4 Pathology of the Brainstem
Abstract
The brainstem contains all cell types of the central nervous system. Consequently, the brainstem may be involved in infectious, oncologic, neurodegenerative, and vascular disease processes. This chapter highlights the common pathological processes affecting the brainstem.
Introduction
The brainstem is an anatomical and functional hub of the central nervous system (CNS) that not only serves as a conduit for nearly all the information between the brain and the spinal cord and elsewhere, but also performs numerous vital functions by the presence of cranial nerve nuclei and centers of control for many essential functions. Even the smallest lesion may have profound effects on brainstem function. From a histological standpoint, the brainstem contains all the cellular elements found throughout the CNS, including neurons, glia, leptomeninges, ventricular surfaces, and a rich vascular supply. Thus, almost all pathological processes found elsewhere in the CNS may be represented in the brainstem, sometimes in isolation or in conjunction with diffuse or multifocal CNS diseases. For obvious reasons, much of the abnormal pathology of the brainstem cannot be easily assessed by large biopsies or resections and is therefore elucidated by neuroradiological studies and autopsy-based examinations.
Developmental and Acquired Malformations
Midbrain
Aqueductal Abnormalities
The cerebral aqueduct of Sylvius is the most common site of obstruction in noncommunicating hydrocephalus. In normal fetal development, the aqueduct is comparatively more distended than in the adult form. The normal adult aqueduct at its narrowest point, usually at the level of the middle of the superior colliculus, ranges between 0.4 and 1.5 mm2 in cross-sectional area 1 and is subject to anatomical variation in length and contour ( Fig. 4.1 ). The normal aqueduct may display forking, depending on the level and angle of the section. Consequently, this finding should not necessarily be considered pathological if seen in the setting of hydrocephalus.

The cerebral aqueduct may be considered abnormally narrow if it is less than 0.15 mm2 in cross-sectional area. Complete obliteration has been referred to as atresia, whereas incomplete obstruction is considered stenosis. Stenosis may be sporadic, X-linked, or, rarely, autosomal recessive. Traditionally, aqueductal narrowings are divided between malformations lacking gliosis and acquired causes with gliosis; however, some forms of experimental viral infection in mice have resulted in aqueductal stenosis without gliosis. Thus, some stenotic conditions without gliosis may be acquired. It has been proposed that aqueductal stenosis may be the result of compression by the expanding hydrocephalic hemispheres in utero on the midbrain tectal plate and the normally developing aqueduct. 2
A particular form of aqueductal stenosis is X-linked recessive in inheritance. 3 This inherited form of aqueductal stenosis accounts for 2% of congenital hydrocephalus. 4 The degree of hydrocephalus may vary widely. Adduction-flexion deformity of the thumbs was reported in 25% of cases. 5 X-linked recessive aqueductal stenosis is associated with congenital absence of the pyramids and is attributed to mutations in the L1CAM gene. Stenosis of the aqueduct is severe in some cases and relatively normal in others. 6 Atresia of the aqueduct may result in an abnormally complex forked configuration. 7
Other forms of atresia result in the lack of a recognizable aqueduct, which is represented by small tubules and other ependymal-lined canals without associated gliosis. This lack of a recognizable aqueduct has been associated with Arnold-Chiari malformation, hydranencephaly, craniosynostosis, 8 or arhinencephalic syndromes, 9 or seen in isolation.
Gliotic obliteration of the aqueduct is marked by the coexistence of widespread ependymitis involving other ventricular walls, commonly associated with meningitis with ventriculitis. 10 Gliotic obliteration of the aqueduct may also occur as a gradual process with a diversity of occlusive causes. Intraventricular hemorrhage in the premature brain or occlusion by necrotic tissue, such as in hydranencephaly, may also be responsible for gliotic obliteration. This type of cerebrospinal fluid (CSF) obstruction tends to result in the gradual onset and worsening of obstructive hydrocephalus in childhood or even adulthood. Microscopy reveals residual nests of ependyma indicating the profile of the aqueduct, whereby the lumen is occluded by an invasive glial reaction. The presence of a thin translucent septum resulting in hydrocephalus is a rare cause of aqueductal obstruction and may be a variant of gliotic stenosis. 11
Pons
Pontocerebellar Hypoplasia
Pontocerebellar hypoplasia (PCH) is group of rare inherited progressive neurodegenerative disorders with prenatal onset that affect growth and function of the brainstem and cerebellum, resulting in little or no normal development. Ten different subtypes have been reported. They are classified based on the clinical presentation, genetic characteristics, and the spectrum of pathologic changes. 12 PCH type 1 (PCH1) is characterized by central and peripheral motor dysfunction associated with cell degeneration in the anterior horn. This condition resembles infantile spinal muscular atrophy and results in early death. In PCH2, there is progressive microcephaly from birth combined with extrapyramidal dyskinesias. PCH3 is characterized by hypotonia, hyperreflexia, microcephaly, optic atrophy, and seizures. PCH4 is characterized by hypertonia, joint contractures, olivopontocerebellar hypoplasia, and early death. Patients with PCH5 have cerebellar hypoplasia, which is apparent in the second trimester, and show seizures. PCH6 is associated with mitochondrial respiratory chain defects. All subtypes share common characteristics, including hypoplasia and atrophy of the cerebellum and pons, progressive microcephaly, and variable cerebral involvement.
The brainstem pathology of PCH is mostly overshadowed by the pathology in the cerebellum, in which the neocerebellar hemispheres are severely atrophic with relative sparing of the flocculi and vermis and fragmentation of the cerebellar dentate nucleus. The basis pontis (the ventral or basilar part of the pons) is very shallow, with few transverse fibers, and often with significant gliosis ( Fig. 4.2 ). Hypoplasia or dysplasia of the inferior olives may also be present.

