30 Focused Ultrasound as a Surgical Treatment for Epilepsy
Abstract
Transcranial magnetic resonance image–guided focused ultrasound (FUS) is a developing technology that has been used to treat essential tremor, Parkinson disease, chronic pain, and obsessive-compulsive disorder. FUS utilizes stereotactically aligned acoustic energy to create discrete thermal ablations within the brain without making incisions, craniotomies, or transgressing the brain parenchyma. Successful clinical trials among patients with essential tremor have led neurosurgeons and epileptologists to pursue FUS as a potential surgical treatment for focal epilepsy. This chapter discusses the key technological advances and clinical trials in FUS that has led to FDA approval for the treatment of movement disorders. This chapter also discusses the candidate seizure disorders that would be ideal for FUS therapy and potential barriers to the application of epilepsy.
30.1 Introduction
Brain applications for high-intensity focused ultrasound (HIFU) were originally conceptualized and developed in the 1950s as an instrument to create discrete, precise lesions within the brain. 1 , 2 Widespread use of HIFU in the brain was hampered by high levels of energy dissipation at the skull, which resulted in heating of the scalp and cortical surface, and by competing noninvasive modalities such as radiosurgery.
Over the course of the past 20 years, there have been considerable technological advances allowing more efficient transcranial ultrasound delivery to the brain. Modern transcranial ultrasound transducers are arranged hemispherically with hundreds of individual ultrasound elements (▶Fig. 30.1). 3 , 4 , 5 , 6 , 7 , 8 , 9 A hemispherical configuration minimizes skull and soft-tissue heating by distributing acoustic energy over a large surface area. Second, a wave phase correction algorithm was developed to account for the variable thickness, density, and contour of the skull, making it possible to correct alterations in the acoustic wave while traveling through media with large discrepancies in acoustic impedance. 10

Among the first reported uses of modern transcranial ultrasound transducer systems for human brain ablations were medial thalamotomies for chronic neuropathic pain syndromes. Martin et al treated nine patients suffering from a wide array of therapy-resistant pain syndromes with bilateral centro-lateral thalamotomies with a mean initial pain relief of 68%. 11 Jeanmonod et al later reported their longer-term outcomes with an average improvement in pain of 57% at 1 year. 12 These initial studies led investigators around the world to seek additional applications for focused ultrasound (FUS) technology.
Following successful thalamic ablations for the treatment of chronic pain by Martin et al and Jeanmonod et al, three pilot studies were performed to assess the feasibility of using transcranial FUS Vim thalamotomy for the treatment of medication-refractory essential tremor. Elias et al reported a 75% improvement in hand tremor at 1 year as well as an 85% improvement in disability and a 66% improvement in quality of life, 13 and Lipsman et al reported 81% improvement in hand tremor at 3 months. 14 Chang et al reported similar improvements in tremor control among a cohort of 11 patients. 15
These three studies led to a randomized, double-blind control trial at eight international centers that included 76 patients. At 3 months, there was a 47% improvement in hand tremor and sustained 62% improvement in quality-of-life measures. 16 The results of this trial led to FDA approval of FUS for the treatment of essential tremor.
With recent technological advances, early success in pilot studies for tremor, and recent FDA approval, there is renewed interest in HIFU brain applications among neurosurgeons. Currently, ablative HIFU is being investigated as a treatment for Parkinson disease, psychiatric disorders, brain tumors, and a wide array of neurological diseases. 17 Studies consistently demonstrate submillimeter precision with this transcranial lesioning modality 12 , 13 , 15 ; so, treatment for focal epilepsy and palliation of nonlocalized epilepsy would obviously be considered as a neurologic application. Despite encouraging laboratory and feasibility studies, there have been no published clinical trials thus far. In this chapter, we discussed some of the barriers in applying current FUS technology to the treatment of epilepsy, and several candidate epilepsy conditions for FUS treatment.
30.2 Barriers in Transcranial MR-Guided FUS for Epilepsy Treatments
The major limitation of transcranial FUS treatments continues to be transmitting enough energy through the skull. It is estimated that more than 90% of the delivered ultrasound energy is either reflected or absorbed by the skull. 18 Despite advancements in transducer design and skull correction algorithms, some patients enrolled in tremor trials have had failed treatments secondary to inadequate tissue heating at the acoustic focus.
Current transcranial FUS systems optimally deliver acoustic energy toward the geometric center of the skull where the most beams converge. Also, the acoustic focus of the currently FDA-approved midfrequency clinical system is discrete to within a few millimeters. In order to treat focal epilepsy lesions of different locations and sizes, these two factors will have to be considered in planning future clinical trials for epilepsy.
