32 Motion Preservation Technologies
A significant interest in spinal motion preservation technologies has been evident in the past decade. 1 – 6 However, conflicting opinions, and therefore controversy, have emerged in the past several years. 7 – 9 Surgical clinical trials are fraught with methodologic flaws and bias issues. These, along with the complexities associated with the performance of meaningful surgical clinical trials, have further complicated this domain of clinical medicine and spine care.
In order to better appreciate the clinical and research landscape, an in-depth look at what is known about the mechanics and clinical concerns associated with spinal motion preservation is undertaken in this chapter. The variety of motion preservation options are first presented.
32.1 Strategies for Motion Preservation
32.1.1 Avoidance of Surgery
The avoidance of surgery (or other interventions) should be considered the ultimate motion preservation strategy. This may often be the most prudent strategy. The avoidance of surgery, unfortunately, is often not the chosen option during the clinical decision-making process. Nearly all invasive interventions either further destabilize or augment stability. In both cases, spinal motion is affected—and almost always in an adverse manner. Fusion diminishes or eliminates motion, and decompression operations or interventions can decrease stability and, hence, increase motion—in particular, pathologic motion. So, perhaps the very best motion preservation strategy is indeed the avoidance of surgery.
In this regard, recent clinical trials have demonstrated the significant efficacy of nonoperative means for the treatment of neurogenic claudication related to lumbar stenosis. 10 , 11 Membrane stabilizers (i.e., gabapentin) were shown to be clinically effective in 60% of symptomatic patients with neurogenic claudication related to lumbar stenosis. The improvements were substantial and sustained. This is illustrative of a minimally invasive approach to a problem whose treatment was previously considered to be solely within the surgical domain.
32.1.2 Neural Element Decompression
Neural element decompression without fusion permits the retention of mobility without the creation of significant destabilization in the appropriately selected patient. The prototypical surgical procedure in this regard is the cervical laminoforaminotomy for cervical disc herniation. 12 The “gold standard” alternative procedure is an anterior cervical discectomy and fusion. The latter involves fusion, whereas the former involves a lesser intervention for decompression and resection of the offending mass.
Laminoplasty has been touted as a gold standard for motion-sparing and stability-sustaining technology. Much has been published regarding the attributes and deficits associated with laminoplasty 13 – 26 and variants. 27 – 30 Although most studies are positive regarding the virtues of laminoplasty, convincing challenges to its efficacy are also eloquently presented in the literature. 13 , 21
32.1.3 Interspinous Spacers
Interspinous spacers have been touted as a strategy to decompress, in a minimally invasive manner, or rather to expand the lumbar neuroforamina, thus managing lumbar stenosis and symptoms of neurogenic claudication in a minimally or less invasive manner than provided by traditional surgical decompression strategies (e.g., lumbar laminectomy and foraminotomy). 31 – 38 Unfortunately, this strategy is obligatorily kyphosing. At the very least, it limits normal spine extension.
32.1.4 Dynamic Spine Stabilization
Dynamic spine stabilization techniques have been employed to “stiffen” the spine in patients with allegedly mechanically unstable or deranged and painful motion segments. 39 – 41 Clinical and imaging improvement has been reported. 42 Although dynamic fixators do indeed stiffen the spine, they have not been shown to be effective for managing mechanical back pain. 43 The mechanics associated with such devices have been critically studied. 44 , 45 They have also been employed to enhance fusion, but also without proof of efficacy.
32.1.5 Nuclear Replacement Devices
Nuclear replacement devices have been developed and employed in a limited manner. They are designed to replace, anatomically and functionally, the nucleus pulposus, leaving the annulus fibrosus for containment and stability purposes. 46 – 50 Although theoretically sound, a demonstration of clinical utility is as of yet lacking. Nucleoplasty has also been used to alter existing nuclear material and to diminish pain. This has been preliminarily studied. 51
32.1.6 Total Disc Arthroplasty
Total disc arthroplasty (TDA) is employed to replace, both anatomically and functionally, the intervertebral disc interspace. The literature is mixed regarding clinical benefit. Nevertheless, because it represents the gold standard for motion preservation device technologies, the discussion in this chapter focuses primarily on TDA.
