29 Stereotactic Radiosurgery for Treatment of Primary and Metastatic Spinal Tumors
Abstract
Spinal tumors are a frequent occurrence and the improvement in available cancer therapy is leading to longer survival. The biggest advancement in oncological treatment of spinal tumors has been the development and integration of spine stereotactic radiosurgery (SRS) which has dramatically improved the ability to provide safe and durable control of spine tumors. For treatment of metastatic spinal disease, an abundance of data show that radiosurgery provides high rates of local-tumor control with limited treatment-associated morbidity. Surgery continues to play an important role in the treatment of spinal metastases, but the integration of SRS has changed both the indications and surgical techniques. Radiosensitive tumors can be effectively treated with conventional external beam radiation therapy, while radioresistant tumors without significant epidural extension can be effectively treated with upfront SRS. In the setting of high-grade epidural spinal cord compression, dose constraints prohibit the delivery of an optimal radiation dose to the entire tumor volume without risking radiation-induced myelitis: thus, surgery is often required not only to decompress the spinal cord but also to provide a margin for the safe delivery of SRS. The role for SRS in the treatment of primary spinal tumors continues to evolve, but there is mounting clinical evidence that chordoma is radioresponsive to SRS. As radiosurgical technologies have become accessible worldwide, utilization of radiosurgery for the treatment of spinal pathology has grown. Spine surgeons must be familiar with radiosurgical concepts including simulation, contouring, and treatment planning to optimize decision making.
29.1 Introduction
Spinal tumors are classified as either primary or metastatic depending on the site of origin. Metastatic spinal cord tumors are far more frequent than primary tumors, as it is known that approximately 40% of all people with cancer develop spinal metastases and postmortem studies suggest that up to 90% of cancer patients may have microscopic evidence of spinal metastases. 1 , 2 Almost 20% of patients diagnosed with spinal metastases progress to symptomatic cord compression. 3 , 4 , 5
Treatment goals for patients with spine tumors include durable local tumor control, preservation or restoration of neurological function, maintenance of spinal stability, and palliation of pain. The goals of treatment among patients with spinal metastases are palliative. However, some patients with primary tumors, especially benign or low-grade malignant primary spine tumors, may be cured. Historically, treatment responses for osseous tumors to systemic therapy were limited and thus conventional external beam radiation therapy (cEBRT) was the mainstay of treatment for spinal tumors. 6 , 7 , 8 In current practice, the integration of medical and technological advancements has led to complex multimodality therapy strategy. The biggest advancement in the oncological treatment of spinal tumors has been the development and integration of spine stereotactic radiosurgery (SSRS) which has dramatically improved the ability to provide meaningful, durable control of spine tumors. This is particularly important and necessary in the current era of extended survivals seen with modern systemic therapy.
As radiosurgical technologies have become accessible worldwide, utilization of radiosurgery for the treatment of spinal pathology has rapidly growing. Spine surgeons must familiarize with radiosurgical concepts to optimize decision making. This chapter will discuss the basic concepts of radiosurgery for primary and metastatic tumors.
29.2 Terminology
Stereotactic body radiotherapy (SBRT) is an umbrella term describing single fraction or hypofractionated image-guided delivery of focused high-dose radiotherapy to targets throughout the body. The fractionation schedule generally varies between one and five fractions, with single-fraction SBRT occasionally being referred to as stereotactic radiosurgery (SRS; Table 29‑1). Once radiosurgery has been selected as the treatment of choice, whether in a primary setting or in the postoperative setting, the treatment process consists of several stages.
First, a simulation is performed. This is the process of obtaining up-to-date imaging, for accurate delineation of tumor and organs at risk (OARs), with patients placed in an immobilization frame that would provide reproducible positioning during therapy planning and delivery. Delivery precision is confirmed through reproducible immobilization, intraprocedural target location confirmation using on-board imaging, active motion detection, and corrections during treatment delivery. 9 , 10 , 11
Next, contouring of the treatment target and OARs is performed on a designated software platform. Optimal imaging translates into optimal contouring and hence often, particularly in the postoperative setting where hardware-related artifacts are common, a computed tomography (CT) myelography is performed during simulation in order to clearly delineate the position of the spinal cord. Alternatively, noncontrast CT can be performed and fused with a contrast-enhanced MRI. Three main volumes are contoured: the gross tumor volume (GTV), clinical target volume (CTV), and planning target volume (PTV). 12 The GTV represents the actual tumor seen on imaging. The CTV is the area of potential microscopic spread of tumor cells that includes the GTV and adjacent bone marrow spaces. The PTV is a geometric expansion around the CTV with a 2- to 3-mm margin to ensure that the CTV receives the planned dose. This margin accounts for factors that are challenging to control precisely such as physical errors of the treatment delivery, uncertainty of patient positioning and motion during treatment, and organ motion during treatment. Guidelines exist for contouring in both the primary 13 (Fig. 29‑1) and postoperative setting 14 , 15 (Fig. 29‑2) and spine surgeons should familiarize with them. In the postoperative setting, the full preoperative tumor volume is used for the GTV delineation, with respective expansions also made around the preoperative tumor.


