Abstract
Spinal vascular malformations are a rare but treatable cause of spinal cord hemorrhage, myelopathy, and associated morbidity. Both clinical presentation and ideal treatments for such lesions vary widely, primarily due to variations in anatomy and associated angioarchitecture. A variety of mechanisms including venous hypertension/congestion, hemorrhage, vascular steal, and mass effect underlie the mechanism of arteriovenous shunting in spinal vascular malformations and their associations with neurologic insult and decline. Given their complexity, many attempts have been made to classify such malformations, with two major systems (the American/French/English connection and the Spetzler system). With the advent and evolution of both microsurgical and endovascular techniques, the ability to diagnose and manage such lesions through conservative management, radiotherapy, microsurgical extirpation, and endovascular obliteration continues to evolve.
Key words
spinal vascular malformations – dural arteriovenous malformations – Foix-Alajouanine syndrome – spinal arteriovenous fistulas – dural arteriovenous fistulas30 Spinal Dural Arteriovenous Fistulas and Malformations
30.1 Goals
To review the literature that forms the basis of our understanding of the natural history of spinal dural arteriovenous fistulas (dAVFs).
To provide clinically relevant details regarding the anatomy, clinical presentation, and radiographic features unique to spinal arteriovenous fistulas (AVFs), their diagnosis, and subsequent management via endovascular and open techniques.
To review the major publications that classify presentations of spinal AVFs based upon location, angiographic features, and other variables.
30.2 Case Example
30.2.1 History of Present Illness
A 30-year-old Caucasian woman presents to the emergency department with progressive, episodic lower back pain with radiation to her neck and bilateral hips. She notes a history of low-grade lower back pain over the past several years but notes particularly discomfort over the past 24 hours with concomitant photophobia and headache. She denies any recent illness, exposure to ill contacts, or exotic travel. She also denies any other neurologic symptoms.
Past medical/surgical history: Negative for history of prior intracranial hemorrhage, hypertension, polycystic kidney disease, collagen vascular disease, or blood dyscrasias.
Family history: Negative for any history of cerebral aneurysms or other intracranial pathology.
Social history: Employed as local schoolteacher. Denies use of tobacco or illicit substances.
Review of systems: Negative with exceptions as aforementioned.
Physical examination: Notable for photophobia and reported 7/10 headache. Fully alert and oriented. Cranial nerves II—XII intact. Strength 5/5 in all testable upper and lower extremities with exception of 4/5 strength in bilateral hip flexors. 2 + deep tendon reflexes in biceps and Achilles distribution. Evidence of negative Brudzinski sign but positive Kernig sign. Sensation grossly intact to light touch but evidence of difficulty with proprioception in bilateral lower extremities.
Laboratory studies: Lumbar puncture performed within the emergency department is notable for positive subarachnoid panel but without evidence of leukocytosis or albuminocyto-logic dissociation. Complete blood cell count, electrolyte panel, and coagulation studies otherwise within normal limits.
Imaging: Noncontrast head computed tomography (CT) negative for evidence of acute intracranial pathology. Magnetic resonance imaging (MRI) of the thoracolumbar spine demonstrates prominent flow voids dorsal to the conus medullaris at T12 (Fig. 30.1a).
30.2.2 Treatment Plan and Follow-up
Based upon irregularities noted above concerning for possible spinal vascular malformation, the patient underwent formal diagnostic spinal angiography that demonstrated a conus medullaris arteriovenous malformation (AVM) fed by the left Til intercostal, right LI, and right L2 intercostal arteries (Fig. 30.1b-d). The patient subsequently underwent T12-L1 laminectomies for resection of the AVM (Fig. 30.1e). She was discharged on postoperative day 3 to inpatient rehabilitation and was evaluated in clinic 6 weeks postoperatively without deficit. She remains asymptomatic 2 years from initial presentation.
30.3 Case Summary
30.3.1 Introduction
Spinal arteriovenous malformations (sAVMs) represent a rare and challenging neurosurgical entity characterized by abnormal connections between spinal arteries and veins, estimated to comprise approximately 4% of all intradural spinal cord lesions. 1 Despite their rarity, accurate and timely diagnosis is of tantamount importance as they are associated with a wide variety of morbidity, including radiculopathy, weakness, paresthesias, sensory deficits, loss of bowel and bladder function, and paraplegia. 2 More recent advances in radiographic technology have allowed for more accurate diagnoses via noninvasive modalities such as MRI and magnetic resonance angiography (MRA), but spinal angiography remains the gold standard for analysis of anatomical, architectural, and morphological features of the lesions.

