3 Development of the Human Brainstem and Its Vasculature
Abstract
Safe and efficient surgery in and around the brainstem depends on a thorough understanding of the development of the human brainstem and its vasculature. This chapter reviews the development of the human brainstem, including embryonic and postnatal developmental milestones, with an emphasis on the developmental mechanisms of tracts and nuclei. The work also highlights both the vasculogenesis and the angiogenesis of the human brain that lead to formation of blood vessels in this part of the brain. Surgery in and around the brainstem requires a thorough understanding of developmental anatomy. An indepth understanding of this anatomy can be used to safely traverse tracts and nuclei to remove pathology while minimizing the disruption of surrounding structures.
Introduction
The cerebral hemispheres (cerebrum) are adjoined to the cerebellum and spinal cord by the most primordial portion of the central nervous system (CNS), the brainstem and the diencephalon ( Fig. 3.1 ). 1 , 2 These structures control the flow of motor and sensory information between the brain and the rest of the body while also controlling breathing and modulating heart rate and blood pressure, in addition to wakefulness and numerous other essential bodily functions. The brainstem lies in the anterior portion of the posterior cranial fossa with its ventral surface abutting the clivus, the basilar part of the occipital bone. It is continuous with the forebrain at the level of the tentorial notch and with the spinal cord at the foramen magnum. The brainstem comprises an ectodermally derived nexus of neuronal cell bodies (gray matter) that form nuclei and axon fibers (white matter) grouped into tracts (i.e., fasciculi, pedunculi, and lemnisci). These internal structures give rise to the characteristic surface anatomy and surgical landmarks of the human brainstem.

Clinical neurosurgery requires an understanding of the art of neurology (the “méthode anatomo-clinique” of Jean-Martin Charcot) and the principles of the neurosciences, as well as an appreciation for embryology begetting regional and functional neuroanatomy. Thus, a thorough understanding of the embryology and anatomy of the brainstem, and of the development of its nearby structures, is essential in the diagnosis, operative planning, and treatment of numerous pathologic conditions of the CNS, especially those involving the brainstem and its vasculature. In this chapter, we explore the embryologic principles guiding the development of the human CNS, with special emphasis on the brainstem and the formation of its network of vessels through vasculogenesis.
Early Embryogenesis
From Zygote to Blastocyst
After spermatozoon–oocyte fusion, a single-celled zygote undergoes a series of mitotic divisions to give rise to a 16-cell morula (Latin mōrus for “mulberry”). At this stage, the 16-cell morula consists of compressed blastomeres, which undergo the process of blastulation ( Fig. 3.2 ). 4 Through compaction and rearrangement, this early blastula forms the inner cell mass (embryo-blast) that differentiates into epiblast and hypoblast layers (i.e., the bilaminar [or two-layered] germ disc), the outer trophoblast layer of cytotrophoblasts and syncytiotrophoblasts, and the discrete blastocele cavity. 3 The inner cell mass gives rise to the tissues of the developing embryo, whereas the trophoblasts are responsible for the formation of the embryonic placenta. Subsequent divisions, continued differentiation, protease digestion, and hatching from the surrounding zona pellucida allow the blastocyst to successfully implant into the richly vascularized endometrium of the uterus approximately 10 to 11 days after fertilization.

Gastrulation
At the beginning of the third week of embryonic development, the cells begin to organize into distinct germ layers through gastrulation. Gastrulation is the process by which the bilaminar embryonic disc reorganizes into a trilaminar structure called the gastrula. The primitive streak is formed at the beginning of gastrulation (days 15–16), and it appears as a narrow groove of pluripotent cells flanked by protruding regions at the caudal midline of the embryo ( Fig. 3.3a ). 3 , 5 The primitive streak is restricted caudally by the cloacal membrane (future site of the anus) but progressively increases in length and forms a thickening at its cranial-most end called the primitive node (Hensen node). 6 , 7 The primitive node is also aptly called the organizer by embryologists because it regulates several important processes such as laterality and notochord formation, thereby making it the primary initiator of the developing CNS.