32.2 Biomechanics of Motion Preservation Technologies
The biomechanics of motion preservation technologies, in particular TDA, are infrequently considered from a holistic perspective. However, it is imperative, when new technologies are applied, to consider the mechanisms of action of the technology at hand. It is also imperative to understand the fundamental physical principles that provide the foundation for clinical use. Hence, it is relevant to study the attributes of TDA and other motion preservation strategies. 55 TDA design characteristics influence the intervertebral kinematic parameters that affect performance and wear and tear. 56
32.2.1 Attributes of Total Disc Arthroplasty
Many factors contribute to the overall functional makeup of TDAs. These must be aligned with the goals of surgery. 57 The predominant attributes of TDA (the replication of anatomy, motion, mechanics, and the instantaneous axis of rotation [IAR]; the incidence of complications; the adequacy of revision strategies in failed cases, the longevity of the implant; the ability of arthroplasty to retard degenerative changes; and the symptom relief associated with arthroplasty) are, from a clinical and structural perspective, most critical.
Replication of Anatomy
A TDA should, in a structural sense, replicate normal disc interspace anatomy (disc interspace height and angle). In general, most currently available TDAs meet this criterion; therefore, the anatomical criterion for most TDAs is appropriately met.
Artificial joints in regions other than the spine (e.g., hip and knee artificial joints) are “ball-in-socket” joints. The ball-in-socket joint is conceptually similar to the synovial (diarthrodial) joint. This type of joint, however, varies significantly from the intervertebral joint, the latter being an amphiarthrodial joint. In its youthful state, the amphiarthrodial joint is composed of a gel-like material (i.e., the nucleus pulposus) that is filled and tightly contained by the annulus fibrosus. First-generation TDAs essentially function as ball-in-socket joints. The application of such a mechanical strategy (ball-in-socket joint) to a clinical environment in which motion is allowed and constrained by a diarthrodial joint poses some significant theoretical and real clinical challenges and presents innumerable partial and nearly complete barriers to true success. 55
Replication of Motion
A TDA should, to one degree or another, replicate the motion of the normal intervertebral disc. This replication of motion should be both quantitative and qualitative. The center of rotation of currently available TDAs usually deviates from the norm. And although flexion–extension and lateral bending may quantitatively approach the norm (i.e., the extent or range of motion), 58 – 62 qualitative parameters may or may not. 63 Spinopelvic alignment and range of motion have been shown to be improved with lumbar TDA. 64 Motion, in addition, may be lost over time. 63 These parameters include coupled motions and the coordinated and orchestrated sequencing of component motions that make up a fluid movement. These often significantly deviate from the norm. Finally, all first-generation TDAs do not allow axial motion, being either metal on metal or metal on polymer, or the equivalent. These do not permit “cushioning” of the motion segment with axial loading. Hence, axial loads are transmitted through the disc to adjacent segments and to the index-level facet joints.
Replication of Mechanics
The replication of the mechanics of a motion segment essentially revolves around the ability of the TDA to mimic stiffness in all planes. Stiffness is a function of both applied stress (applied load) and resultant strain (motion), defined by the following equation:
For biological tissues, the stress/strain curve has two fundamental components: a neutral zone and an elastic zone. If loaded to a point nearing failure, a plastic zone is observed, and finally failure occurs (Fig. 32.1). Stiffness is determined by calculating the slope of the elastic zone of the stress–strain curve.

