36 Spinal Bracing
The achievement of spinal stability is optimized by (1) appropriate and not excessive decompression, (2) appropriate bone graft or spacer placement (fusion), (3) appropriately placed internal fixation constructs, and (4) the appropriate use of external splinting techniques. The latter may not be necessary or may include such strategies as bed rest, traction, and spine bracing. The latter is the focus of this chapter. General references regarding orthotics and bracing are available elsewhere. 1 – 3
Both bed rest and traction (see Chapter 35) and spine bracing are fraught with difficulties. The duration of bed rest required to attain adequate bony healing is considerable. Furthermore, lengthy bed rest is not without risk. Associated complications include, but are not limited to, deep vein thrombophlebitis, pulmonary embolus, pneumonia, decubitus, joint contractures, and depression.
Spine bracing presents its own unique set of associated problems, the most significant of which is its relative lack of effectiveness in achieving its goal (i.e., to minimize excessive spinal movement). The amount of soft tissue separating the spine and the brace itself minimizes the effectiveness of spinal bracing. In fact, there is an inverse relationship between the thickness of the soft tissue between the spine and inner surface of the brace and the effectiveness of the brace.
A longer brace usually provides more spinal stability than a shorter one. Therefore, the length-to-width ratio of the brace plays a significant role with respect to the efficacy of stabilization (Fig. 36.1).

The goals of spinal bracing include (1) restriction of movement, (2) achievement and maintenance of spinal realignment, and (3) trunk support. The achievement of these goals and the mechanisms by which they are achieved are of great importance. The surgeon planning to use a spinal brace must understand the patient-specific and clinical scenario–specific goals of bracing and must have the ability to realistically appraise, within reason, the properties of individual orthoses.
Various external splinting techniques have been used to facilitate the early ambulation of the patient with an unstable spine. Spinal splints are made from a variety of materials. The splints generally are constructed of materials that furnish some minimal flexibility and simultaneously provide adequate structural support. Plastic polymer brace materials have several advantages: diminished weight, the use of ventilation holes to provide increased comfort, relative ease in the doffing and donning of the brace, and ease of fabrication. 1 , 4 Splinting devices that do not closely conform to the torso (e.g., the halo brace and the Jewett brace) have several disadvantages. The Jewett brace, for example, applies a dorsally directed force at the sternum and the pubic region and a ventrally directed force at the thoracolumbar junction. This latter force is often applied at the level of painful pathology (e.g., site of a gibbus following a thoracolumbar wedge compression fracture). Because the pressure applied may be significant, it frequently causes discomfort. In the case of lumbar instability, it does not place the ventrally directed force in an appropriate location (i.e., in the low lumbar or lumbosacral spine). Furthermore, the Jewett brace and similar techniques do not promote maintenance of the cylindrical body shell. Contact with the torso is made over a relatively small surface area (Fig. 36.2). The concept of the body shell has been previously addressed from several viewpoints. 5 – 8 The Jewett brace, however, does provide a three-point bending biomechanical advantage (Fig. 36.3). 3 , 7 This has been shown to be an important factor in the stability achieved with external splinting. Maintenance of the body shell also increases the stability of the ventral and dorsal spinal elements (columns). Morris and Lucas have shown and illustrated the significant role of the trunk (maintenance of the body shell) as a stabilizer of the spine. 5 Finally, the Jewett and similar braces do not significantly restrict lateral bending.


The conformation (close fit) between the ventral and dorsal halves of a brace are critical to the ability of the brace to stabilize the spine. A suboptimal fit between the halves of a brace allows a parallelogram deformation of the brace itself. This in turn diminishes its desirable biomechanical effects and its ability to protect spinal column integrity. The halves of the brace should not only be secured so that one does not slide past the other but should also be rigidly attached to each other (Fig. 36.4).

36.1 Cervical Spine Bracing
The cervical spine is perhaps the region of the spine that is most effectively stabilized by external splinting techniques. This is related to the smaller amount of soft tissue separating the brace from the spine itself. It is also associated with the substantial points of fixation available at the rostral and caudal termini of the cervical region: the cranium and the thoracic cage.
Difficulties associated with the prevention of rotation and bending in all directions is variably associated with all techniques. 9 – 15 The extent of lateral bending is difficult to assess—the surgeon must rely on anteroposterior radiographs. These are inherently more difficult to assess than equivalent lateral radiographs. Rotation is even more difficult to assess. Johnson and colleagues and Maiman and colleagues used a goniometer scheme to assess rotation following cervical bracing. 14 , 16 Lateral and rotatory movements, however, usually are less important than sagittal plane movements with regard to clinical stability concerns. Cervical collars are associated with variable efficacy in all the aforementioned regards. 17 – 20 Of note, rigid cervical collars can have unexpected negative effects. They have been shown to adversely alter automobile driver performance by restricting neck movement. 21
The parallelogram-like bracing effect is a unique characteristic of the cervical spine. It is related both to the significant mobility of the cervical spine and to the lack of adequate fixation points in the mid-to-low cervical region. The extensive mobility of the upper cervical (atlanto-occipital) and middle and lower cervical regions combines the unique characteristics of both capital and true neck flexion and extension movements to exaggerate the parallelogram-like bracing effect (Fig. 36.5).

