3 Intraoperative Ultrasound in Spine Surgery: A Versatile and Useful Adjunct
Abstract:
Intraoperative ultrasound (IOUS) has been used in spine surgery for more than 30 years. Modern devices are able to provide real-time images of exceptionally high resolution and precise delineation of anatomical structures. This easy-to-use and effective intraoperative tool provides spine surgeons with excellent real-time visualization of the spinal cord and nerve roots as well as of neurocompressive structures. Thus, IOUS is a useful adjunct for guidance and confirmation of adequate surgical interventions in a multitude of spine pathologies such as spinal tumors, spine trauma, degenerative, congenital, and vascular spine disease.
3.1 Introduction
The utility of ultrasound in the field of medicine was first recognized by brothers Karl Theodore and Friederich Dussik in the 1930s and 1940s. These pioneers attempted to scan human brains through the skull by using a 1.5-MHz transmitter and proposed that ultrasound might be able to diagnose brain tumors. 1 Research by Wild led to the use of A-mode imaging with a 15-MHz transducer to visualize and determine bowel wall thickness and gastric cancer in the 1950s. 2 “Amplitude” or A-mode ultrasound was the earliest ultrasound imaging method and described a one-dimensional image displaying the amplitude or strength of a wave along a vertical axis and the time along the horizontal axis. By the late 1940s “brightness” or B-mode ultrasound was developed and was able to obtain two-dimensional (2D) images of tissue where each pixel on a screen represented an individual amplitude spike, relating brightness to the ultrasound wave amplitude. Over the following decades with better physical, physiological, and engineering understanding, the technology of ultrasonography was further improved and advanced. As large and cumbersome ultrasound machines were transformed into smaller and more portable devices, the intraoperative use of ultrasound started to gain popularity. By the 1980s, first reports of intraoperative ultrasound (IOUS) in spine surgery as a valuable adjunct for real-time visualization of various pathologies such as tumors, cysts, and syringomyelia were published. 3 , 4 , 5 , 6 Dohrmann and Rubin recognized that by performing a laminectomy and placing the ultrasound probe directly on the dura, they were able to obtain higher quality images since ultrasound waves were not compromised by impenetrable bone or narrow interlaminar windows. 3 , 7
Many spine surgeons today routinely use real-time IOUS to more precisely tailor the extent of bone resection and to more safely guide resection of intradural and intramedullary tumors while preserving normal tissue. Additionally, IOUS can help confirm adequacy of decompression of lesions ventral to the thecal sac such as in the case of calcified thoracic disk herniations or retropulsed bone fragments in spine trauma. 8 , 9 , 10 With recent advances in the field of three-dimensional intraoperative computer-assisted navigation, the primacy of IOUS for real-time image guidance has been somewhat supplanted by CT- and/or MRI-based navigation systems in many areas of spine surgery such as for intradural tumors. 11 However, ultrasound still remains the most widely used guidance modality today, due to its availability, relatively low cost, easy operability, and ability to provide real-time visualization with high-spatial resolution.
3.2 Basic Technical Principals of Ultrasound
Ultrasound refers to the transmission of energy as mechanical pressure waves within matter with frequencies above 20 kHz, which is above the human audible range. In order for these waves to propagate, ultrasound essentially requires a physical medium; it cannot be transmitted in a vacuum. In biological tissue with high water content, ultrasound waves propagate mainly longitudinally at an average speed of 1,540 m per second. In medicine, ultrasound frequencies used generally range from 2 to 20 MHz with very short wavelengths allowing for high spatial resolution. As a general rule, the higher the frequency, the higher the spatial resolution and the lower the ultrasound penetration becomes. Conversely, with lower frequencies, spatial resolution is decreased and penetration of the ultrasound beam is increased. Frequencies in the gigahertz (GHz) range can be used for ultrasound microscopy to visualize subcellular structures. The “piezoelectric effect” refers to the capability of quartz crystals or synthetic ceramics within the ultrasound transducer to intermittently deform under pulsatile electrical stimulation. This sudden and rhythmic structural deformation generates mechanical vibration, and ultimately the ultrasound. Piezoelectric transducers are able to transform electrical signals into mechanical waves (ultrasound transmitter), and also to change reflected (“echoing”) ultrasound waves back into electrical signals (ultrasound receiver). Ultrasound waves may be partially absorbed by biological tissue and energy is then transformed into heat. As the ultrasound beam propagates across different tissues with different acoustic impedances, interfaces are created. Depending on the acoustic impedance at an interface, the ultrasound waves may be partially or completely reflected, or absorbed and transformed into heat. Only returning echoes are picked up by the transducer, which ultimately constitutes the source of the diagnostic information and contributes to the ultrasound image.