Lastly, treatment planning is performed. The plan is generated based on the prescribed radiation dose and the tolerable dose to the surrounding OARs. Due to the complexity of decision making involved in these cases, it is preferable for treatment plans to be generated in a multidisciplinary fashion composed of radiation oncology, medical physics, and neurosurgery teams.
29.3 Radiobiology of Stereotactic Radiosurgery
Conventional external beam radiation therapy results in mitotic or cytotoxic cell death via breakage of double-stranded DNA within a tumor and may subsequently decompress the spinal cord. 8 , 16 , 17 Using cEBRT, radiation is typically delivered through one to four beams to a treatment field that spreads beyond the tumor margins. Hence, the dose of radiation is limited since vital OARs that surround the tumor receive doses of radiation similar to the doses prescribed to the tumor. 18 Healthy tissues are able to repair most of the damage from this radiation, while tumor cells generally have impaired DNA repair mechanisms and can succumb to these fractionated doses of radiation. Treatment response rates to cEBRT are variable, likely due to the limitations in radiation-dose delivery to the tumor. Historically, tumors have been classified as either radiosensitive or radioresistant based on the response to cEBRT. Lymphoma, myeloma, and seminoma are considered radiosensitive histologies, as are solid tumors such as breast and prostate. 19 , 20 Renal cell carcinoma (RCC), colon, non-small cell lung carcinomas (NSCLC), sarcomas, melanoma, thyroid, and hepatocellular carcinoma represent radioresistant tumors. 7 , 8 , 19 , 20
Patients with radiosensitive tumors can be treated effectively with cEBRT, irrespective of the degree of epidural spinal cord compression (ESCC). 6 , 8 Furthermore, patients with radiosensitive tumors are more likely to maintain ambulation and remain ambulatory longer than patients with unfavorable histologies after cEBRT. 8 , 20 , 21 , 22
The integration of SSRS has revolutionized the treatment of spinal metastases. Radiosurgery provides high-dose per fraction and uses intensity-modulated radiotherapy consisting of numerous beam angles (typically nine or more) to create conformal radiation contoured to optimize the dose delivered to the target while minimizing injury dose to OARs. Technological advancements in treatment planning and delivery including patient and tumor immobilization, image guidance, and intensity-modulated radiation delivery through multi-leaf collimators have made spinal SBRT safe and effective. 23 , 24 Ultimately, the ability to deliver high-dose per fraction of conformal radiation enables delivery of cytotoxic tumoral doses, which, in turn, overcomes the radioresistance of most tumors to cEBRT. 25 In experimental models, different radiobiologic responses have been shown between low-dose and high-dose per fraction radiation. 26 , 27 High-dose per fraction (i.e., >10 Gy per fraction radiation) kills tumor cells via breakage of double-stranded DNA but additionally elicits tumor control through effects on the tumor vasculature. 28 One theory is that SRS induces the sphingomyelinase pathway, which, in turn, results in microvascular endothelial dysfunction and apoptosis, subsequent hypoperfusion of tumor tissue and ultimately tumor cell destruction. 29 The vascular damage then causes ischemic or indirect/secondary tumor cell death within a few days after radiation exposure. Moreover, the extensive tumor cell death secondary to both the radiation and the vascular damage may lead to the release of tumor-associated antigens and various proinflammatory cytokines, thereby triggering an antitumor immune response. 30 , 31 Ultimately, by all these pathways, SRS offers improved tumor cell death with limited side effects.