Stiffness has been arbitrarily subclassified into several categories. One such classification scheme compartmentalizes stiffness into three categories: unconstrained, semiconstrained, and constrained. Unfortunately, each subtype is not clearly defined and is often used differently by various authors, researchers, and manufacturers/vendors. For example, a first-generation TDA may be considered a semiconstrained device by some, whereas others consider it to be an unconstrained device. Hence, for the purposes of discussion here, the term unconstrained is mechanically portrayed by the neutral zone portion of the stress/strain curve, and the term semiconstrained defines the characteristics associated with the elastic zone of the stress/strain curve. Constrained (or fully constrained) implies no motion and infinite or nearly infinite stiffness, such as might be seen with a solid fusion or rigid instrumentation. A fully constrained construct is depicted mechanically by a vertical line on the stress/strain curve (Fig. 32.2). This would be characteristic of a solid fusion. 55

The biomechanical correlate of a degenerated disc whose motion and mechanics are dysfunctional is portrayed by a shift to the right of the stress/strain curve (Fig. 32.3). Thus, the neutral zone is widened and the elastic zone is shifted to the right. A “sloppiness,” if you will, of segmental motion is observed. This represents an expansion of the zone of disengagement (neutral zone). Because of the increase in laxity associated with a dysfunctional motion segment and a widened neutral zone, a pain syndrome may result. This is classically mechanical in nature and is termed mechanical pain. Mechanical pain is characterized by a clinical triad: it is deep in location, agonizing in nature, and worsened with spinal loading and improved with unloading. The term zone of disengagement is used here to depict a zone in which very little force is required to cause motion or displacement. This is a zone where relative disengagement between components of the system occurs. It is in this zone (neutral zone) that the aforementioned sloppiness of motion exists.

The various spinal motion–altering devices can be categorized and quantitatively assessed by noting their stress/strain characteristics. Such an assessment should evaluate flexion–extension, lateral bending, and axial loading. With each (flexion–extension, lateral bending, and axial loading), a stress/strain curve, as depicted in Fig. 32.1, can be generated. In flexion–extension and lateral bending, the first-generation TDA ball-in-socket–like joint is associated with a widened neutral zone until a hard stop is reached at the extreme of motion (Fig. 32.4a). This is strikingly different from the mechanics observed in vivo in a normal or nearly normal disc.

An elastomeric disc, in which elastomer is sandwiched between the end plate caps, as in the short-lived AcroFlex (DePuy-AcroMed, Raynham, MA) and to some degree the new second-generation discs, may have the mechanical characteristics depicted in Fig. 32.4b. This approximates the normal stress/strain relationships in flexion–extension and lateral bending more closely than does the ball-in-socket–like joint.
A dynamic stabilization device, such as the Dynesys implant (Zimmer, Warsaw, IN), stiffens the spine and may be expected to shrink or normalize a pathologically widened neutral zone (Fig. 32.4c), whereas a fusion, as already noted, is associated with nearly infinite stiffness and is portrayed mechanically as a nearly vertical and straight “curve” (see Fig. 32.2). 55
Implant loading mechanics are quite different for axial loading. Metal-on-metal or metal-on-polymer implants do not effectively cushion loads. Hence, they are nearly infinitely stiff in axial loading, as depicted for fusion in Fig. 32.2. When compared with one another regarding axial stiffness and shock absorption, such devices perform similarly. 65 Elastomeric implants, on the other hand, cushion loads and have the mechanical characteristics depicted in Fig. 32.4b. They protect the dorsal elements from shear loading and more accurately reproduce normal intervertebral disc mechanics. 66 , 67 Depending on the stiffness and unique physical attributes of a dynamic spine stabilization implant (see Fig. 32.4c), the slope of the elastic zone is likely to be located between that of the metal-on-metal or metal-on-polymer implant (see Fig. 32.4a) and that of the normal spine in vivo (see Fig. 32.1). 55
As already stated, the nearly infinite stiffness in axial loading associated with first-generation TDAs causes axial loads to be directly transferred to adjacent levels and to the facet joint complex at the surgical index level. This may cause significant wear and tear over time.
Finally, it must be recognized that not all spines are alike. Stiffness varies with age and from patient to patient. A youthful disc is well hydrated and is composed of a gelatinous nucleus pulposus and a tough, constraining, and functional annulus fibrosus. Pressures are uniformly high across the entirety of the disc in loaded conditions (Fig. 32.5a). The internal pressure in the loaded disc begins to drop with age, and most of a load is borne by the perimeter of the disc in the region of the degenerated annulus fibrosus, which has gradually become a fibrocartilaginous scar (Fig. 32.5b, c). In extreme cases, the intradiscal pressure can temporarily fall below zero, resulting in the appearance of intradiscal gas on plain radiography (Fig. 32.5d and e).