30.3.2 History
In 1888, Gaupp first published his findings of what seems to be most consistent with a dural arteriovenous fistula (dAVF), characterizing the lesion as “hemorrhoids” of the pia mater. Shortly thereafter, in 1890, Berenbruch made similar observations of a lesion most consistent with a spinal vascular malformation. In 1911, Fedor Krause was first to describe visualization intraoper-atively of a spinal vascular malformation during a laminectomy, and Charles Elsberg became the first surgeon to document attempted resection of such a lesion, in 1916. Foix and Alajoua-nine in 1926 described their observations of rapid neurologic decline secondary to sAVM thrombosis, now recognized as subacute necrotic myelopathy or Foix-Alajouanine syndrome. 3
30.3.3 Incidence
While not as common as brain AVFs and AVMs, spinal AVFs account for 70% of all spinal arteriovenous shunts. Several classification systems have been devised based upon anatomical location, vascular features, genetics, and types of shunts involved (nidus vs. fistula) in an attempt to distinguish the subtypes of such lesions, the major systems of which are described in further detail below. 4 , 5 , 6 , 7 , 8 , 9 , 10 While such pathologies typically manifest independent of any syndromic correlate, spinal dAVFs and dAVMs have been linked with hereditary hemorrhagic telangiectasia or Osler-Weber-Rendu disease and associated genes HHT1 and HHT2.
30.3.4 Anatomy
In order to understand and appreciate both the pathophysiology of various manifestations of sAVMs, an understanding of spinal anatomy and its evolution is required. The work of Adamkiewicz in 1881 was the first comprehensive treatise on the subject of spinal cord vascularization. Significant further advances in spinal vascular anatomy came with the advent of spinal angiography and work by Doppman, Djindjian, and Laz-orthes. 11 Building on such work, the work of Lasjaunias et al further improved our understanding of anatomy of both normal spinal angioarchitecture and development as well as that central to pathologies such as spinal vascular malformations, allowing for modern day treatments in the form of microsurgical resection and endovascular obliteration. 12
From a basic level, the aorta derives from the heart and branches into segmental arteries, which further subdivide into spinal radicular and medullary arteries. The medullary artery subsequently bifurcates into anterior and posterior divisions, which then merge to form the anterior spinal artery coursing through the pia of the anterior median fissure and posterior spinal arteries coursing through the pia of the posterolateral sulci. The cervicothoracic spinal cord is supplied by a number of segmental vessels from the aorta as well as subclavian and both internal and external carotid arteries. The midthoracic spinal cord is supplied from segmental vessels deriving from the aorta, but due to lack of the lack of anastomotic redundancy that the cervicothoracic cord enjoys, stands at a higher risk of possible infarction. Separately, the thoracolumbar cord is supplied by segmental vessels from the abdominal aorta and iliac arteries.
While previous classification systems described spinal anatomical nomenclature based upon spinal artery location (i.e., posterior, anterior, and posterolateral distributions), the Lasjaunias classification sought to differentiate radicular arteries by regions of supply, including radiculopial, radiculomeduUary, and radicular. Via posterior radicular arteries, the radiculopial arteries supply the dorsolateral superficial pial system and nerve root. Via anterior radicular arteries, the radiculomeduUary arteries supply the anterior superficial pial system, nerve root, and the gray matter of the spinal cord. Spinal radicular arteries at every segmental level supply the neighboring nerve root and adjacent dura but not the spinal cord itself.
The anterior spinal artery, originating from bilateral vertebral arteries, travels along the anterior medial fissure with additional contributions from 2 to 14 (on average, 6) segmental radiculomeduUary arteries to supply the anterior two-thirds of the spinal cord. Of note, during embryological development, each radicular artery gives rise to its own respective radiculomedul-lary artery to supply the spinal cord, although the number of radicular arteries subsequently diminishes by postnatal life. This anterior circulatory supply gives rise to multiple sulcocom-missural arteries within the ventral sulcus to supply the central gray matter and portions of peripheral white matter. 13 The largest of the anterior radiculomeduUary arteries is the artery of Adamkiewicz (arteria radiculomedullaris magna), which arises close to the thoracolumbar enlargement, typically between T9 and LI. The posterior one-third of the spinal cord (i.e., the dorsolateral pial supply or centripetal system) relies upon circulation from the posterior spinal arteries, which derive origination from the posterior inferior cerebellar artery and intradural portion of the vertebral artery in addition to contributions from over a dozen radiculopial branches. 14 This network supplies radial arteries that circumferentially course along the spinal cord to anastomose with the ventral system, allowing for axially oriented perforating branches into white matter. Ultimately, both anterior and posterior spinal circulation anastomoses at the conus medullaris via two anastomotic semicircles known as the “arcade” of the cone. 15
The spinal venous system begins with radially oriented intrinsic veins and small superficial pial veins leading into superficial longitudinal medial spinal cord veins. Ultimately, these drain with significant transmedullary anastomoses into larger vein tributaries mimicking the arterial system (anterior and posterior medial spinal veins) which reach the extraspinal veins and epidural plexus (also known as Batson plexus). Anteriorly draining sulcal veins and posterior draining radial veins drain into the coronal venous plexus through the pia and through medullary veins into epidural veins, which drain into the dural sleeve of the dorsal nerve root. The spinal venous system is unique, in that it is a valveless system and, as such, does not prevent retrograde flow—a key consideration in fistula formation and pathophysiology as congestion throughout the entire circuit can manifest in myelopathy.