3.3 Ultrasound Modes and Techniques
3.3.1 B-Mode Ultrasound
B-mode ultrasound is certainly one of the most widely used ultrasound applications in medicine. As briefly mentioned earlier, B-mode (brightness-mode) refers to a 2D ultrasound image composed of different dots representing ultrasound echoes. The amplitude of the reflected ultrasound signal (the echo) defines the brightness of each dot. All of these different dots allow for visualization and quantification of anatomical structures as gray scale images.
The intrinsic brightness of these structures is described in terms of their echogenicity, which is compared relative to the surrounding tissue. In general, hyperechoic tissue is brighter, and hypoechoic tissue is darker. If two different tissues are equally bright, they are isoechoic to one another. When the ultrasound beam has no tissue interface to reflect upon, such as within a fluid, the representation of that medium is anechoic and appears dark (black). For instance, pure water will appear black, whereas the presence of red blood cells within the water will appear as bright echogenic specs.
3.3.2 Doppler Ultrasound
Doppler ultrasound is the combination of the Doppler effect with measurement of both direction and velocity by color scale within gray scale (B-mode) images. While the Doppler effect assesses whether objects (usually red blood cells) are moving toward or away from the transducer, color Doppler has the ability to quantify velocity of objects. In spinal surgery, this modality is often used to identify the presence and direction of flow in abnormal vessels and to confirm successful surgical disconnection of dural arteriovenous fistulae (DAVF; see below).
3.3.3 Contrast-Enhanced Ultrasound
Both B-mode and Doppler ultrasound have the limitations of not being able to detect microvasculature. Also, signal detection may often be insufficient due to artifacts and compromised by background noise, especially for deep-seated lesions. Furthermore, with Doppler only one or a few vessels can be studied at a time with the signal changing as soon as the insonation angle is altered. These drawbacks have led to the development of ultrasound contrast media given to the patient intravenously to amplify signals reflected from regions of interest. Contrast media used today are suspension of microbubbles (mean diameter of 5 μm), which modify the acoustic impedance of tissues and the interaction with the ultrasound beam. This leads to increasing echogenicity and blood backscattering, since the insonated microbubbles themselves will emit ultrasound in a radial fashion. Thus, contrast-enhanced ultrasound delivers high contrast images, and it is a very useful technique for assessing tissue blood perfusion.
3.4 Intraoperative Use of Ultrasound
For the use of IOUS, a mobile ultrasound machine that can easily be operated by the surgeon in a sterile fashion is necessary. Nowadays, specifically designed transducers allow for high-resolution digital imaging of anatomical structures. Depending on the site and extent of bony removal, the spine surgeon may choose from various ultrasound transducers, such as convex or linear probe tips with frequency ranges from 3 to 13.33 MHz. Modern high-frequency transducers up to 18 MHz exist for small or superficial spinal lesions. The linear “hockey stick” spinal cord guidance transducer or the convex transducer with a small footprint of 20 mm is well suited for use in spine surgery (Fig. 3‑1).

Following sufficient bony removal through a hemi- or full laminectomy, the dura overlying the surgical region of interest (e.g., the tumor) is exposed. Transdural standard B-mode direct sonography will then confirm sufficient or insufficient bony removal above the lesion of interest before opening of the dura.
To obtain optimal imaging quality, the surgical field is filled with saline for acoustic coupling. Irrigation with a laminar flow will help reduce the accumulation of air bubbles, which can compromise the ultrasound image. Also, bleeding into this fluid bath will lower the image quality since erythrocytes are highly echogenic. An appropriate transducer is then placed just above the dura in the saline bath and images should be obtained in both the axial and sagittal planes by gradually moving the probe across the operative field. Tilting the transducer accordingly within the laminectomy site may be necessary depending on the extent of bony removal to view adjacent dura and spinal cord underlying bony structures. Holding the transducer directly on the dura may cause an artifact called “near field clutter,” since structures in the near field can be obscured due to high amplitude of oscillations by the transducer itself. 12 It is advised to always determine the cranial and caudal, or left and right, orientation of the transducer at the beginning. Depending on the transducer, one side is usually clearly marked and corresponds to the left- or right-hand side of the ultrasound monitor. Structures are most clearly seen by sonography when they are in line or perpendicular with the central acoustical axis.