In a way, TDAs are designed to mimic the youthful disc. Unfortunately, they fall short of this “goal” from a mechanical perspective. Intervertebral disc mechanics and mechanical relationships change with time. Loading patterns degrade over time (see Fig. 32.5). The inability of a TDA to change with time, as an aging intervertebral disc can, further muddies the waters regarding the quest for the perfect motion preservation implant.
Replication of the Instantaneous Axis of Rotation
The location of the IAR of the intervertebral motion segment changes under differing loading conditions and loads. It is normally maintained in the region of the disc interspace. Some TDA implants have a fixed IAR, whereas others may have a variable IAR. None of the currently available implants replicate the normal or natural IAR motion characteristics. The clinical implications of this are not yet fully known but have been studied. 68 , 69 However, it is likely that a fixed IAR will prove to be detrimental to motion segment mechanics.
Complications
Although the initial experiences with TDA application yielded reports of significant complications, including fatalities associated with dislodgement, improvements in implant design and surgical technique have minimized both the incidence and significance of complications. 70 – 73 Van Ooij et al observed substantial complications associated with the lumbar SB Charité disc (DePuy-Acromed, Raynham, MA). 74 Most complications were related to facet joint pathology at the same level, degeneration of the facet joints and discs at adjacent levels, and subsidence and migration of the prosthesis.
Soft bone and suboptimal geometry and footprint size and shape were associated with implant subsidence, 73 , 75 – 77 but advanced age (older than 45 years) was not an absolute contraindication for TDA. 78 Material failure was also observed. Investigators argue that the predominant reason for such complications is related to mechanical design issues. Many of these biomechanical issues were raised in prior paragraphs in this chapter. Of relative note here is that it appears that less retraction of the esophagus is required to insert a cervical TDA than a fusion with plate. 79
Heterotopic ossification has been problematic, particularly with the Bryan cervical disc (Medtronic, Minneapolis, MN). 80 – 85 This is likely related, at least in part, to the requisite bone milling involved with insertion. However, the same complication has been observed with prostheses that do not require extensive bone milling at the time of insertion. 86 Other complications that are device-specific include sagittal vertebral body split fractures related to the use of a keeled prosthesis. 87 – 89 Finally, failure at the implant–end plate junction can occur because of poor bone quality. Zhang et al, using peripheral quantitative computed tomography, observed a significant correlation between end plate failure stress and bone mineral content. 90
The generation of wear debris, with a subsequent inflammatory response, has been of concern. This has been observed with total hip and knee prostheses and in the TDA arena, as well. 91 Conflicting reports have been generated, 92 though. Of note, wear debris has been observed as early as 12 weeks after insertion in a postmortem case. 93
Kyphotic deformation has also been observed following the placement of a TDA. This may be related to surgical technique and/or device-specific issues. 94 , 95 In the lumbar spine, angular mismatch between the implant and the end plate can affect outcome. Such mismatch is associated with a reduced segmental range of motion. 96 It is most common at L5–S1.
