30 Principles of Endoscope-Assisted Microneurosurgery

30.1 Introduction
Endoscope-assisted microneurosurgery (EAM) evolved in the late 1990s when innovative neurosurgeons adept at neuroendoscopy introduced the endoscope during keyhole cranial microscopic procedures. The realization that the endoscope could deliver superior optic capabilities that augmented microscopic visualization in a minimally invasive fashion stirred excitement because now surgical dissections could be performed effectively in areas previously not accessible by microscopic visualization alone. Prior to this, steady and progressive scientific and technological improvements in optics, magnification, and illumination were occurring and were applied to microscope and endoscope development. The technological advances of these optical instruments paralleled each other but progressed on separate courses. The paths of these two unique optical devices would converge for the first time in the neurosurgical operating suites in the late twentieth century with the introduction of EAM procedures. To appreciate the merits of each optical device and the resultant synergy between them, their independent evolution and subsequent entwinement in neurosurgical history must be understood.
30.2 Historical Evolution of Microscopy and Endoscopy
Early microscopes were built upon principles of optic magnification that can be traced back to Egyptian hieroglyphs from 800 BC.1 Subsequently, the development of refined-lens magnifying glasses in the early tenth century led to the invention of primitive microscopes when lenses were introduced into a sliding tube, presumably in 1590 by a Dutch spectacle-maker named Zacharias Janssen. The term microscopewas coined in the early 1500s by a German papal doctor named Giovanni Fabe by combining the Greek word micro for “small” and scope, referring to the ability to “aim or shoot.” In the 1840s, Carl Zeiss, an ambitious German lens manufacturer, further perfected the lenses and honed stereopsis. The term stereopsis, derived from the Greek word stereo, meaning “solid” and opsis, implying “appearance or sight,” describes the optical principle in which the impression of depth is perceived by two eyes with normal binocular vision.2 As an adjunct to Zeiss’s microscope, a Swedish otolaryngologist named Gunnar Holmgren fastened a light source to the microscope in 1922, thus spurring the revolutionary marriage between magnification and illumination.1 Interestingly, neurosurgeons were slow to adopt illuminated microscopy. Initial introduction occurred only in 1957, when an American neurosurgeon, Theodore Kurze, used an operating microscope to remove a seventh nerve neurilemmoma in a 5 year old. Rapid integration of the microscope into the neurosurgical operative arena ensued, which allowed neurosurgeons to limit their cranial exposures due to more effective illumination, concomitant magnification, and preservation of stereoscopy.3
In the following years, as neurosurgical microscopic experience advanced and the potential attributes of illumination and magnification were realized, neurosurgeons began to search for means to further limit surgical exposures by performing keyhole craniotomies. As explained by Perneczky and Fries,4 keyhole microneurosurgery does not involve performing craniotomies through trephinations the size of a keyhole, rather the craniotomy site is chosen specifically to expose only the amount of brain necessary to perform the given surgical feat, thus precluding as much tissue traumatization as possible. Keyhole craniotomies were executed along predetermined corridors that exposed anatomical windows on the basis of preoperative imaging to facilitate less-invasive microscopic approaches to deep-seated cranial lesions. However, microscopic visualization via keyhole approaches was restricted because of poor illumination potential. A means to enlighten deep, dark corridors and to maneuver around important neurologic structures without manipulation was lacking. Ironically, these key deficiencies in microscopic visualization were the intrinsic advantages in endoscopic optics.
