3 From the Head to the Feet: Anatomy of the Upright Position
Abstract
The sagittal anatomy of the vertebral column cannot, at present, be studied in an isolated fashion. The surgeon should include it together with the skeleton, from the head to the feet in the standing position. This is characteristic of the biped Homo sapiens. Studies must comprise balance during movement, primarily walking. This chapter (1) summarizes animal phylogenesis to review the various mechanisms of implementation of this fragile upright posture, which is difficult to maintain comfortably because of the aging of the vertebral column; and (2) describes the various ways to evaluate this henceforward classic sagittal balance, in particular with the EOS system without forgetting to consider the behavior of the spine during ambulation. The authors describe the systems of compensation in pathological situations.
3.1 Phylogenesis
3.1.1 The Skull
From an embryological and descriptive point of view, the skull is very different from vertebrae. However, Jean Dubousset coined the terms “cranial vertebra” to include the skull—situated above the cervical spine—together with the vertebral column. It is demonstrated in this chapter the phylogenetic evolution that animals developed so that the gaze of quadrupeds and, later, that of bipeds would be horizontal.
Numerous angles have been described by anthropologists to study the course of the shape of the skull according to animal species and in the evolution of hominids. These angles are employed to clarify the orientation of the great occipital foramen (or foramen magnum) and of the eye sockets. The occipital angle of Broca (Fig. 3‑1) is measured between the line joining the nasion (point situated at the nasal root) and the opisthion (posterior edge of the great occipital foramen) and a line joining the same opisthion to the basion (the anterior edge of the foramen magnum). This angle decreases from a skull of a quadruped (45°) to a human skull (10°). The decrease in the occipital angle of Broca corresponds to a horizontalization of the foramen magnum, which could be designated “intracranial horizontalization.” This horizontalization is very marked when comparing the skeleton of primates, which presents a whole spine kyphosis (cervical, thoracic, and lumbar kyphosis) and a human skeleton, which presents lumbar and cervical lordosis (Fig. 3‑2). The second angle described by anthropologists is the orbito-occipital angle drawn between the axis of the eye and the line joining the basion and the opisthion. This angle, with 63° to 90° in quadrupeds, have lower values of 30° to 69° in monkeys and reaches 20° in modern humans. Beauvieux, an anatomist from Bordeaux, clearly demonstrated that the nasion–opisthion line is parallel to the external semicircular canal of the internal ear (Fig. 3‑3). In agreement with that, one may consider that the nasion–opisthion line is the reference line of the horizontal plane. The axis of the eye (and consequently that of the gaze) is directed 30° downward and forward. This gaze directed 30° anteriorly and downward corresponds to the reference position of the head as proposed by many ergonomists (Fig. 3‑4).




From the posterior to the anterior in the skull base, the foramen magnum locates more posteriorly in chimpanzees, with intermediary position in Australopithecus, and to Pithecanthropus and being located more anterior (or less posterior) in Homo sapiens. This morphology is in line with the direction of greater leverage of the trapezius muscle to augment its action of stabilization of the head 1 (Fig. 3‑5).

3.1.2 The Pelvic Ring
The phylogenetic evolution of the shape and orientation of the various constituents of the pelvic ring (sacrum, iliac wings, ischium, and pubis) has been extensively described notably in the other chapters of this book. Nevertheless, it is necessary to review the main concepts.
Tardieu et al 2 studied the 3D anatomy of the pelvic ring of 19 infant and 50 adult hominid fossils. These authors investigated the evolution of the shape of the hips and sacrum during acquisition of biped walking and during growth. They used two angles, pelvic incidence (PI) 3 and an original angle they called “bow angle” (designated here as the iliopubic angle) limited by the line joining the middle of the sacral endplate and the middle of the acetabulum or the femoral heads (FHs) and the line from the middle of the FHs and the pubis in front (Fig. 3‑6). This angle, which increases with the width of the pelvis in front, increased during the evolution of primates toward bipedalism in the same way as PI, which increases with the width of the pelvis.

According to Tardieu et al 2 and Morvan et al, 4 the iliac wings widen and become more sagittal (Fig. 3‑7) during the evolution of bipedalism. Thus, the pelvis enlarges in its anteroposterior (AP) dimensions (accounting for the increase in PI) (Fig. 3‑8), grows anteriorly (accounting for the increase in iliopubic angle), and opens upward to better support internal organs and the trunk.


Tardieu et al 2 studied the pelvic ring of the Australopithecus afarensis named Lucy, that lived 3 million years ago. The authors observed that the pelvis was retroverted, with a small PI and a shape and orientation of the iliac wings intermediate between chimpanzees and H. sapiens (Fig. 3‑9).