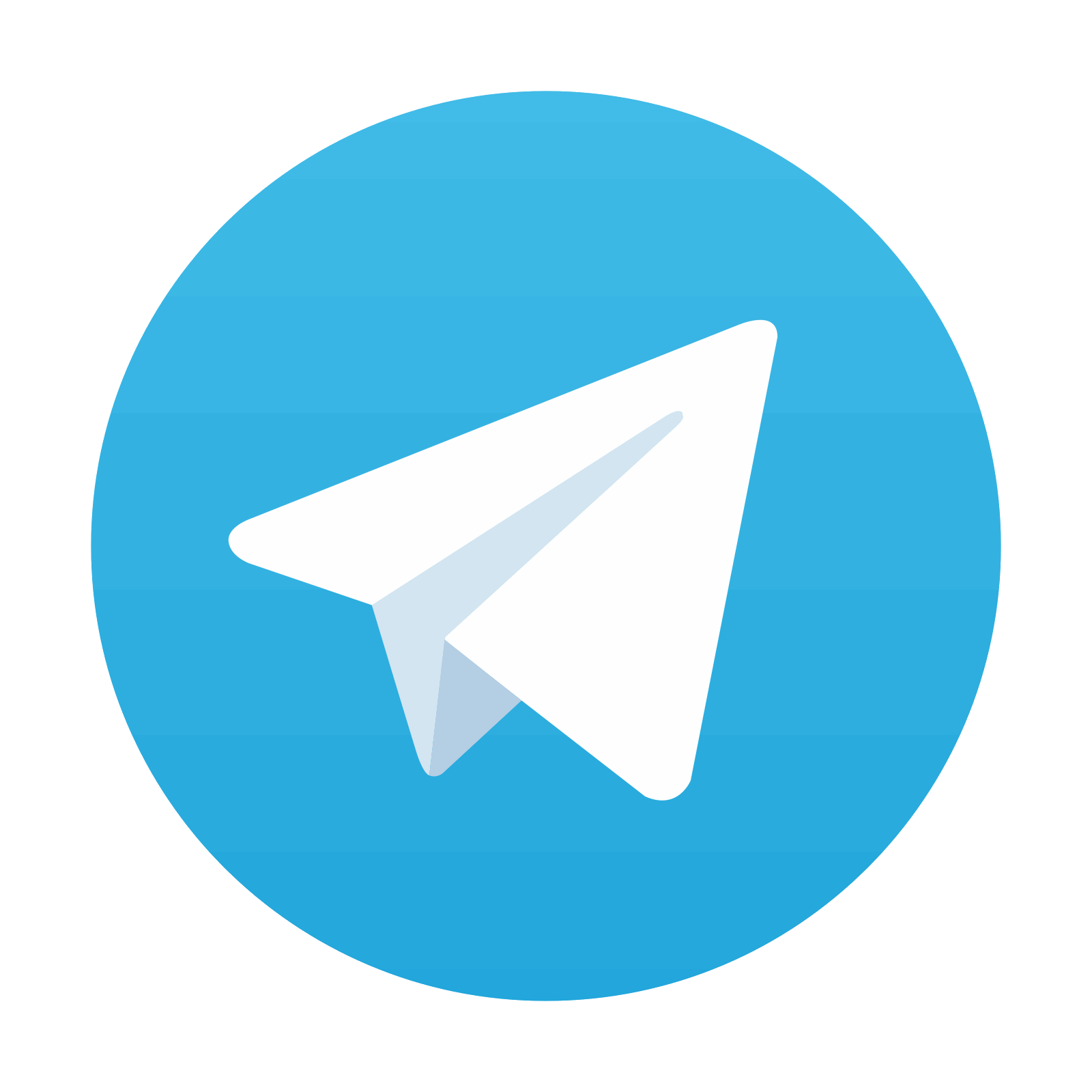
Stay updated, free articles. Join our Telegram channel

Full access? Get Clinical Tree
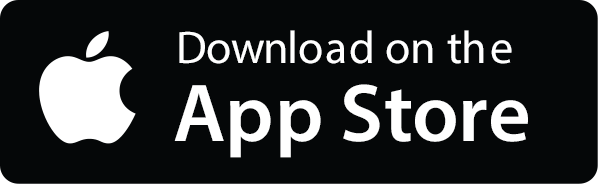
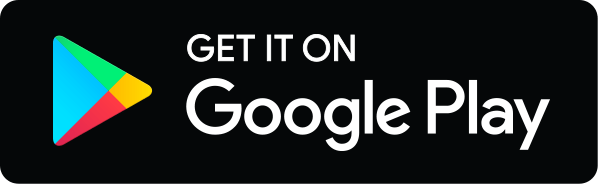