The development of surgical endoscopy likewise progressed over a large span of time. Back in the early to mid-tenth century, Abulkaism of Cordoba and Giulio Cesare Aranzi used closed tubes with mirrors to reflect ambient light into human cavities.1 This primitive means of illumination was further refined in 1806 when Philipp Bozzini, a German physician, created a lichtleiter or “light conductor” that consisted of a tube illuminated by a cradled external wax candle. In 1853, a French surgeon named Antonin Jean Desormeaux designed a kerosene lamp that burned alcohol and turpentine and coupled it to a reflective lens to illuminate the bladder. He eventually coined the term endoscopy, meaning “looking into.” In 1873, Gustav Trouve, a French electrical engineer and inventor, applied electricity via platinum wires, introducing light via a small iridescent airtight bulb at the device’s distal end.5 A German urologist named Maximilian Carl-Fredrich Nitze created a working endoscope from a series of lenses using a platinum filament light at the distal end that provided direct illumination in 1879.6 In 1910, Victor de L’Espinasse, a French urologist, was the first surgeon to utilize the cystoscope for neurosurgical purposes, performing choroid plexus resections in infants with hydrocephalus. In 1923, American neurosurgeon William Jason Mixter performed the first endoscopic third ventriculostomy via a urethroscope. Around this time, Walter Dandy, one of the epic American neurosurgical pioneers in neuroendoscopy, referred to as the “father of modern neuroendoscopy,”7 readily adopted the endoscope into his surgical practice, advanced its technology, and expanded its application. However, during the mid-nineteenth century, the innovation of cerebral spinal fluid shunting and the neurosurgical preoccupation with the microscope diverted attention away from endoscopy. Renewal of interest occurred in the late 1960s, when British optical physicist Harold Hopkins developed the rigid rod-lens system, revitalizing neuroendoscopy by introducing enriched color, higher resolution, and superior illumination through the creation of his optical apparatus.8,9 Neurosurgical recognition of the endoscope’s enhanced illumination, magnification, and visualization capabilities catapulted its application to expanded neuroendoscopic indications in the 1970s and 1980s, as evidenced by the myriad of publications involving large series of endoscopic cases.6
In the late 1990s, key innovative neuroendoscopists, fueled by the optical deficiencies of the microscope and driven by the need for enriched visualization, began introducing the rigid endoscope into the operative field during keyhole microscopic craniotomies. The introduction of the endoscope allowed for improved visualization of key structures without requiring further dural and bony resection. Ideal because of its superior light intensity and its ability to be more easily controlled as it maintained its shape securely in tight operative chambers, the ridged rod-lens endoscope was preferred over fiber-optic systems that provided “exceptional maneuverability at the cost of visualization.”4,10 At this point in history, the synergy inherent between the endoscope and microscope was recognized.
30.3 Principles Governing Microscopy and Endoscopy
An appreciation of the application of EAM lies in understanding the technical advantages as well as the shortcomings associated with magnification, visualization, illumination, and ergonomic qualities of each optical apparatus (Table 30.1). Interestingly, the benefits of one apparatus complement the innate drawbacks of the other. For example, microscopes equip the neurosurgeon with excellent stereotactic, three-dimensional (3D) magnification. Thus, the spatial relationship of the microinstruments to the neuroanatomical field is easily appreciated as it is naturally perceived through the microscope’s maintenance of stereopsis. Visualization capabilities are also superior due to the increased resolution afforded by the microscope’s larger lens diameter as well as the direct transmission of the image to the retina through the microscopic lens.11 However, microscopic illumination has its drawbacks owing to the manner in which the light is delivered, along with the natural physical properties governing light intensity. Light from a microscope enters the surgical field (from a practical perspective) in a conical fashion10 (Fig. 30.1a). Light emitted from the microscopic aperture serves as the base of the cone. As the light travels through the space between the aperture and the surgical field, conical tapering occurs as the parallel light rays are then obstructed by the opening into the surgical field. Even fewer rays enter the operative window due to obstruction by surgical instruments. This mechanical illumination deficiency is further augmented by the natural physical property of light intensity. Due to the inverse square law of light (intensity µ[directly proportional symbol] to 1/distance squared), the intensity of light deep in the field is lessened.12 Thus, even the rays that eventually form the “tip of the cone” are less intense due to the distance they must travel. Finally, the microscope only illuminates structures that appear directly in front of the lens, thereby precluding visualization of the neuroanatomical space deep in the surgical corridor. Owing to the delicate nature of these structures, manipulation is often contraindicated, thus limiting further dissection.

a Note: Advantages for one optical instrument are in bold. The synergy in use of both instruments is because in each case, what is a advantage for one instrument is a disadvantage for the other.