Cells of the epiblast migrate toward the primitive streak and, upon arrival, detach from the epiblast to form an intermediate layer (the mesoderm) between the epiblast and hypoblast layers ( Fig. 3.3b ). 8 In addition, some of these migrating cells displace the existing hypoblast to create the embryonic endoderm, while others remain in the epiblast layer, forming the overlying ectoderm. 8 This inward movement (i.e., invagination) represents the earliest stage of the trilaminar embryo. Later in gastrulation, epiblast cells migrating more posteriorly form the paraxial mesoderm, lateral plate mesoderm, and extra-embryonic mesoderm sequentially. 3 , 8 As more cells migrate from the epiblast layer to the hypoblast layer, they begin to increasingly spread in the cranial and lateral directions of the developing embryo, thereby contributing to subsequent stages of embryogenesis.
Early Development of the CNS
Formation of the Notochord
The notochord is the defining structure formed in all chordate embryos that is required for the patterning of surrounding tissues. Its formation begins with the ingression of a population of epiblastic cells into the primitive streak during gastrulation, where they become prenotochordal cells at the primitive node and continue to move cranially toward the prechordal plate (oropharyngeal membrane, the future site of the mouth) ( Fig. 3.3a ). 3 , 9 As the hypoblast is replaced by endoderm cells, the cells of the notochordal plate separate from the surrounding endoderm and proliferate. 3 Ultimately, this proliferation results in the formation of a solid, but flexible, cord-like cellular structure termed the definitive notochord. The notochord serves phylogenetically and ontogenetically as the original support along the longitudinal axis of the developing embryo, and it serves a central role in the transformation of undifferentiated embryonic cells into definitive tissues and organs. Specifically, through inductive signaling, the notochord stimulates conversion of the overlying surface ectoderm into neural tissue (i.e., neural plate), it specifies floor plate cells within the developing CNS, and it transforms certain somitic mesodermal cells into vertebral bodies, thereby contributing to the formation of the axial skeleton. 10 , 11 , 12 Although the notochord is an embryonic anatomical structure critical for the development of the human CNS, it is largely transient, being lost in the adult human, with the exception of its contribution to the nucleus pulposus of the intervertebral disc. 13
Neurulation