30.2.1 Therapeutic Envelope of Current Systems
As an ultrasound wave propagates from a medium of low density to another of higher density, an acoustic impedance mismatch causes a reflection, absorption, or distortion of energy at the interface of the two media. This phenomenon is especially important for transcranial ultrasound as there is a high mismatch between soft tissues of the scalp and the skull. Incident acoustic waves that are perpendicular to the impedance mismatch are more likely to propagate unimpeded. Thus, the multi-element, hemispherical transducer configuration not only disperses acoustic heating over a large surface area of the scalp but also promotes the likelihood that individual ultrasound elements will interact perpendicularly with the skull.
This configuration establishes the geometric center of the skull as the theoretical optimal target for the most efficient transcranial ultrasound delivery, while peripherally located targets closer to the skull would have less efficient delivery. As such, deeply located epileptic foci such as periventricular nodules or hypothalamic hamartoma are more ideally located for FUS targeting than superficially located lesions such as cortical dysplasia with current transcranial FUS systems (▶Fig. 30.2).

Although this presents a theoretical challenge for treating peripherally located epileptic lesions, there is little direct evidence that excludes these lesions. Furthermore, there are several individualized skull factors that can affect the efficiency of transcranial ultrasound delivery, which could allow for adequate tissue heating of lesions near the skull.
A second consideration regarding the skull and its heating are far-field effects of ultrasound especially regarding targets near the skull base. In a laboratory study, Monteith et al studied skull base heating during modeled treatments for trigeminal neuralgia. Significant heating of the skull base was observed, particularly near the internal auditory canal and adjacent cranial nerves. 19 The authors also found that this could be largely mitigated with careful masking of ultrasound elements analogous to shielding with radiosurgical treatments. 19 This study highlights the importance of far-field ultrasound effects on the skull base and its structures—a factor that will be important for treating the mesial temporal lobe. As the number of transcranial FUS treatments increases, there will be a better understanding of transcranial sonications and refinement of skull simulation algorithms that may allow for further efficiency and safety.
30.2.2 Volume Mismatch in Acoustic Focus and Epileptic Target
Since HIFU heats the brain resulting in thermal ablations, FUS lesions have similar appearance to radiofrequency ablations by histological analysis and by magnetic resonance imaging (MRI). 20 Wintermark et al reported a three-zone appearance on T1- and T2-weighted images. Zones I and II have restricted diffusion and correspond to coagulative necrosis, while zone III represents vasogenic edema and nonpermanent tissue damage. 21
On MRI, FUS lesions evolve in size over time. Wintermark et al reported that zone I + II diameters were 2.7, 4.0, and 2.1 mm at 1 day, 1 week, and 1 month, respectively. 21 Similarly, Chang et al reported FUS lesion size. In their study, the mean lesion volumes were 98, 202, and 217 mm 3 at 1 day, 1 week, and 1 month, respectively. 15
These thalamotomy ablation volumes are sufficient to treat essential tremor and chronic pain, but much larger ablation volumes are likely needed to treat an epileptic focus or control epilepsy. There are a few strategies that could be used to increase ablative volumes during FUS treatments. The first strategy is to simply adjust the ultrasound transducer to ablate adjacent tissue in successive sonications. This has already been reported in the literature. Jung et al have made multiple transducer adjustments to perform bilateral anterior capsulotomies for the treatment of obsessive-compulsive disorder. 22 Although simple, this adds a considerable amount of time to the treatment. The second strategy is to utilize an ultrasound transducer with a larger acoustic focus that can ablate a larger volume. Ultrasound transducers operating with lower parent frequencies can have larger acoustic foci. This technology exists but is not yet approved for clinical use.
Another potential strategy to increase treatment volumes is to use adjuvant administration of intravenous microbubbles during sonications. Arvanitis et al demonstrated that microbubble administration in conjunction with low-intensity ultrasound could result in cavitation-mediated ablations that occupied much high volumes than reported with thermal ablations alone. 23 Although these authors were able to achieve high ablation volumes in a large-brain animal model, there are real concerns regarding cavitation-based treatments and the potential for inertial cavitation and adjacent tissue damage in the brain. Further research is necessary in this field.
