29 Subsidence and Dynamic Spine Stabilization
Following the attainment of adult stature and height, humans shorten (subside). This process is minimal (limited) through the third and fourth decades of life, but it accelerates continuously thereafter. The loss of vertical height associated with aging is multifactorial. It can in general be considered to be related to three phenomena: (1) disc interspace height loss, (2) vertebral body collapse, and (3) deformity progression. All involve axial deformation (deformation along the neutral axis) and/or angular deformation (deformation about an axis of rotation—for example, kyphosis). Another form of deformation, rotation about the long axis of the spine, is not well understood and does not warrant discussion here, although it is identifiable with imaging studies. 1
Angular deformation is associated with a loss of either or both ventral disc height and vertebral body height. This is typified by a process that results in progressive kyphosis (Fig. 29.1a). Of note, a “stable kyphosis” can be associated with axial neck pain. 2 This is reason enough to aggressively address kyphosis prevention at the time of surgery (see the following). Perhaps more importantly, a kyphosis creates a long moment arm, which in turn creates a tendency toward kyphosis progression. This is perhaps most appropriately portrayed by the phrase “deformity begets deformity” (Fig. 29.1b).

Two terms, settling and subsidence, are used interchangeably here and specifically with reference to the spine, as they pertain to axial deformation (settling or subsidence). Of note is that the axis along which axial deformation occurs is different from the one along which pure subsidence (vertical height loss) occurs (along a plumb line; Fig. 29.2). Both angular deformation and axial deformation contribute to subsidence (loss of vertical height).

Specifically regarding the spine, the concept of subsidence is not new. As previously mentioned, the fact that the spine “collapses” during the aging process has been known for centuries. Spine surgeons have similarly known for years that interbody fusions are often complicated by the “pistoning” of interbody grafts into vertebral bodies, which in large part is related to angular deformation (e.g., kyphosis). Kyphosis contributes to a loss of vertical height (see Fig. 29.1 and Fig. 29.2) and therefore is a component of subsidence. This process involves a combination of (1) pistoning of the strut into the vertebral bodies, (2) collapse (shortening) of the graft (strut) itself, and (3) poor carpentry. Poor carpentry results in persistent gaps that “encourage” subsidence or, even worse, persistent gaps between the strut and the end plate. The latter may lead to nonunion.
A substantial body of information regarding settling and subsidence has been accumulating. This is predominantly of note regarding the cervical spine. In this chapter, therefore, most of the discussion focuses on the cervical spine, most specifically the ventral cervical spine.
Hughes and colleagues have observed that significant subsidence occurs following ventral cervical fusion procedures and that this is related to the number of levels fused (see Table 29.1). 3 Subsequently, Bishop and colleagues corroborated these findings regarding ventral cervical discectomies. Furthermore, they demonstrated that allografts subsided and angled more than autograft fusions in single and multi-level fusions (Table 29.2 and Table 29.3). 4 Graham and colleagues have shown that rigid spinal implants may retard, but do not eliminate, the kyphotic deformation process. 5 Therefore, the subsidence process appears to be inevitable.
Number of levels | Associated loss of height |
Two levels | 7.6 mm |
Three levels | 8.08 mm |
Four levels | 14 mm |
Source: From Hughes et al. 3 |
Allograft | Autograft | |
Collapse | 2.4 mm | 1.4 mm |
Angulation | 2.8 degrees | 1.4 degrees |
Source: From Bishop et al. 4 |
Allograft | Autograft | |
Collapse | 3.0 mm | 1.8 mm |
Angulation | 4.1 degrees | 1.9 degrees |
Source: From Bishop et al. 4 |
As stated, “deformity begets deformity” and “kyphosis begets kyphosis.” As such, aging results in the loss of an initially robust ventral disc interspace height (Fig. 29.3a, b). This in turn results in straightening (loss of lordosis) of the spine (see Fig. 29.3b) and the creation of a moment arm that encourages further kyphotic deformation (Fig. 29.3c).

29.1 Spine Deformation
In general, the spine “wants” to deform, even in the presence of previously placed interbody strut grafts. 6 This is related to the inevitable and obligatory effects of the aging process, gravity, and repetitive loading—predominantly the repetitive axial impulse loading associated with ambulation. It is perhaps useful to think of spinal deformation as occurring in one (or more) of three planes: (1) rotation (about the long axis of the spine), (2) axial deformation (deformation occurring along the neutral axis), and (3) angular deformation (deformation occurring about an instantaneous axis of rotation [IAR] in the sagittal or coronal plane). Rotation about the long axis of the spine is difficult to assess and quantitate. Furthermore, it is not of clinical significance in most instances in the subaxial cervical spine. Extreme rotation, and the locking of a facet joint, is an obvious exception.
As previously stated, both axial deformation and angular deformation occur naturally as part of the degenerative process (see Fig. 29.3). Both are often exaggerated following corpectomy and interbody strut grafting, as well as following dorsal decompression via laminectomy. Also, both axial deformation and angular deformation contribute to the loss of vertical height (subsidence; Fig. 29.4).