The human brain begins modestly as a flat sheet of ectodermally derived cells at the dorsal midline of the embryo. In response to inductive notochord signals (i.e., relief of bone morphogenetic protein-4 [BMP-4] inhibition by chordin, noggin, and follistatin), this sheet of cells thickens to form a large placode called the neural plate around day 17 of embryonic development. 14 , 15 , 16 , 17 The neural plate undergoes a phase of rapid growth, leading to the formation of a neural groove with neural folds on each side along the longitudinal axis of the developing embryo. Through continued cellular proliferation, the neural folds move closer together and ultimately fuse at the dorsal midline, thus forming the neural tube ( Fig. 3.4 ). 18 This process by which the neural tube initially forms is called neurulation. Moreover, neural tube fusion begins at the midcervical level and proceeds in both cranial and caudal directions. 3 , 19 The cells forming the walls of the neural tube constitute the neuroepithelium, which will give rise to the entire CNS. At this point in embryonic development, the neural tube has two temporary openings, the cranial and caudal neuropores, which communicate with the amniotic cavity ( Fig. 3.5 ). 3 With the exception of notable pathologic conditions (e.g., anencephaly and spina bifida), the cranial neuropore closes by the middle of the fourth week, and the caudal neuropore closes by the end of the fourth week. 20 , 21 The final position of the cranial neuropore is represented by the lamina terminalis in the adult brain. 22


Neural Crest
As the neural folds combine to form the incipient neural tube, cells located along the lateral margins of the neural plate break free to form the neural crest, which is a grouping of cells between the neural tube and the overlying ectoderm ( Fig. 3.4 ). 18 These bilaterally paired neural crest cells arise through induction from adjacent nonneural ectoderm and mesoderm, causing them to change their shape and properties compared with other neigh-boring ectodermally derived tissues. 18 In particular, these cells lose their cell-to-cell adhesiveness through decreased expression of cell adhesion molecules, thereby permitting a migratory phenotype. 8 , 23 As the CNS continues to develop, some populations of neural crest cells remain posterolateral to the neural tube, giving rise to sensory ganglia of the spinal nerves (dorsal root ganglia) and the cranial nerves (CNs) and to the ganglia of the autonomic nervous system. 24 Other populations of neural crest cells migrate along well-defined pathways to differentiate into several elements: neural (e.g., Schwann and satellite cells of the peripheral nervous system, meninges of the spinal cord, and enteric nerve plexus), neuroendocrine (e.g., chromaffin cells of the adrenal medulla and parafollicular cells of the thyroid), and nonneural (e.g., melanocytes and craniofacial bones). 25 , 26 , 27 , 28