Medulla
Inferior Olivary Abnormalities
Several types of abnormalities occur in the inferior olive ( Fig. 4.3 ). Olivary heterotopia may be single or multiple and assume a number of sizes and conformations whereby there is variable folding and surrounding by neuropil, much like normal inferior olives ( Fig. 4.3a ). Most occur laterally near the inferior cerebellar peduncle ( Fig. 4.3b ). The normal migration of inferior olivary neurons occurs before the end of the third month of gestation. 13 Therefore, cerebral agyria or pachygyria may coexist with inferior olivary heterotopia. Other conditions associated with olivary heterotopia include the Dandy-Walker syndrome, pyruvate dehydrogenase deficiency, megalencephaly, and trisomy 13. 6

The inferior olives may also show a number of other dysplastic abnormalities with certain known associations ( Fig. 4.3c-f ). They are neither known to occur in isolation nor associated with any symptoms. These include Joubert syndrome, Dandy-Walker malformation, Miller-Dieker syndrome, Zellweger syndrome, dentate-olivary dysplasia with intractable seizures in infancy, trisomies 13 and 18, pachygyria, thanatophoric dysplasia, and others. Importantly, the inferior olives and dentate nuclei of the cerebellum have a common ancestry in the rhombic lip; therefore, dysplasias of the dentate nuclei may frequently coexist with those of the inferior olives.
Inferior Olivary Hypertrophy
A distinctive form of pathology of the inferior olives occurs with transsynaptic degeneration when accessory and inferior olivary neurons lose synaptic input, such as from interruption of the transsynaptic degeneration of the inferior olivary nucleus after injury to the dentato-rubro-olivary pathway, as occurs in an infarct to the ipsilateral central tegmental tract, or surgical disruption in the removal of cerebellar tumors in childhood. Some patients develop palatal myoclonus.
Histologically, unlike the usual atrophy and degeneration seen with other neurons of the CNS following deafferentation, the neurons of the inferior olives undergo hypertrophy with microscopic vacuolation, atypia, dispersal of Nissl substance, and even neurofibrillary tangle formation ( Fig. 4.4 ).