The natural history of cervical spondylosis and the degenerative aging process is frequently associated with a cervical kyphosis. This is not ideal. Kyphosis affects adjacent segments adversely. 7 , 8 The operative decision-making process for patients with myelopathy patients is often dictated and guided by the extent of the kyphosis. Finally, a stable angular deformity accelerates end-fusion degenerative changes. 9 , 10 This can be prevented or minimized by the attainment and maintenance of sagittal balance. 11
In general, most surgeons perform ventral procedures for ventral pathology (particularly if a kyphosis is present) and a dorsal procedure for dorsal pathology (particularly if a lordosis is present). An intermediate spinal configuration (straightened spine) may be approached surgically from either a ventral or a dorsal approach. Some have recommended a laminectomy-plus-fusion approach for the latter patient population (see Chapter 4 and Fig. 29.5). It is emphasized that the attainment and maintenance of cervical lordosis should be one of the goals of any cervical spine decompression procedure. This pertains to the lumbar spine, as well.

29.2 Dynamic Spine Stabilization: A Historical Perspective
Spinal column deformation in the form of subsidence, particularly in postoperative patients with osteoporosis, is exceedingly common. This occurs in both successful and unsuccessful cases. Therefore, striving to prevent subsidence may be unwise. An implant that permits some deformation, but that limits the extent and controls the type and trajectory of the deformation, may be desirable. This concept is not new. Dynamic hip screws have been employed for femoral neck fractures with success. They allow the femoral neck to “shorten” or “collapse” along its axis so that the bone (femoral neck) “sees” adequate or optimal bone healing–enhancing forces (e.g., compression; Fig. 29.6). This, as well as other “dynamic” techniques, uses the phenomenon of subsidence and Wolff’s law to advantage. As previously stated, subsidence, to one degree or another, is inevitable.

Wolff’s law (initially published by Wolff in 1892) 12 , 13 describes the phenomenon associated with the response of bone to stress. This “law” has been interpreted and translated many times since its initial presentation. The modern interpretation, as stated in the American Heritage Medical Dictionary (copyright © 2007, 2004 by Houghton Mifflin Company), is perhaps the clearest and most succinct:
“The principle that every change in the form and function of a bone, or in the function of the bone alone, leads to changes in its internal architecture and in its external form.”
Wolff’s law suggests that bone loading alters bone integrity and bone healing. A corollary of Wolff’s law is that bone heals optimally when exposed to compressive loads. Interestingly, Yasuda et al 14 demonstrated that bone has piezoelectric properties, such that it can generate electric potentials in response to mechanical stress. When bone (in vivo) is exposed to compressive loads, a negative charge is observed over its surface via the aforementioned piezoelectric effect. This in turn stimulates osteoblastic activity, among other physiologically favorable parameters regarding bone healing. Hence, the compression of bone induces bone healing à la Wolff’s law via the electrophysiologic properties first observed by Yasuda and colleagues.
In the case of a femoral neck fracture treated with a dynamic hip screw, the femoral neck axially deforms along a trajectory defined by the surgeon (along the “axis” of the femoral neck, as defined by the location of the hip screw). This axial deformation increases the compressive loads applied to the bone fragments, which in turn enhance the chance of healing (see Fig. 29.6). These concepts are applicable to spine surgery, as well. Weiss developed a spring with hooks for attachment to the dorsal thoracic and lumbar spine. This was subsequently modified by Larson (Fig. 29.7). 15 When combined with ventral interbody fusion, this technique provides compressive forces that both encourage subsidence (by compression) and simultaneously increase bone healing–enhancing forces.

Spinal implants that are not rigid (all implants except fixed moment arm cantilevers and three- and four-point bending devices) provide some degree of dynamism. Early cervical spine dynamic implants included Caspar plates, lateral mass plates (nonfixed moment arm cantilevers—for example, screws that toggle), and cerclage wiring techniques (tension-band fixators). More modern techniques employ these same principles in a more sophisticated manner (Fig. 29.8). They permit a significant transfer of load through the bone graft (load sharing), thus encouraging fusion. The nonfixed moment arm nature of the screws, however, causes degradation of the screw–bone interface with cyclical loading, even when bicortical purchase is achieved. 16 This engenders significant concern with respect to unstable spines or long constructs. Caspar attempted the overt “allowance” of axial deformation with the use of paired slotted holes, but this was subsequently abandoned. 17