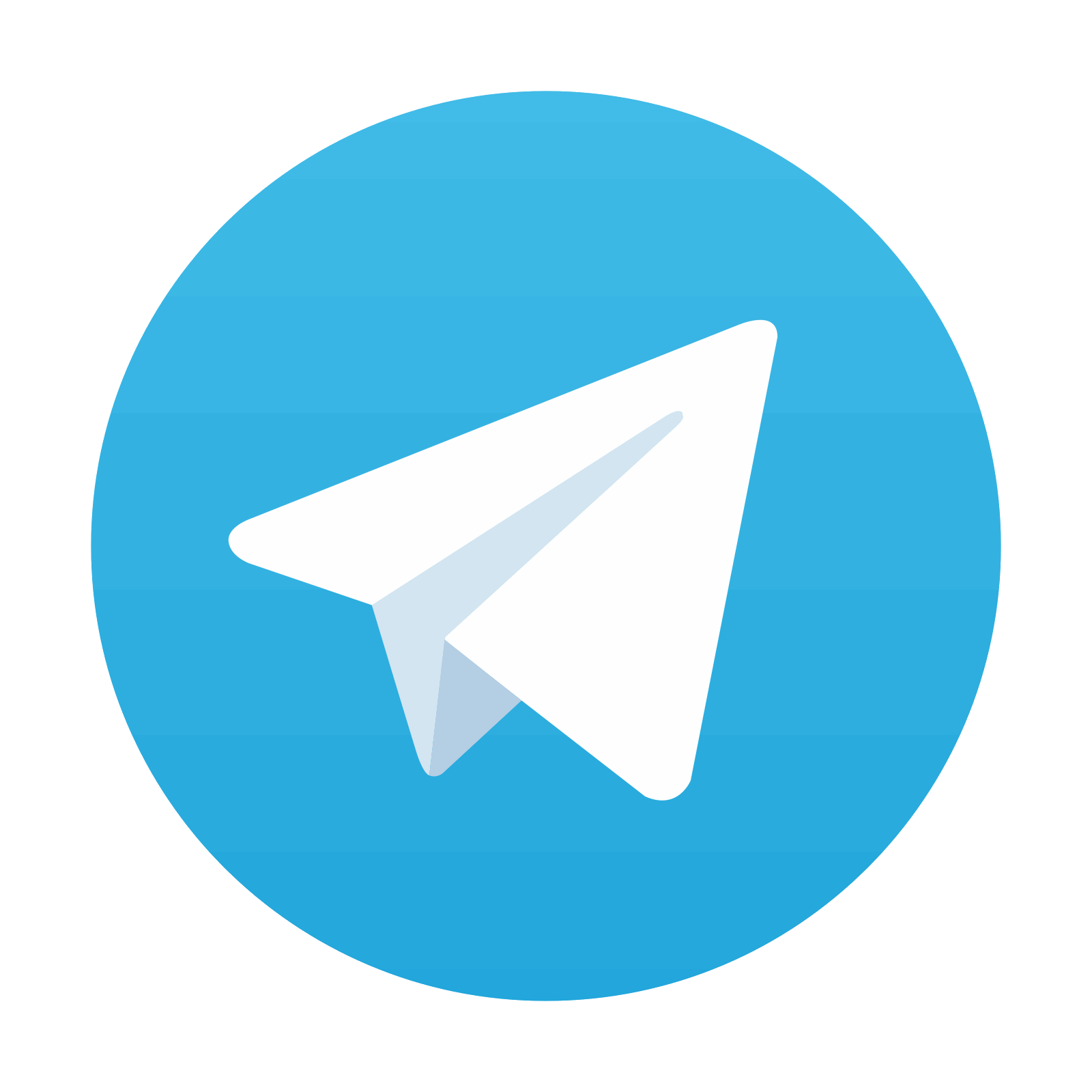
Stay updated, free articles. Join our Telegram channel

Full access? Get Clinical Tree
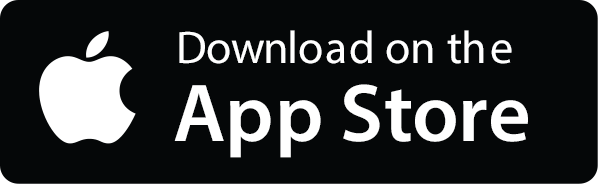
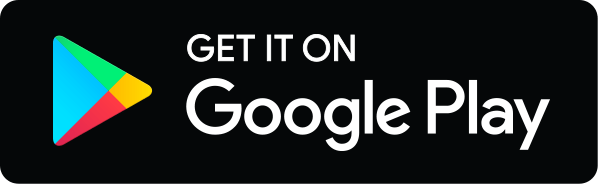