Conversely, the rigid neuroendoscope using the Hopkins II rod-lens system (Karl Storz GmbH & Co. KG, Tuttlingen, Germany) emits light in a divergent fashion, similar to a flashlight10 (Fig. 30.1b). Consequently, it provides a wide-angled vantage point resulting in a more panoramic view that is useful in visualizing adjacent structures and affords the neurosurgeon the benefit of a better anatomical perspective for orientation purposes. The endoscopic viewing angle can be extended by using angled endoscopes, reflecting light 30º, 45º, or 70º degrees rostral in the field (Fig. 30.1c). In addition, the endoscope can be rotated to help illuminate concealed areas, which facilitates further exploration into deep crevices within the field (Fig. 30.1d). Endoscopic illumination and extended viewing angles allow the neurosurgeon to explore the anatomical landscape lateral and posterior to structures so that the neurosurgeon can, in essence, “look around the corner” without anatomical manipulation, which can be damaging to such delicate and vital anatomy.13 Furthermore, the endoscope is more ergonomically sound as it does not require constant refocusing because the lens is located at the tip of the illumination aperture. However, shortcomings of the endoscope lie in the quality of its visualization, when compared with microscopic viewing, due to the indirect transfer of the image through the video camera of the endoscope and subsequently to the retina. Even modern cutting-edge, high-definition images generating 1080 pixel lines and more than 2 million pixels do not offset this lack of quality.11 The endoscopic view also lacks depth, as the image is captured in a monocular fashion by the endoscope camera and is presented to the retina after it is displayed in a two-dimensional manner on a monitor. This lack of stereoscopy in endoscopic optics manifests as the “fish eye effect” in which structures closer to the camera appear larger than more distant objects. With movement, this “fish eye effect” propagates “motion parallax,” which is manifest in the perception that objects adjacent to the endoscope appear to move more than those distal to it.11 As a result of the loss of stereoscopy and thus the natural perception of depth that is afforded by normal binocular vision, the endoscopist must learn to interpret imaging differently. Through visual cues extracted from an understanding of the “fish eye effect” and “motion parallax,” he or she learns the relationship of objects to the endoscope and adjacent structures can extrapolate their location within a theoretical 3D space.
This coalescence of microscopic and endoscopic technology, resulting in the expansion of visualization through small corridors along with the refinement of magnification in deep fields, has advanced the neurosurgeon’s abilities to work effectively, operating in tight, deep corridors. Visual integration of the microscopic and endoscopic viewing fields can be managed by alternation or fusion technique.4 Alternation of the viewing fields occurs when the surgeon’s attention must be divided between the microscopic ocular field and the endoscopic video image as projected upon an ancillary monitor positioned adjacent to the microscope (Fig. 30.2). This conventional method, although less expensive, is certainly not as ergonomic compared with fusion options. Moreover, some consider alternation methods slightly more hazardous as the surgeon must switch attention and body stance to move away from the microscopic oculars to visualize the endoscopic viewing monitor (Fig. 30.2). In contrast, fusion methods demonstrate the viewing fields within a “picture-in-picture” mode on either a screen placed in front of the surgeon, on head-mounted liquid-crystal display (LCD) screens, or, as more recently developed, within microscope oculars.10,14 Through the fusion technique, the merits of each optical apparatus can be integrated more seamlessly and safely. The neurosurgeon can preferentially tailor his or her attention without moving or changing stance to the more ideal optical image during specific portions of the procedure. Further, this amalgamation of technology, coupled with the application of stereotactic neurosurgical techniques and modern imaging, has further enriched neurosurgical precision during EAM procedures.

