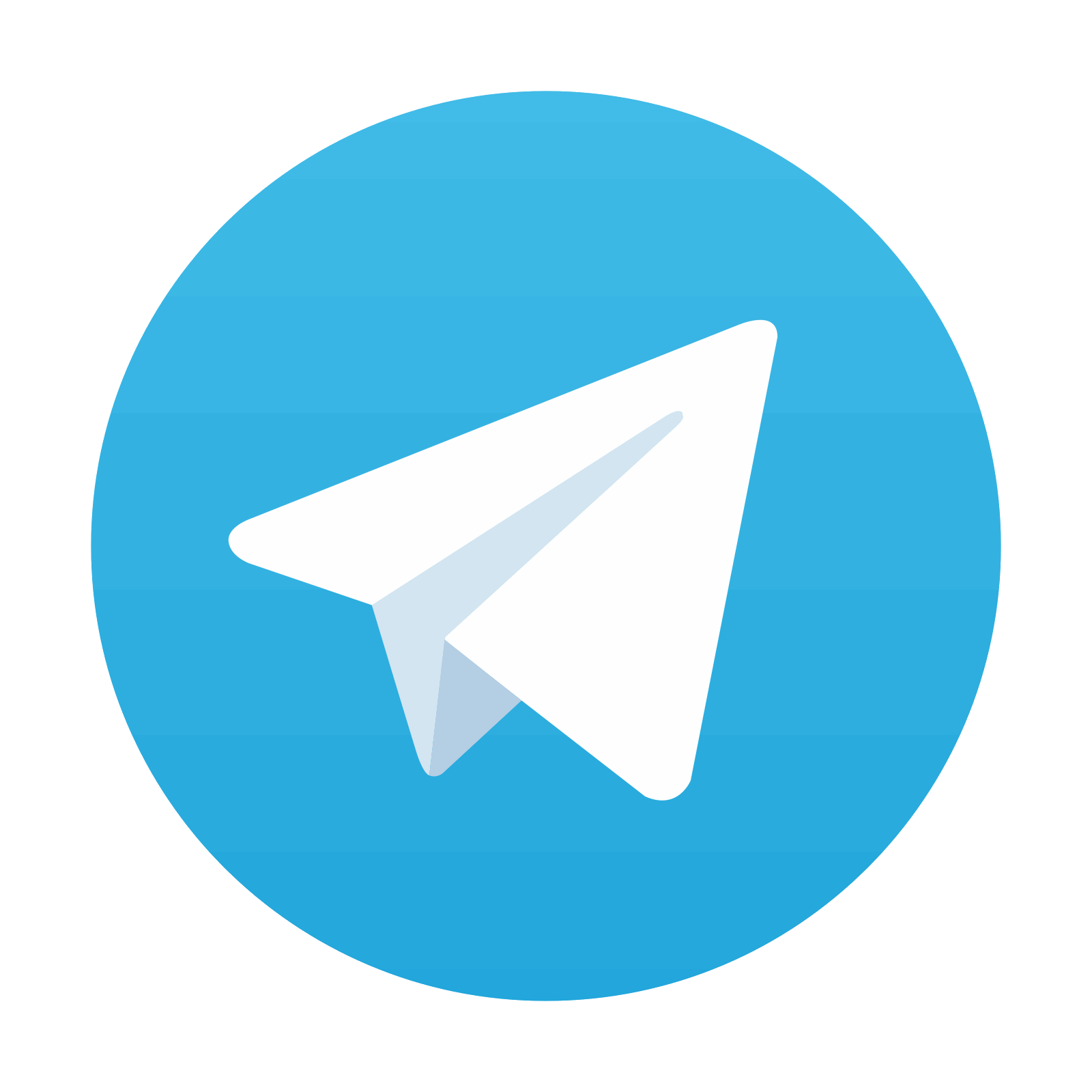
Stay updated, free articles. Join our Telegram channel

Full access? Get Clinical Tree
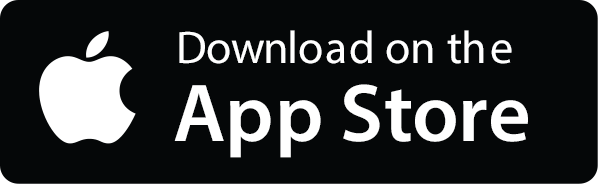
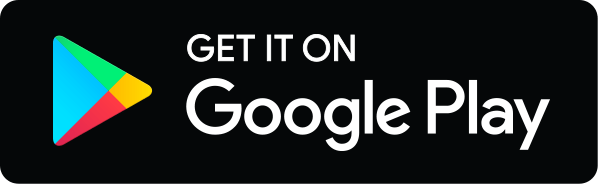