38 Biomechanical Testing
Up to this point in the book, armchair biomechanics have in a sense been applied to the clinical arena—that is, physical, kinematic, and biomechanical principles have been applied in a logical manner to clinical problems. Intuition plays a role in this process. All surgeons conceive patient-specific clinical strategies that are based, at least in part, on intuition. They also use the principles outlined in this book and their individual prior experiences.
A large portion of our knowledge base is derived from biomechanical studies. It is emphasized, however, that the performance, documentation, and interpretation of biomechanical studies are prone to error and even misinterpretation. This chapter specifically addresses such issues: the performance, documentation, and interpretation of spinal biomechanical research.
The performance or assessment of a biomechanical study involves critical appraisal of the methodology used, as well as a knowledge or awareness of the pitfalls of biomechanical testing and interpretation. 1 , 2 Panjabi set the standards decades ago. 3 This chapter addresses the factors and pitfalls associated with biomechanical testing.
38.1 Methodologies
A variety of methodological approaches to the study of biomechanical spinal column behavior can be employed. Many variables play a role in the decision-making process regarding the planning, performance, and interpretation of a biomechanical study. These are discussed in the pages that follow.
38.1.1 Determination of the Experimental Strategy
The question(s) to be answered by a study must first be determined. Is the spinal column, a spinal implant, or the implant–bone interface to be studied? Is information about stiffness, flexibility, strength, failure, or fatigue desired? The experiment must be tailored to answer the question at hand. Care must be taken to avoid using a strategy that will not answer the intended question. For example, traditional multiple-cycle (millions of cycles) fatigue testing to assess the long-term integrity of the bone–metal junction of a construct provides limited information. Stress-to-failure testing may similarly not be appropriate for the assessment of a screw–bone interface in osteoporotic bone. In fact, short-term (fewer than 100,000 cycles) fatigue testing may provide more clinically relevant information. A cadaver spine obviously is not living tissue and therefore cannot physiologically respond to the stresses placed upon it (e.g., remodel). Hence, the experimental bone–metal interface will degrade more rapidly than it would in an in vivo situation. Hence, if cyclical testing is to be performed, it must be accomplished before the specimen decomposes and in a biomechanical time frame during which remodeling would not occur if this were an in vivo experiment (i.e., fewer than 100,000 cycles). On the other hand, cyclical loading (fatigue testing) may indeed be appropriate for the study of implant integrity and efficacy. The average spine is exposed to 1 million to 3 million cycles per year. 4 The average spine implant is generally expected to maintain stability until fusion is achieved. This, for the sake of argument, is generally about 6 months. Therefore, the implant should be able to withstand approximately 1 million cycles of “normal” load magnitude, although it is customary to test to 5 million or 10 million cycles or more. This testing can be by the application of such physiologic loads as polyurethane blocks to which the implant is secured (Fig. 38.1a).

Significant limitations of laboratory testing, however, exist. The aforementioned strategy tests only the implant. Cadaver specimens do not have surrounding attached functional musculature and soft tissues (Fig. 38.1b). Therefore, they do not effectively mimic or replicate the in vivo situation. Furthermore, there exists significant specimen variability. This obligatorily increases the sample size required to achieve statistical significance and thus cost. Animal specimens provide greater uniformity regarding geometry and bone density. They are therefore superior to cadavers for assessing instrumentation performance, but not for assessing kinematics related to the human spine.
38.1.2 Choice of Specimens
Human cadavers are a good model for biomechanical testing. They generally replicate the clinical situation. However, they are expensive, handling is complicated by the nearly insignificant but finite risk of infection, and they are often not representative of the situation to be studied. For example, the specimens are often aged. This poses significant challenges and problems if a researcher intends to study spine trauma, which most commonly afflicts adolescents and young adults. If young human spines are unavailable or impractical, and if bone quality is an important factor in the planned study (e.g., a study to assess the probable in vivo efficacy of an implant), animal spines that are anatomically similar to human spines and of similar size, such as calf or sheep spines, may be more appropriate than human specimens. Regardless, such specimens should be fresh and free of any embalming or chemical treatments.
38.1.3 Specimen Preparation
Appropriate techniques for specimen preparation are an integral part of the mechanical testing process. Creep, temperature, and specimen hydration are particularly important considerations. Misleading information may be accrued if these and other factors are not appropriately accounted for (see the following).
In general, spine specimens are cleared of nonstructural soft tissue. The spinal ligaments and discs are usually left intact. Specimens are often frozen until testing. Therefore, they must be thawed completely before testing and should be kept moist with saline-soaked wrappings.
38.1.4 Specimen Mounting
The specimen must be affixed to the testing machine at both ends. Specialized machines that grip the fixtures housing the specimens are designed to fit into the materials-testing machine and can be customized to apply offset loads to the specimen. Potting is the process of embedding the specimen into the gripping fixture with plaster of Paris, polymethylmethacrylate, or a polyester resin. Plaster of Paris is brittle, so it is a less desirable choice in most circumstances. Fixation security may be enhanced with screws or bolts. The vertebrae rostral and caudal to the motion segment(s) or vertebrae to be tested should be potted (affixed to the biomechanical testing machine). The specimen should be centered in the fixture (pot), or untoward bending moments will be introduced (Fig. 38.2). Often, specimens are potted while frozen to avoid misalignment in the pot caused by the flexibility of the thawed spine (Fig. 38.3). If the aforementioned strategy is employed, the specimen is thawed after potting, but before testing.