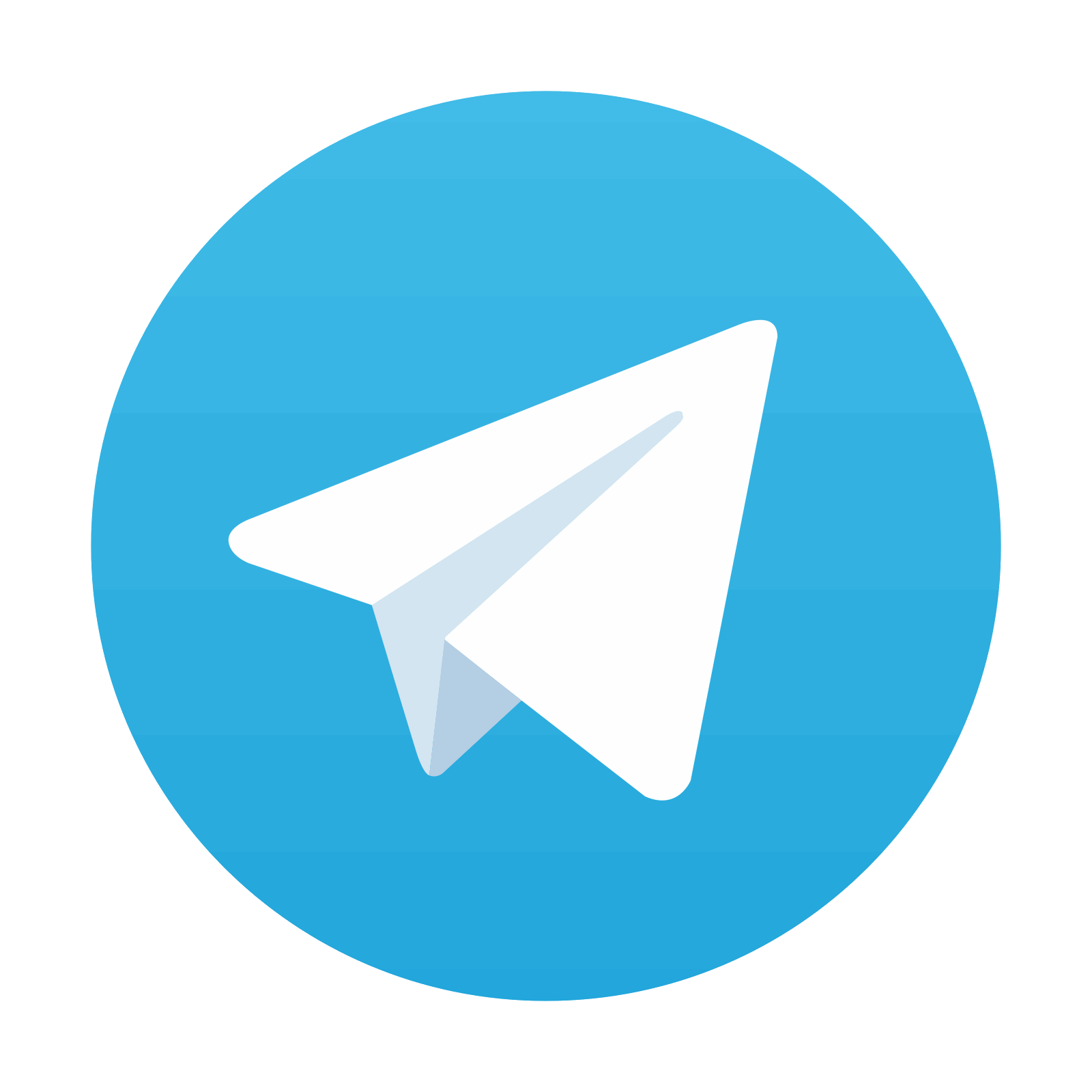
Stay updated, free articles. Join our Telegram channel

Full access? Get Clinical Tree
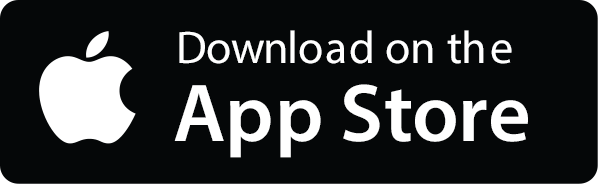
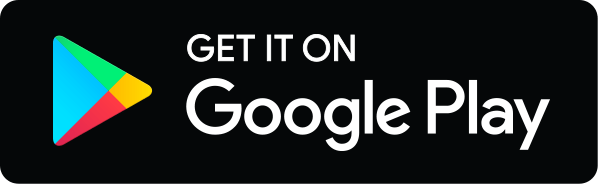