Pyramidal Tract Anomalies
Corticospinal tracts may display a variety of abnormalities, best visualized in the pyramids of the medulla. These include exaggerated fasciculation, in which the fascicles are divided into ovoid bundles separated by glial tissue. 14 Aplasia of corticospinal tracts is a predictable feature of anencephaly, holoprosencephaly, or hydranencephaly; they also may be associated with X-linked congenital aqueductal stenosis. 15 Corticospinal tracts may also show unilateral hypertrophy with associated asymmetric decussation, due to an actual increase in the number of fibers in the enlarged pyramid. This abnormality causes dorsal displacement of the ipsilateral inferior olive.
Tumors
Tumors of the brainstem include many among the primary neuroepithelial tumors, including astrocytomas, glioneuronal tumors, and very rarely oligodendrogliomas. Given the predilection of childhood brain tumors to originate in the posterior fossa as a whole, it is important to make the distinction between tumors arising in the brainstem itself versus those originating in the cerebellum, such as medulloblastomas, cranial nerve tumors, and intraventricular tumors such as ependymomas, which may compress or encircle the brainstem secondarily ( Fig. 4.5 ). This section will describe tumors that arise within or frequently involve the brainstem. Among these tumors, it is useful to subdivide those that typically arise in the midbrain, pons, or medulla.

Midbrain
Tectal Glioma
Clinical Features
The tumor most associated with a primary location in the midbrain is also known as the tectal glioma. Its location is prone to cause aqueductal stenosis and obstructive hydrocephalus. Magnetic resonance imaging (MRI) examination typically reveals a dorsally exophytic mass extending from the quadrigeminal plate. 16 Tectal gliomas tend to be indolent and rarely cause neurological impairment. Progression occurs in 15 to 25% of tumors. 17 Management is usually directed toward relief of hydrocephalus by shunting or endoscopic third ventriculostomy followed by observation. Biopsy and adjuvant therapy are reserved for patients with radiographic and clinical progression.
Pathology
Histological documentation, not to mention contemporary molecular profiling, is virtually nonexistent in published cases of tectal glioma. However, in keeping with their indolent clinical behavior, the majority of these tumors that have been biopsied show low-grade histology, diagnosed as World Health Organization (WHO) grade I or, more frequently, grade II astrocytomas, although other low-grade gliomas, including oligodendrogliomas and ependymomas, have been reported. 18 , 19
Pons
Diffuse Intrinsic Pontine Glioma
Clinical Features
A large series conducted by Schroeder et al found that 15% of malignant primary brain tumors in children under 20 years old arise in the brainstem, with the majority being the diffuse intrinsic pontine glioma (DIPG) subtype. 20 DIPG is a leading cause of brain tumor-related death in children, with a median survival of less than 1 year; more than 90% of children die within 2 years of diagnosis. 21 The median age of diagnosis is 6–7 years, and patients typically present with brainstem dysfunction or CSF obstruction over a period of 1 to 2 months. Additional neurological signs include cranial neuropathies, long tract disturbances, and ataxia. 22 DIPGs also account for 45 to 50% of brainstem gliomas in adults. 23
The expansion of the pons with convex intrusion into the fourth ventricle is so characteristic of these lesions as a midsagittal radiographic finding that biopsy is usually considered unnecessary. No effective treatment has been found, despite the standard administration of radiation therapy and chemotherapy. 24
Pathology
The 2016 WHO classification of brain tumors includes the DIPG as a form of diffuse midline glioma that is H3K27-mutant and classified as WHO grade IV. 21 The malignancy tends to produce distortion and enlargement of the pons by diffuse infiltration of the pontine parenchyma. Necrosis and hemorrhage may be seen. Microscopically, there is infiltrative growth into gray and white matter. The tumor cells vary from small and monotonous to large, pleomorphic forms ( Fig. 4.6a, b ). Nuclear morphology may be reminiscent of astrocytic differentiation, with hyper-chromatic irregular shapes, or it can show oligodendroglial morphology. Ten percent lack mitotic figures, microvascular proliferation, and necrosis, and therefore qualify only by microscopic criteria for WHO grade II status. The remainder show high-grade features with features of glioblastoma. Regardless, all forms are associated with the same dismal prognosis.