Bracing that attempts fixation from the mandibular region to the base of the neck and shoulder often does not effectively prevent parallelogram-like movements (Fig. 36.6). In fact, this method of bracing may encourage it. A review of published data on cervical orthotic effectiveness illustrates this point (Table 36.1 and Table 36.2). 14 Devices using mandible fixation points without thoracic fixation points permit excessive movement at each motion segment. The prevention of lower cervical spine movement via the attainment of solid points of thoracic fixation appears to provide a significant reduction of segmental movement at all cervical spine levels. This may pertain to upper thoracic spine segmental movement, as well. The combination of capital and compensatory true neck movements (or vice versa) can be controlled by limiting one or the other type of movement because they are compensatory. Because capital (upper) cervical movement is indeed difficult to restrict, the most effective alternative is the minimization of true (mid-to-low) cervical neck movement. This can be accomplished with the use of solid points of thoracic fixation (Fig. 36.7).
Test situation | Motion | O–C1 | C1–C2 | C2–C3 | C3–C4 | C4–C5 | C5–C6 | C6–C7 | C7–T1 |
Normal unrestricted | Flexion | 0.7 ± 0.5 | 7.7 ± 1.2 | 7.2 ± 0.9 | 9.8 ± 1.0 | 10.3 ± 1.0 | 11.4 ± 1.0 | 12.5 ± 1.0 | 9.0 ± 1.1 |
Extension | 18.1 ± 2.1 | 6.0 ± 1.2 | 4.8 ± 0.8 | 7.8 ± 1.1 | 9.8 ± 1.2 | 10.5 ± 1.3 | 8.2 ± 1.2 | 2.7 ± 0.7 | |
Soft collar | Flexion | 1.3 ± 1.3 | 5.1 ± 1.9 | 4.5 ± 1.2 | 7.4 ± 1.5 | 8.4 ± 2.4 | 9.9 ± 1.7 | 9.7 ± 0.9 | 7.7 ± 2.5 |
Extension | 13.7 ± 3.5 | 1.9 ± 1.4 | 3.9 ± 1.0 | 5.8 ± 1.7 | 6.8 ± 1.6 | 7.8 ± 1.2 | 7.4 ± 1.4 | 2.8 ± 1.9 | |
Philadelphia collar | Flexion | 0.9 ± 1.0 | 4.0 ± 1.8 | 1.6 ± 1.0 | 3.1 ± 1.1 | 4.6 ± 1.8 | 6.2 ± 1.9 | 6.2 ± 1.6 | 5.5 ± 1.8 |
Extension | 6.8 ± 2.2 | 4.5 ± 1.5 | 1.8 ± 0.9 | 3.4 ± 1.0 | 5.8 ± 1.2 | 5.9 ± 1.2 | 5.8 ± 2.0 | 1.3 ± 0.9 | |
SOMI brace | Flexion | 3.6 ± 1.8 | 2.7 ± 1.8 | 0.9 ± 0.7 | 1.6 ± 1.1 | 1.9 ± 0.8 | 2.8 ± 1.2 | 2.9 ± 1.6 | 3.1 ± 1.8 |
Extension | 9.1 ± 2.6 | 5.4 ± 1.9 | 4.4 ± 1.1 | 6.3 ± 1.4 | 6.0 ± 1.8 | 6.0 ± 2.0 | 5.6 ± 1.8 | 2.1 ± 1.1 | |
Four-poster brace | Flexion | 2.9 ± 2.0 | 4.4 ± 2.1 | 1.6 ± 1.0 | 2.1 ± 1.1 | 1.8 ± 0.9 | 3.0 ± 1.2 | 3.9 ± 1.6 | 2.8 ± 1.4 |
Extension | 9.3 ± 2.2 | 3.2 ± 1.4 | 2.0 ± 0.7 | 3.2 ± 1.2 | 3.4 ± 1.3 | 2.9 ± 0.9 | 3.1 ± 1.5 | 1.6 ± 0.8 | |
Cervicothoracic brace | Flexion | 1.3 ± 0.9 | 5.0 ± 1.9 | 1.8 ± 0.8 | 2.9 ± 1.2 | 2.8 ± 0.7 | 1.6 ± 0.8 | 0.7 ± 0.6 | 2.4 ± 1.0 |
Extension | 8.4 ± 2.1 | 2.5 ± 0.8 | 2.1 ± 0.7 | 1.6 ± 0.7 | 2.2 ± 0.9 | 2.8 ± 0.9 | 3.4 ± 1.1 | 1.7 ± 0.8 | |
Abbreviations: O, occiput; SOMI, sternal occipital mandibular immobilizer. Note: Data are expressed in degrees as mean and 95% confidence limits of the mean. |
Stabilization device | O–C1 | C1–C2 | C2–C3 | C3–C4 | C4–C5 | C5–C6 | C6– C7 | Sum of angles | Average movement at each | Sum of angles to C6 or C7 | Measured movement |
Halo jacket | 4.5 ± 2.7 | 1.3 ± 1.1 | 4.1 ± 2.6 | 4.1 ± 3.2 | 3.1 ± 2.6 | 3.0 ± 1.9 | 6.3 ± 5.7 | 23.4 ± 13.7 | 3.7 ± 3.1 | 23.4 ± 13.7 | 5.2 |
Minerva jacket | 3.5 ± 2.1 | 2.1 ± 1.1 | 1.7 ± 1.7 | 1.9 ± 1.2 | 2.0 ± 2.1 | 2.5 ± 1.6 | 2.3 ± 1.8 | 14.8 ± 4.4 | 2.3 ± 1.7 | 14.8 ± 4.4 | 5.2 |
Source: From Benzel et al. 10 Abbreviation: O, occiput. Note: Data are expressed in degrees as mean ± standard deviation. O = occiput; * = statistically significant difference (p < 0.025) aStatistically significant difference (p < 0.025). |