Recently, Schlösser et al 5 introduced a new angle, the ilioischial angle (Fig. 3‑10), between the line connecting the middle of the sacral endplate to the center of the acetabulum and a line along the middle of the ischium. This angle decreases with the acquisition of bipedalism and also with growth. It decreases with the increase of the width of the posterior part of the pelvis to supplement the “bow angle” of Tardieu et al, 2 which increases with the increase of the width of the pelvis in the front and the PI angle, which increases with the increase of width of the pelvis in the middle. The decrease in the ilioischial angle tends to increase the leverage of hamstrings, indispensable to maintain the femurs extended while standing.

The sacrum—the base of the ship’s mast that is the spine (Fig. 3‑11)—represents one-seventh of the spine’s height. It has widened and gained in height during evolution of hominids until H. sapiens. The sacrum also evolved by curving anteriorly (Fig. 3‑12). Abitbol 6 used the angle of the sacral curve between the line following the anterior wall of the S1 vertebral body and the line following the anterior wall of L5. The sacrococcygeal angle (Fig. 3‑12) described by Marty et al 7 is drawn between a line perpendicular to the upper endplate of S1 and a line perpendicular to the endplate of S5. According to Tardieu et al, 2 this angle increases with the acquisition of bipedalism (Fig. 3‑12, Fig. 3‑13).



During the acquisition of bipedalism, anatomical modifications such as the widening and sagittal orientation of the iliac wings, the anterior curving of the sacrum, along with the increase in lumbar lordosis are explained, according to Tardieu et al, 2 by the action of the extensor muscles (lumbosacral muscles, glutei, and hamstrings) but also, notably for the sacrum, by the tension of the strong sacrospinal ligaments (Fig. 3‑13, Fig. 3‑14, Fig. 3‑15).


3.1.3 The Spinal Curvatures
Cervical and lumbar lordosis are specific to bipedalism. In 1957, Goff and Landmesser 8 demonstrated this by transforming rats into bipeds by amputating the forelimbs and obliging the rats to feed on their hindlimbs to survive by placing their feeders high. Long-term spine X-rays of these rats rendered bipedal showed an increase in the cervical lordosis and a flattening of the lumbar kyphosis (Fig. 3‑16).

Hominids such as gorillas or orangutans have the ability to remain upright. However, because of the lack of lumbar lordosis combined with a very flat pelvis inducing a PI inferior to 10°, they are forced to maintain an almost seated position with marked retroversion of the pelvis and flexion of the knees. These two compensatory phenomena are observed in human pathology when there is substantial loss of lumbar lordosis and severe anterior imbalance. The four-legged gait most often used by nonhuman hominids is facilitated by the length of their forelimbs (or upper limbs) with a much higher intermember index than in humans. Lumbar lordosis and pelvic anteversion are intimately linked and maintained thanks to the action of the paravertebral muscles and the gluteus maximus, making the contour of the buttocks characteristic of the real biped H. sapiens (“only humans have buttocks” said Buffon). The lumbar lordosis is lacking in other hominids, which have flat buttocks.
3.2 The Aging Process of the Spine
The aging process is inexorable and may be accelerated by genetic and especially mechanical influences (i.e., repetition of professional or sporting activity constraints of the spine). The intervertebral disk begins to dehydrate very early, at the age of 18 years old. There may occur a reduction of the physiological lordosis (Fig. 3‑17) estimated at 25° in L5-S1, 15° in L4-L5, and 10° to 12° in the upper lumbar spine. Indeed, the aging of the lumbar spine is “propagated” from bottom to top in the whole spine, as a result of the hinged position of the lower segments L5-S1 (and even L4-L5) relative to the pelvis (Fig. 3‑18). This results in a very significant loss of lordosis between L4 and S1, where two-thirds of the lumbar lordosis is located. The same phenomenon exists in the cervical spine with loss of disk height initially occurring in the lower cervical disks (C7-T1, C6-C7, and C5-C6) because of a tightening effect and extended preservation of the height of the upper disks, which often remain hypermobile notably in extension to maintain a horizontal gaze.


In parallel with the loss of intervertebral disk height, which contributes to lumbar or cervical kyphosis, there is an enlargement of the facet joints that creates posterior bulk, limiting the straightening (or extension) of the patient in the same way as the increase in height of lumbar spinous processes (Fig. 3‑19), as demonstrated by Aylott et al, 9 using a longitudinal computed tomography (CT) study.