SSRS yields a clinical benefit regardless of tumor histology, providing a durable symptomatic response and high local-control rates, but these responses appear to be dose dependent. 32 , 33 , 34 Recent evidence 35 shows excellent outcomes with SSRS for traditionally radioresistant histologies such as RCC, 36 , 37 , 38 sarcoma, 39 and melanoma. 40
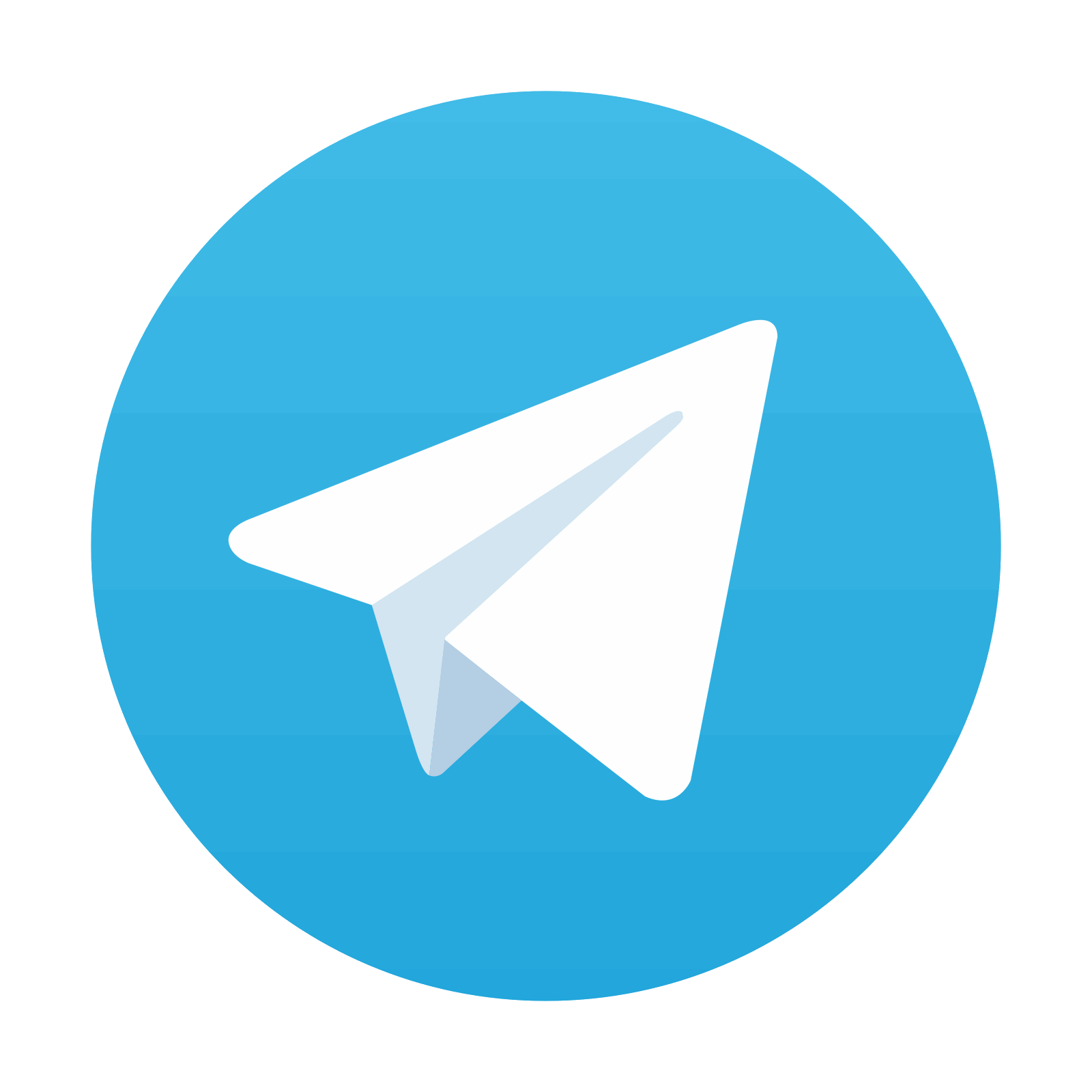
Stay updated, free articles. Join our Telegram channel

Full access? Get Clinical Tree
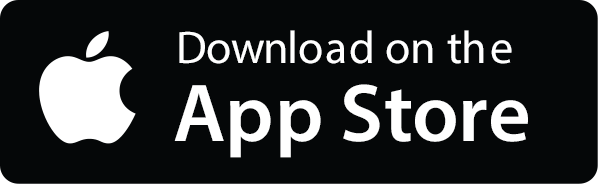
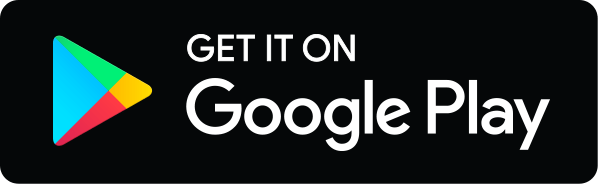