38.1.5 Specimen Length
The number of motion segments included in the specimen can significantly impact the results. 1 Snaking can occur if multiple motion segments are included. This effect is exaggerated if the normal spine posture is altered (e.g., loss of normal cervical lordosis; Fig. 38.4). 5 , 6 In this regard, assumption or attainment of the lordotic posture augments stability by causing the facet joints to be engaged bilaterally. Of note, several ligaments span multiple spine segments. Therefore, dividing a long ligament at an intermediate point decreases its efficacy and decreases its functional single motion segment impact on stability, thus somewhat diminishing the clinical meaningfulness of short-segment biomechanical studies. Furthermore, longer specimens permit additional motion, as a result of the inclusion of a greater number of disc interspaces during the biomechanical testing procedure. As a result, both the complexity of the data acquired and its analysis are increased. This decreases the validity of the test result and its clinical relevance.

38.1.6 Forces and Moments
A spinal motion segment or vertebra becomes stiffer as it is progressively deformed. Similarly, rapid loading rates are associated with greater stiffness than slower loading rates. The duration, configuration, and magnitude of bending moments should replicate the clinical situation to be studied. The researcher should be cognizant of these factors when interpreting laboratory data.
One or more of several loading vectors may be applied to the spine during a biomechanical study. If more than one is used, loading is considered to be complex. An increase in complexity of spinal loading essentially decreases, in an exponential manner, the validity of a study. In addition, pure axial loading is associated with unpredictable eccentric spinal element loading. This is particularly so if the normal spine posture is not used and/or multiple motion segments are studied (see Fig. 38.4 and Fig. 38.5). 6

Pure bending moment application is difficult, if not impossible, to achieve absolutely because at least some component of an applied bending moment is axial in nature. This translates into some, although perhaps trivial, axial load application (Fig. 38.6). Of note is that an apparatus and experimental design have been developed to accomplish the task, at least in part, of providing a pure moment. 7 Biomechanical model standards, though, are clearly needed. 8

38.1.7 Intermediate Muscle Force Application
To accurately replicate the in vivo situation, the researcher must simulate intermediate muscle load applications. This is of great biomechanical significance, 9 – 11 particularly if multiple motion segments are being studied (Fig. 38.7). The difficulty with the application of intermediate muscle forces, however, is that they are difficult to replicate and that unknown and often immeasurable variable(s) are introduced. This makes the addition of simulated muscle forces in biomechanical studies a guess at the very best.

In addition, muscle forces apply diagonal and axial force vectors. This results in an increase in the applied axial load (Fig. 38.8a). Furthermore, an asymmetric diagonal load application can result in a rotational or component that is due to the coupling phenomenon (Fig. 38.8b). If the spine specimen is rigidly potted at each end, as is usually the case, the rigid fixation at the termini of the specimen will resist the aforementioned rotational component. This introduces significant error and substantially taints the results.

38.1.8 Type of Test
There are four basic types of biomechanical testing procedures: (1) strength, (2) fatigue, (3) stability, and (4) mathematical tests. Strength testing involves construct loading to determine load-bearing or load-resisting capacity. Fatigue testing determines the ability of a construct to withstand cyclically applied loads, usually until a fusion would theoretically at least partially mature (about 80,000 to 100,000 cycles) or an implant would fracture (more than 1 million cycles). Stability testing assesses the ability of the construct to minimize fusion-degrading motion. This is an indicator not only of stability but also, more importantly, of implant or construct stiffness (or flexibility). Finally, mathematical testing can be used. This strategy usually employs a finite element model (FEM) and finite element modeling computerized strategies.
Strength Testing
Strength testing, also known as load-to-failure testing, involves the application of a load, usually gradually, until the construct fails. A load–deformation curve is thus generated (Fig. 38.9). Photography and motion analysis, as well as the analysis of stresses and strains at the point of failure with electronic equipment (e.g., extensometers, goniometers, and strain gauges), may be employed to enhance the ability to assess the characteristics and nuances of failure. This documents not only the time of failure, but also its geometry. Strength correlates with load magnitude and deformation at the time of failure. Stiffness can also be determined from the data (slope of load–deformation curve; see Fig. 38.9 and Fig. 38.10).

Strength testing in a load-to-failure manner assesses the integrity of the spine or a spinal construct. It is therefore useful to study the mechanisms of spine failure and the theoretical efficacy of spinal instrumentation constructs and techniques. 12 As stated previously, the failure load (load applied at the moment of failure) and the extent of deformation at the time of failure can be used to assess construct strength and stiffness. This information may also be attained from the load–deformation curve (see Fig. 38.10). Stiffness is measured as the slope (of the tangent) of the load–deformation curve, usually in the elastic zone (within which a nearly linear stress/strain relationship is usually present). Energy absorption may also be assessed (Fig. 38.11). 6 Measurement of these parameters provides insight into the mechanical properties of the specimen.