The aging of paravertebral muscles is characterized by a loss in the number of both type 1 and type 2 muscle fibers. Atrophy of muscle fibers is also observed and especially affects type 2, fast twitch fibers. Degenerative change or fatty involution is a natural aging phenomenon of muscles. It was quantified in the lumbosacral muscles by Hadar et al 10 who described three stages of fatty degenerative changes in posterior muscles:
Stage 1: less than half of the cross sectional area of the affected muscles.
Stage 2: 50% fatty transformation.
Stage 3: more than 50% fatty tissue (Fig. 3‑20).
Fig. 3.20 Muscular fatty degeneration with the three stages of Hadar.
The fatty involution progresses from the deepest zone toward the surface, the multifidus being the first muscle affected, and also from below upward (i.e., from the lumbosacral junction toward the thoracolumbar junction). Cruz et al 11 demonstrated a direct correlation between aging, loss of lumbar lordosis, and degree of fatty involution of the paravertebral muscles.
Fortin et al 12 conducted a longitudinal magnetic resonance imaging study of multifidus over 15 years and observed an atrophy more marked in L5-S1 than in L1-L2 with fatty replacement independent of physical activity (work or sport) but dependent on the body mass index. In patients with degenerative (or arthrogenic) kyphosis, signs even more marked; we found almost complete disappearance of type 2 fibers, with severe fibrous and fatty involution involving both lumbar and thoracolumbar muscles, and an abnormal number of moth-eaten, core or targetoid, and ragged red fibers. All these histopathologic signs are observed in myopathies 13 (Fig. 3‑21).

The aging processes affecting disks, facet joints, ligaments, and muscles lead to a progressive loss of lumbar lordosis. It may cause limitation of bipedalism with obligation for aging subjects to use mechanisms of compensation. The first is pelvic retroversion, then flexion of the knees and, when these two means are insufficient, canes may be necessary. Senile osteoporosis, leading to kyphosis-induced vertebral compression fractures, which often occur one after the other like dominoes, only exacerbates this anterior imbalance.
The mechanisms of compensation 14 for the control of the balance in the sagittal plan include the following:
Cervical hyperlordosis.
Displacement of the cranial center of gravity.
Extension of the vertebral column.
Retroversion of the pelvis.
Extension of hips.
Flexion of the knees.
Flexion/extension of the ankles.
Inversion/eversion of the feet.
Compensations involving the lower limbs are very often overlooked by the clinical examination and imaging studies.
Two strategies of compensation are most typically adopted by the patient, depending on the level and type of thoracic kyphosis, whether there exists a reserve of lumbar extension (in some cases at a single level), and, above all, the residual capacity of the gluteal and hamstring muscles. In some patients with proximal thoracic kyphosis, with preservation of lumbar lordosis (or with a single abnormally mobile lumbar segment), and when the muscles are still somewhat effective, the compensation strategy adopted by the patient consists in straightening the spine as much as possible while maintaining hips and knees extended and flexing the ankles (Fig. 3‑22, Fig. 3‑23, Fig. 3‑24a). The trade-off is the head/neck segment in a position of R1 retraction, but fatigue can also lead to loss of a horizontal gaze. Only a certain degree of hip and knee flexion may then restore it.



The most usual strategy employed—when there is no possibility of lumbar extension—consists in compensating for the thoracolumbar kyphosis and for the posterior pelvic tilt by using maximum femoral extension (while on imaging studies, one might conclude in a flexion of the hips). Knees and ankles are stabilized in flexion. This posture lowers the center of mass very effectively. A horizontal gaze is consequently possible (Fig. 3‑22, Fig. 3‑23b). However, if the patient extends the knees, this results in anterior imbalance (Fig. 3‑23b).
When the vertebral curvatures become worse and the cervical and thoracolumbar extensor muscles become weaker, the horizontalization of the gaze becomes impossible (Fig. 3‑23c). The neck is forced into P1 protraction. The line of gravity then falls in front of the base of support. An anterior support becomes indispensable (Fig. 3‑23c).
Osteoarthritic stenosis of the spinal canal will lead to more symptoms in extension (or lordosis) than in flexion (or kyphosis), because regardless of the level of the spinal canal, the AP diameter decreases in extension and increases in flexion. At the same time, according to nerve root dynamics, the rootlets and roots advance toward the vertebral bodies and disks in flexion and move backward toward the posterior arch in extension (Fig. 3‑24, Fig. 3‑25). This explains the exacerbation of compressive osteoarthritic neck and arm pain in extension of the neck and the improvement of neurogenic claudication caused by lumbar stenosis in flexion or lumbar kyphosis. Standing radiographs in extension without (Fig. 3‑25) or with injection of contrast (myelography; Fig. 3‑26) demonstrate this dynamic compression.