Rigid techniques for ventral spine stabilization subsequently achieved popularity. These employ fixed moment arm cantilevered screws (Synthes, DePuy Spine, Raynham, MA; Orion [Sofamor-Danek, Memphis, TN]; and others (Fig. 29.9). The extent of their rigidity is quantifiable. 18 The greater the rigidity (stiffness) of an implant, the smaller the fraction of the load transmitted to the graft. 19 , 20 Ventral cervical implants vary considerably regarding the extent of rigidity imparted to the spine. Problems related to excessive rigidity have been observed, opening the door for a resurgence of enthusiasm for dynamic implants. The Codman system (Codman & Shurtleff, Raynham, MA) is such an implant (Fig. 29.10). It permits screw toggling, and therefore also spine deformation. It is purported not to require bicortical purchase. 21 Fixed moment arm cantilever systems, however, have been shown to be associated with fewer complications than nonfixed moment arm systems; hence the aforementioned resurgence of more rigid systems. 22


An advancement regarding controlled subsidence technology came in the form of the ABC system (Aesculap Implant Systems, Center Valley, PA). It provides a similar advantage by allowing screws to toggle via a nonfixed moment arm cantilever beam strategy. It also uses paired slots that permit axial subsidence. This concept was previously abandoned by Caspar. 17 The DOC system (DePuy-AcroMed, Raynham, MA), 23 , 24 the ABC system, 25 and the Premier plate (Sofamor-Danek, Memphis, TN) permit controlled dynamism (Fig. 29.11). Controlled dynamism is defined as the encouragement of permissive axial deformation along a predetermined trajectory. With such strategies, the surgeon permits subsidence by controlling or dictating the trajectory along which it occurs. Such techniques permit, but limit, the amount of axial deformation (subsidence). This has also been accomplished in the thoracic and lumbar spine. 15 , 26

This is not unlike the situation in which the orthopedic surgeon applies a dynamic hip screw to a fractured femoral neck (see Fig. 29.6). In this case, the surgeon permits subsidence, but along a trajectory that he or she chooses. Hence, the close approximation of bone is achieved, and bone healing–enhancing forces (à la Wolff) are encouraged.
Buttress plates permit axial and angular deformation. They are occasionally used to minimize the incidence of caudal bone graft kickout. 27 , 28 This strategy may provide the simplest form of dynamism. However, it also permits angular deformation (see Chapter 28). Of note is that caudal kickout is most significant at the cervicothoracic junction, where the spine angles significantly. The effects of this regional change in geometry have been studied biomechanically. 29
29.3 Dynamism
Dynamism is herein defined as permissive spine deformation. The term implies that an implant “permits” or allows the spine to deform. However, the deformation is assumed to be controlled. This is accomplished by permitting only desirable, and not permitting undesirable, deformation, as well as by limiting the extent of desirable deformation (e.g., axial deformation)—hence the term controlled dynamism (the encouragement of permissive axial deformation along a predetermined trajectory). 30
Dynamism can be achieved via two fundamental mechanisms: (1) the employment of absorbable implants and (2) the use of implants that themselves deform. These mechanisms differ fundamentally. An absorbable implant permits spinal deformation only after the integrity of the implant is lost (by absorption). Therefore, absorbable implants permit deformation late (e.g., after 1 to 2 months), whereas deformable implants permit spine deformation immediately. After surgery, subsidence occurs early (1 to 2 weeks after surgery; Fig. 29.12). 23 – 25 , 30 – 40 Of additional note is that to be strong enough to appropriately resist spinal deformation, the absorbable implants considered to date must be excessively thick.

Deformable implants, as stated, permit deformation immediately. They permit one or both of two types of spine deformation: (1) angular deformation and (2) axial deformation. Angular deformation may be permitted by nonfixed moment arm cantilevers (Fig. 29.13), by tension-band fixation devices (Fig. 29.14), 41 or by failure of fixed moment arm cantilevers ( Fig. 29.15 ). Axial deformation may be permitted by slotted plates, collapsible plates, screws that slide along a rod or plate, or sliding platforms (Fig. 29.16). 23 , 24 A variety of dynamic fixation cervical strategies have been compared biomechanically. 42 Each differs mechanically. Dynamic plating allows greater load transfer through the graft 43 both angular and axial deformations are permitted by kick plates (Fig. 29.17). Kick plates, however, allow angular deformation and do not absolutely eliminate kickout. In fact, catastrophic kickout may be associated with their use. A situation such as that depicted in Fig. 29.17d may result in asphyxia related to trachea compromise. 44