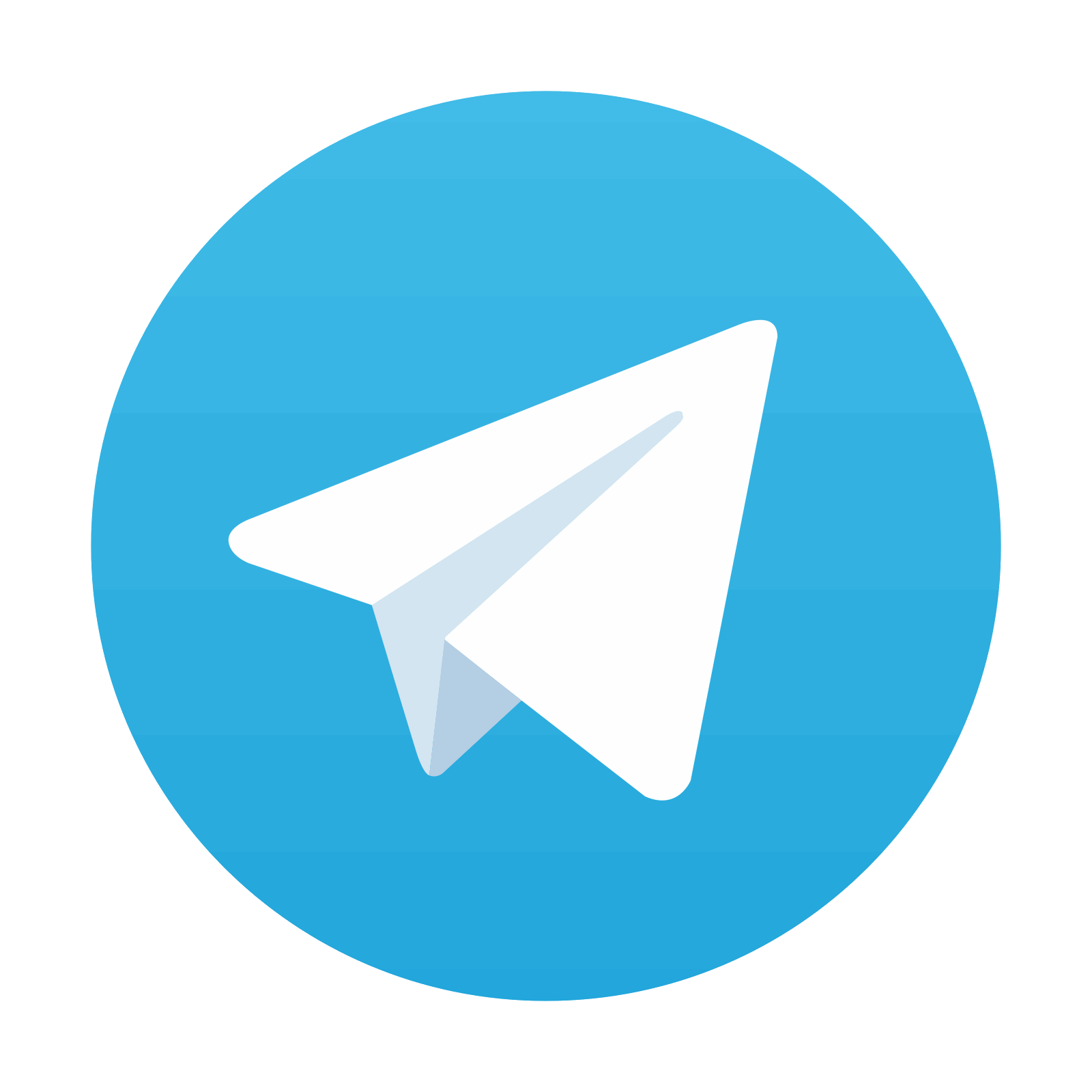
Stay updated, free articles. Join our Telegram channel

Full access? Get Clinical Tree
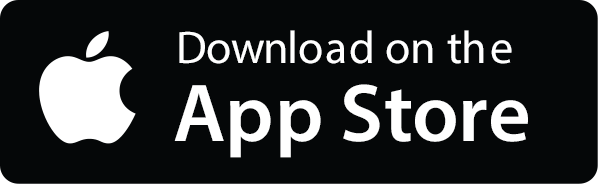
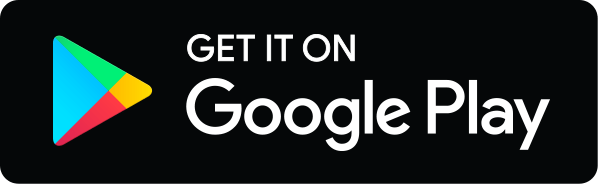