The kyphotic changes of the vertebral column may cause compression of the neurological elements, primarily the cauda equina in the narrow lumbar canal. Neurogenic claudication following stenosis is aggravated in extension. Some cases of anterior imbalance can be improved by simple root decompression.
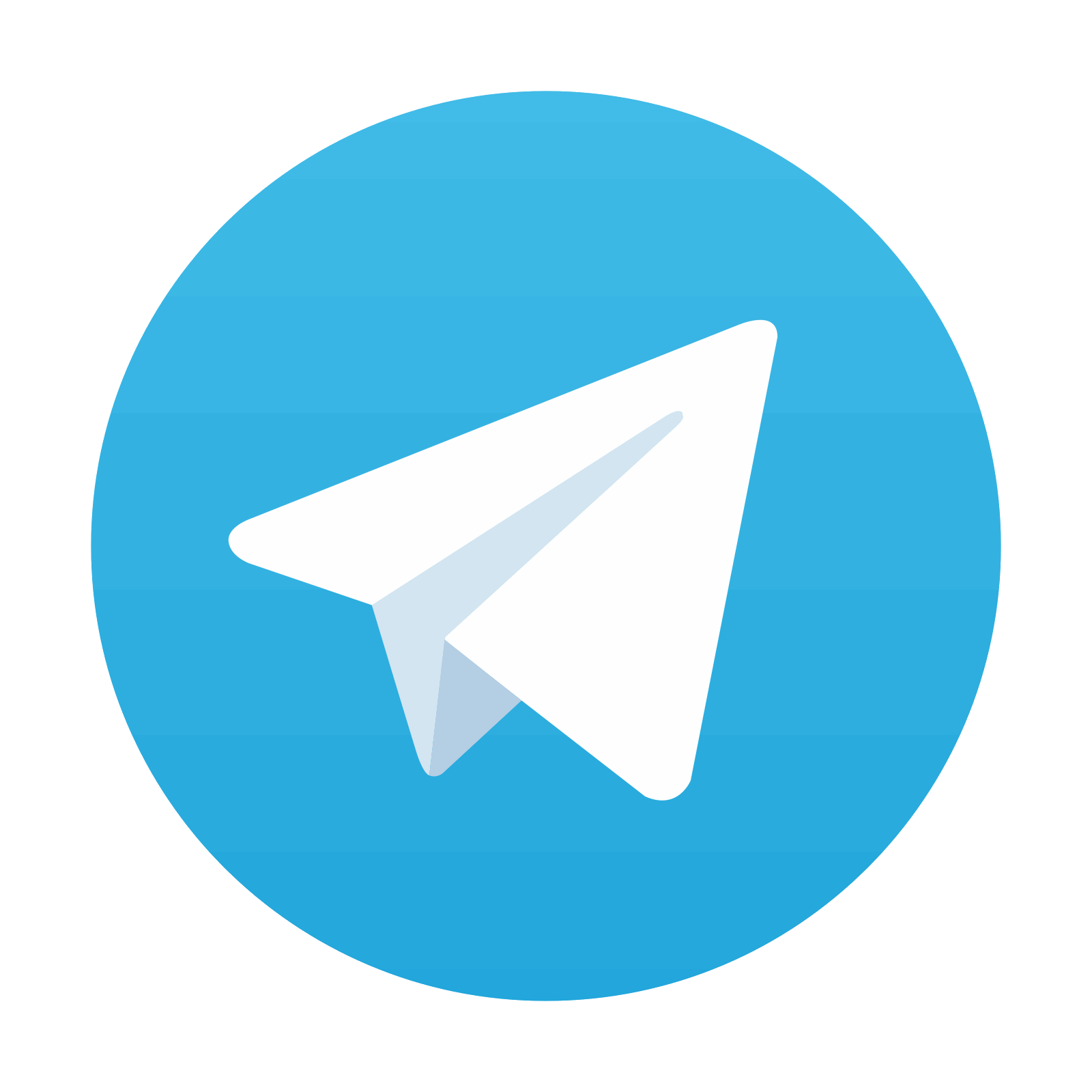
Stay updated, free articles. Join our Telegram channel

Full access? Get Clinical Tree
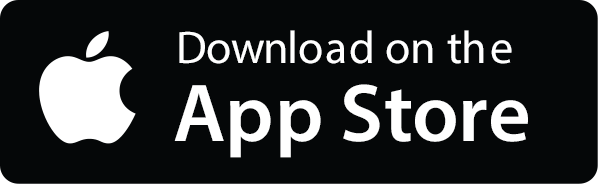
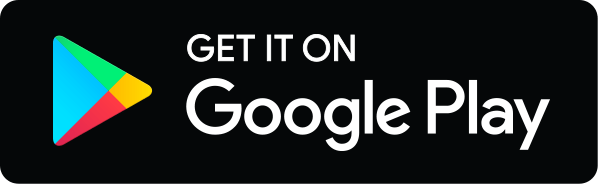