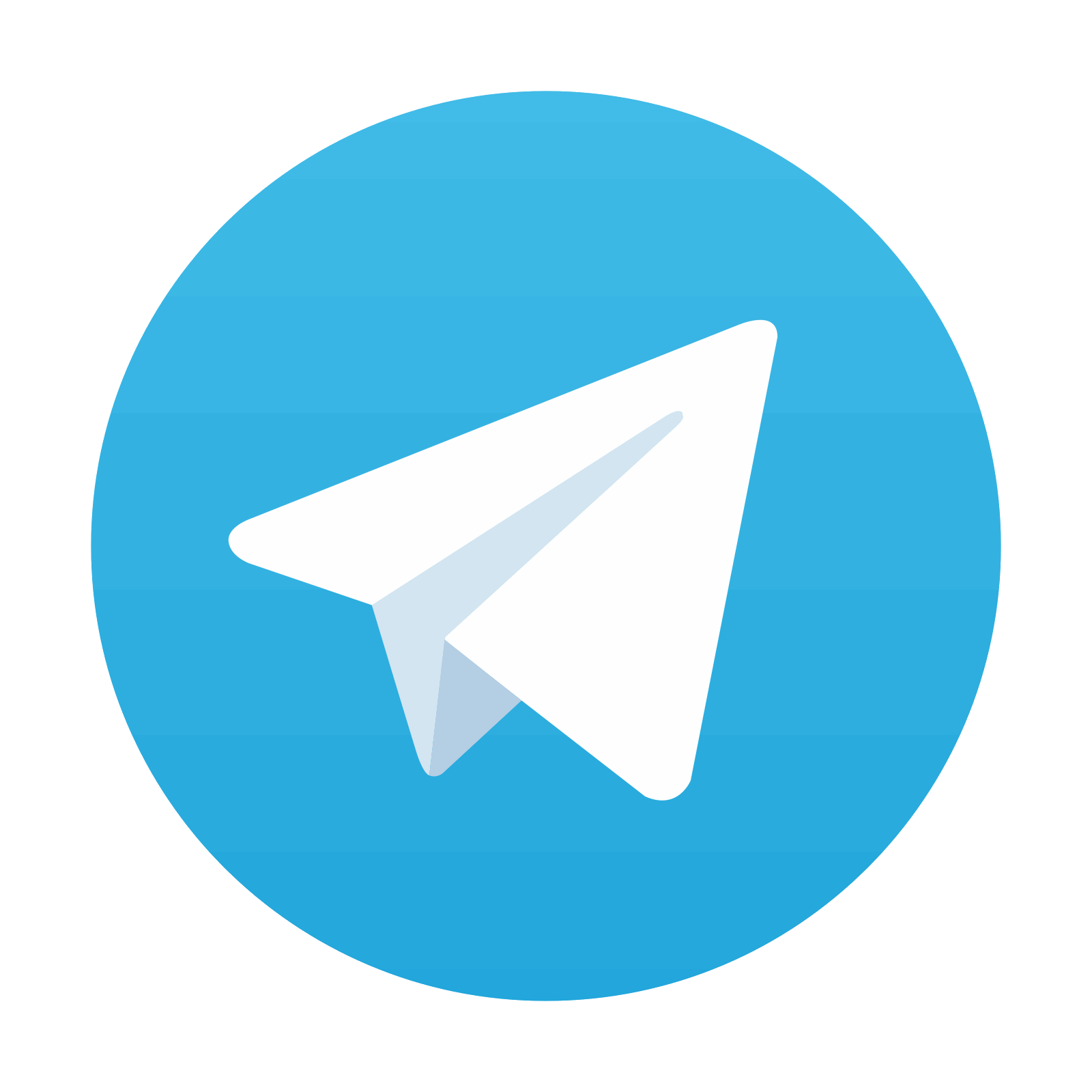
Stay updated, free articles. Join our Telegram channel

Full access? Get Clinical Tree
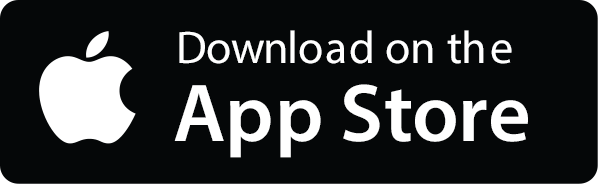
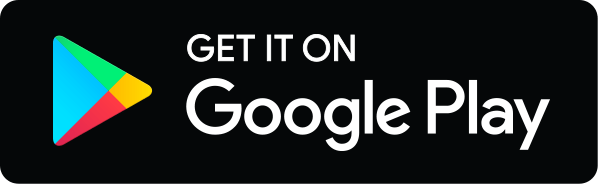