Development of the Primitive Brain
Even before neural fold closure, the neural plate is noticeably larger at the cranial end of the developing embryo. At this stage of development, the major divisions of the developing brain become increasingly evident. As previously mentioned in the section on neurulation, the brain begins as a flat sheet of cells, but through the processes of neurulation and differentiation, the human CNS becomes increasingly complex and functionally specialized. By the end of the fourth week, the cranial end of the neural tube has three distinct swellings that correspond to the three primary brain vesicles: the cranial-most prosencephalon, or forebrain; the mesencephalon, or midbrain; and the caudal-most rhombencephalon, or hindbrain ( Fig. 3.6, Table 3.1 ). 24 , 29 During the fifth week, the secondary vesicles develop from the prosencephalon and rhombencephalon, creating a total of five secondary vesicles: the cranial-most telencephalon, the diencephalon, the mesencephalon, the metencephalon, and the caudal-most myelencephalon ( Fig. 3.6, Table 3.1 ). 24 , 29 Each of these secondary vesicles undergoes distinct developmental changes as brain development progresses, thus driving further structural and functional specialization.

Coinciding with the rapid shape changes of the developing brain, the lumen of the neural tube develops into the ventricular system of the mature brain and the central canal of the spinal cord ( Fig. 3.6 ). 22 , 29 In the adult brain, the lateral ventricles of the cerebral hemispheres communicate with the third ventricle of the diencephalon via the interventricular foramen (foramen of Monro). The rhombencephalon contains the fourth ventricle and is situated between the cerebellum posteriorly and the brainstem anteriorly. The fourth ventricle communicates with the superior third ventricle via the cerebral aqueduct (aqueduct of Sylvius) of the mesencephalon. By the fifth month of development, openings in the roof plate (the two lateral foramina of Luschka and the foramen of Magendie) establish communication of cerebrospinal fluid between the ventricular system and the subarachnoid space. 29
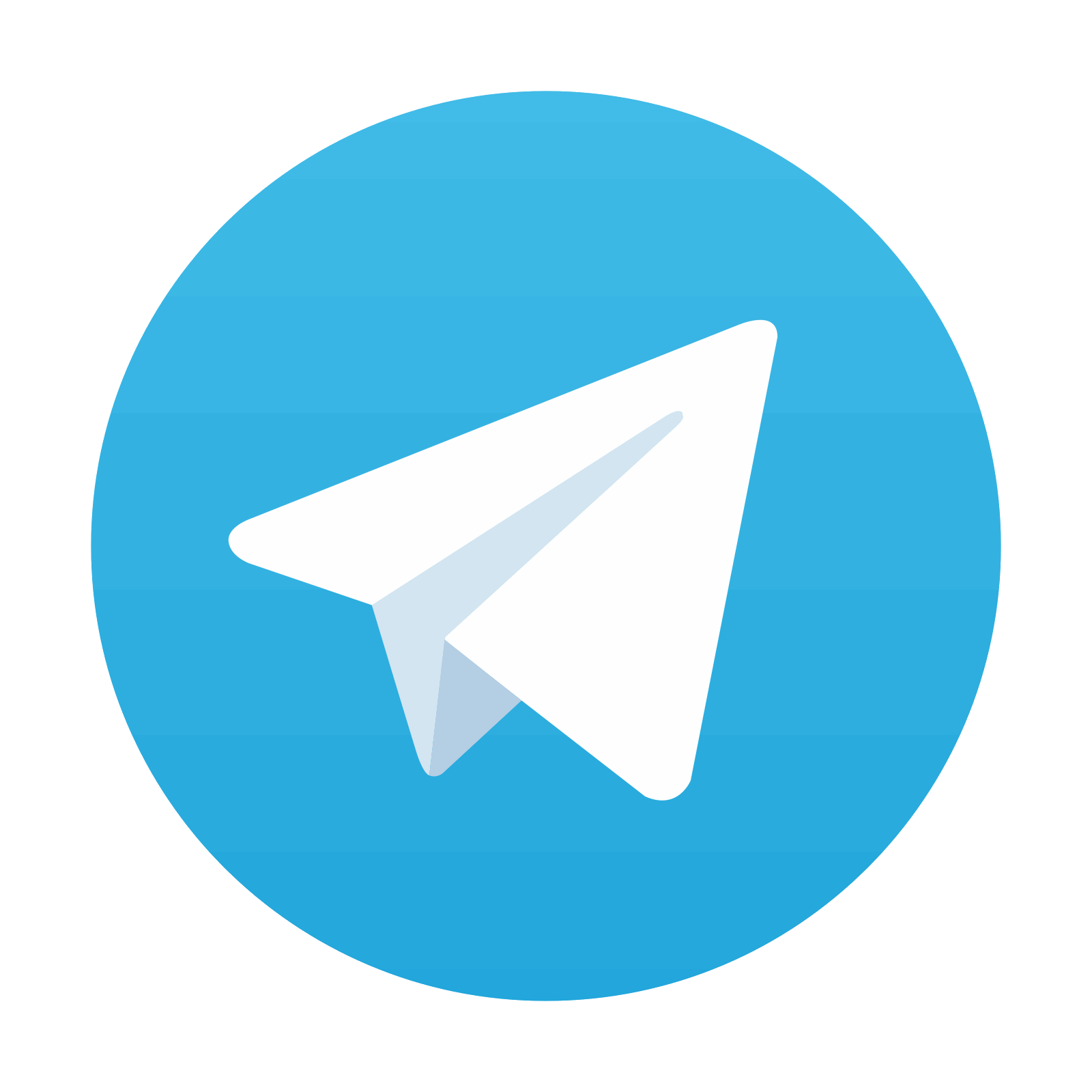
Stay updated, free articles. Join our Telegram channel

Full access? Get Clinical Tree
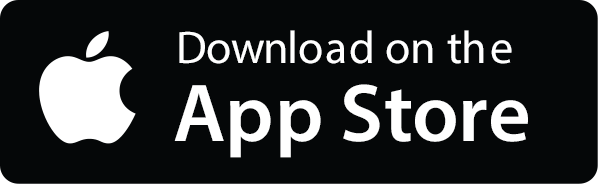
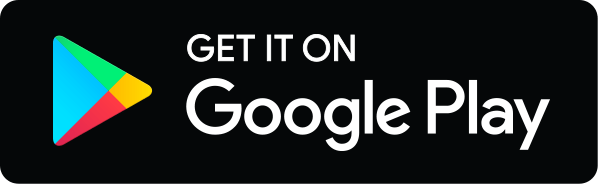