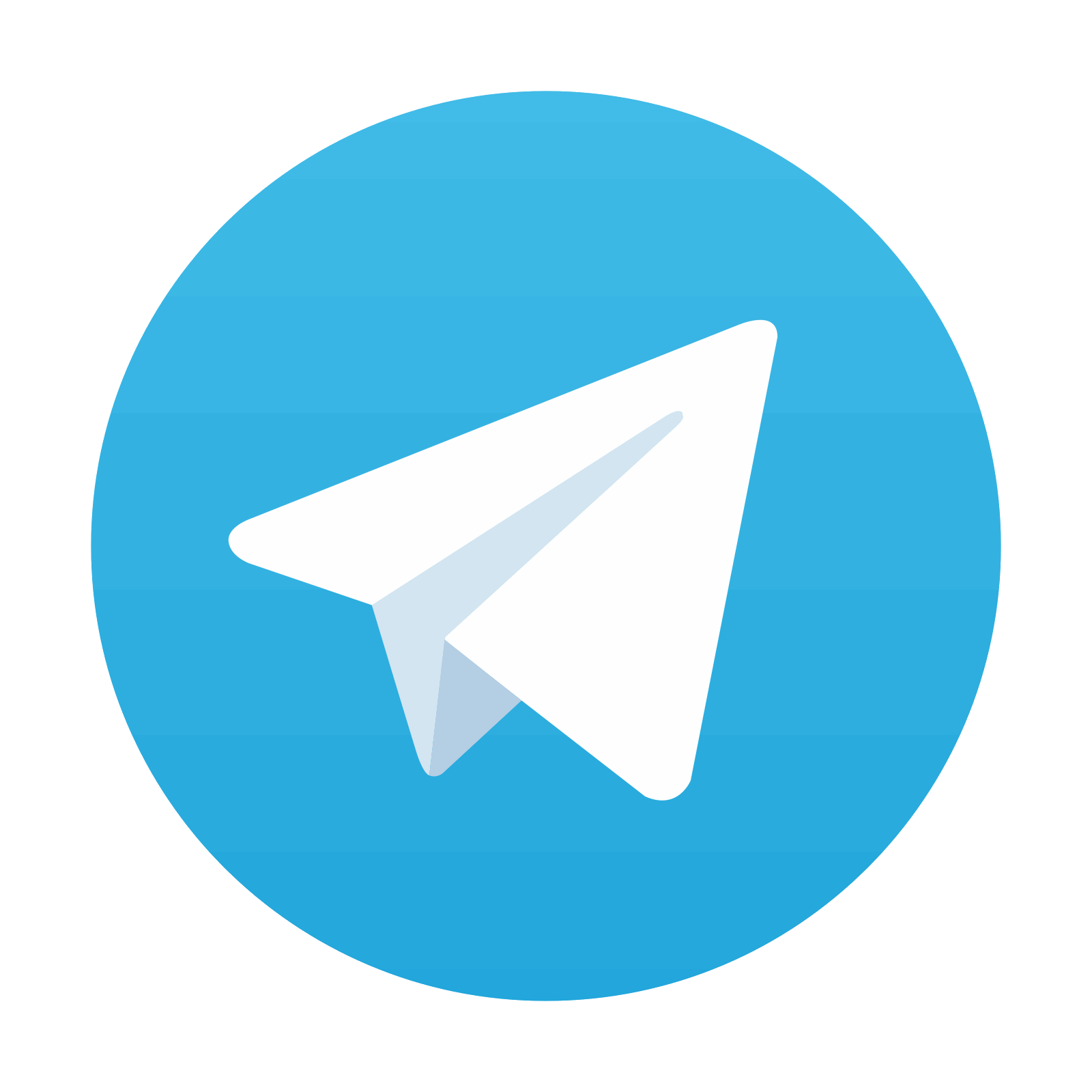
Stay updated, free articles. Join our Telegram channel

Full access? Get Clinical Tree
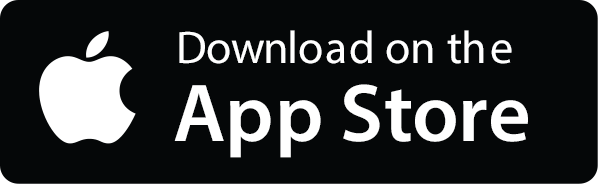
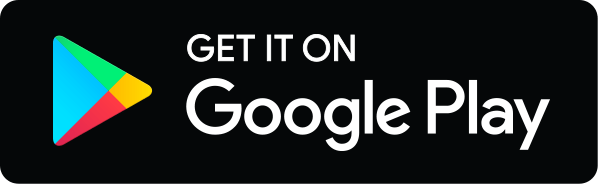