Another problem associated with cervical spine fixation is that of snaking. This phenomenon, which occurs most commonly when rigid fixation is used, 13 , 22 , 23 is defined as a serpentine movement of the spine in which a simple overall movement (e.g., flexion or extension) is accompanied by a combination of flexion and extension movements at each intervertebral level. 10 , 14 Although overall movement from the head to the thorax may be minimal, the cumulative segmental movement between these points may be substantial. Therefore, snaking can be defined quantitatively as the difference between the sum of all segmental movements (the sum of the absolute values of the segmental motions) between the head and the thorax and the overall movement between the head and the thorax. 10 Although segmental movements of the spine cannot be fairly and objectively assessed, on account of inconsistent responses of the patient (see the following), the difference between the observed overall movement and the sum of segmental movements can be measured. This then becomes a relatively objective assessment that relies only on each subject’s own control. 10
The means used to assess the efficacy of cervical bracing techniques are somewhat artificial. Therefore, the surgeon must be careful not to rely too heavily on the published data. The movement measured in most studies is elicited voluntary neck movement, the extent of which depends on the cooperativeness of the braced patient. Furthermore, and much more significantly, such movement depends on a consistent submaximal attempt at flexion, extension, rotation, or lateral bending. True consistency is nearly impossible to attain, and more importantly, it is nearly impossible to quantify. These factors are especially important in the cervical spine, but they also play a role in the thoracic and lumbar spine.
The available data on the attributes and faults of individual external cervical spine splinting techniques are discussed in the following text. The techniques are grouped to facilitate the objective assessment of each technique. These groups are (1) limited cervical bracing techniques, (2) cervical–shoulder bracing techniques, (3) cervical–thoracic bracing techniques, and (4) cranial–thoracic bracing techniques.
36.1.1 Limited Cervical Bracing Techniques
Limited cervical braces have no neck base or shoulder fixation points. These points of fixation are important for the restriction of cervical motion. All of these techniques offer, to one degree or another, a mandibular point of fixation. They vary, however, in their ability to affix to the base of the neck, to the shoulder, or to the thoracic region. Collars that do not affix to the base of the neck, shoulder, or thorax region are the least effective (Fig. 36.8a). They include the soft cervical semirigid collars. Flexion–extension movement is essentially unrestricted by these cervical collars (see Table 36.1). Because these devices do not substantially restrict movement in any direction, they do not enhance the parallelogram-like bracing effect. Their overall ineffectiveness in restricting cervical movement, however, makes this a moot point.