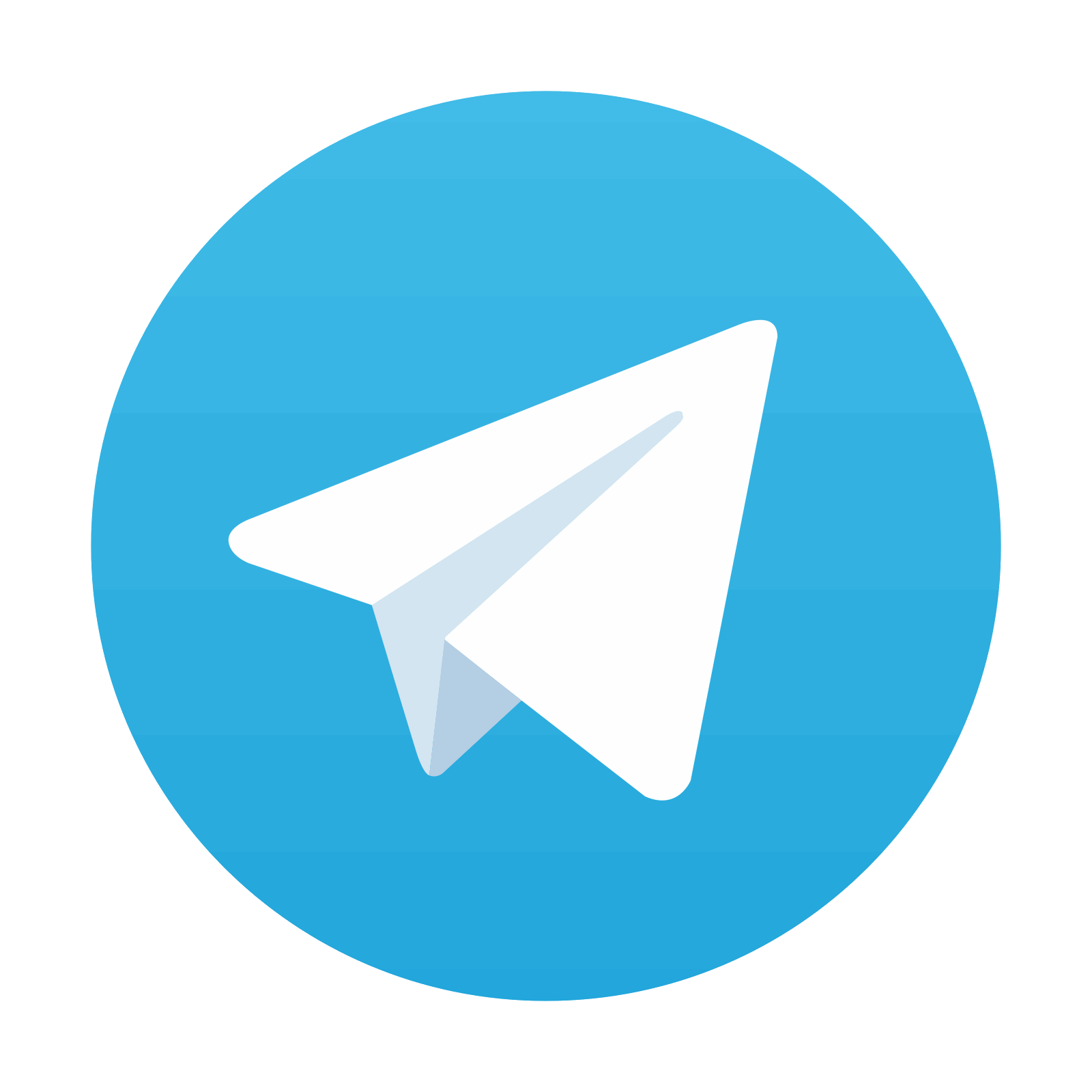
Stay updated, free articles. Join our Telegram channel

Full access? Get Clinical Tree
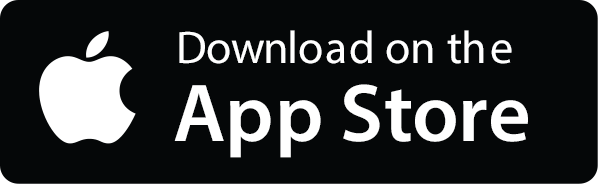
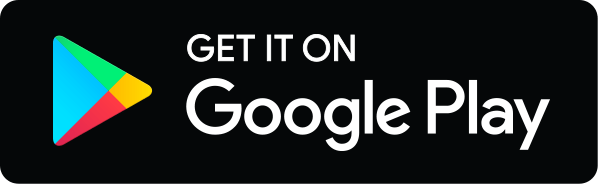