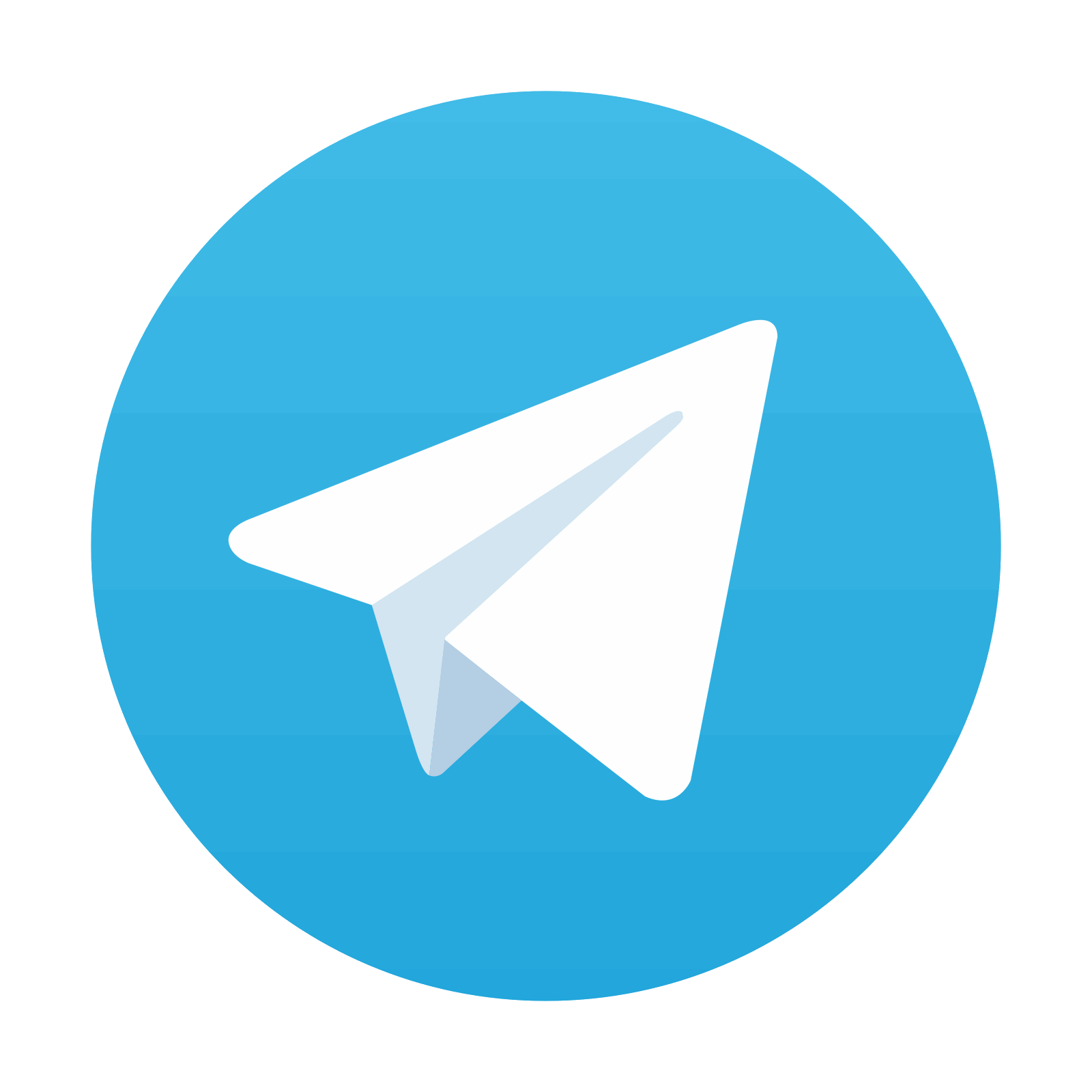
Stay updated, free articles. Join our Telegram channel

Full access? Get Clinical Tree
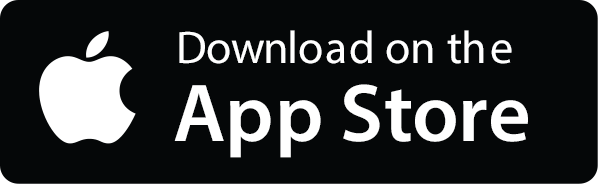
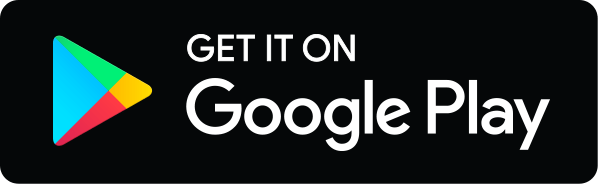