The diagnosis of a H3K27M glioma can be reliably done by immunohistochemistry; however, diagnosis is aided by concurrent immunostaining for the H3K27me3 (trimethylated) protein, which is seen in H3K27 wild-type cells ( Fig. 4.6c, d ). Among pontine gliomas with the H3K27 mutation, a recent series shows that such tumors are highly represented within the pediatric patient population, with an average age at diagnosis of 7 years and only 1 adult among 18 patients with pontine gliomas that showed the H3K27 mutation. IDH1 mutations and H3K27 mutations are believed to be es sentially mutually exclusive. 25 , 26 This report also underscores the particular association of H3K27 mutant gliomas with the pons; midbrain and medulla gliomas do not show the mutation. In adults, gliomas of higher grade may be seen that more closely correspond to the histology and molecular profile of supratentorial malignant gliomas. 23 , 27
Pilocytic Astrocytoma
Clinical Features
The pilocytic astrocytoma is a WHO grade I astrocytoma. It may occur in many locations within the CNS, especially the cerebellum and optic-hypothalamic regions. A minority arise in the brainstem, which is estimated to account for 9% of all pilocytic astrocytomas, 28 or involve the brainstem by infiltrative growth from the cerebellum. In either circumstance, such tumors understandably carry a worse prognosis because of the difficulty in surgical options. 29
Brainstem pilocytic astrocytomas present similarly to cerebellar examples, as circumscribed cystic or lobulated lesions with bright contrast enhancement, but solid types may arise as dorsally exophytic lesions of the brainstem, often from the pontomedullary region. Thus, radiographic localization and characterization are important in distinguishing brainstem pilocytic astrocytomas from diffuse astrocytomas. Brainstem pilocytic astrocytomas are particularly associated with NF1 as part of a spectrum of radiographically detected focal regions of brainstem enlargement, which can be seen with or without contrast enhancement. A series of 125 patients with NF1 and brainstem abnormalities included one biopsied patient with proven pilocytic astrocytoma. The other lesions were mostly indolent and many remained stable or even regressed. 30
Pathology
Pilocytic astrocytomas display a tan-pink color with a distinctly mucoid texture, often recognizable even in the smallest specimens. The most classic microscopic appearance of pilocytic astrocytomas is that of a microcystic tumor with a rich fibrillary background that shows a biphasic pattern, alternating between dense eosinophilic vasocentric regions and looser intervening areas ( Fig. 4.7a ). Either of the two patterns may dominate the microscopic appearance of the specimen; therefore, the dense or compact differentiation when unaccompanied by looser areas is especially prone to misdiagnosis as a diffuse fibrillary astrocytoma. This may prove especially challenging with small brainstem biopsies.

At the cytological level, astrocytes in these tumors have elongated profiles (“piloid” or hair-like) with benign ovoid nuclei. Multinucleated cells may be prominent. Clear cell differentiation may be extensive, sometimes indistinguishable from that of oligodendrogliomas ( Fig. 4.7b ). Considering the distinct rarity of oligodendrogliomas in the brainstem, a clear cell glioma in the posterior fossa is in fact more likely to be a pilocytic astrocytoma. Vascular proliferation is characteristic and may be one of the most essential features, along with contrast enhancement, in distinguishing the pilocytic astrocytoma from a WHO grade II diffuse astrocytoma. Mitotic activity is very unusual, and when division figures are identified, they are often within vascular endothelial cells.
Rosenthal fibers are eosinophilic elongate or beaded structures, usually within the compact perivascular compartments; these fibers are also quite useful in securing the diagnosis of pilocytic astrocytoma ( Fig. 4.7c ). Eosinophilic granular bodies (EGBs) are more frequent in loose regions, whereas Rosenthal fibers are found in denser, compact, typically perivascular areas. Both EGBs and Rosenthal fibers share immunoreactivity for glial fibrillary acidic protein (GFAP), ubiquitin, and αB crystallin, 31 and EGBs may express α1-antichymotrypsin, α1-antitrypsin, and lysozyme. 32
Pilocytic astrocytomas are quintessential WHO grade I gliomas. However, “malignant” forms exist, marked by numerous mitotic figures (at least 4 mitoses/10 high-power fields at 400X), robust vascular proliferation, hypercellularity, and moderate-to-severe cytologic atypia, with or without necrosis, which may even be of the pseudopalisading type. A report of 34 cases did not appear to suggest that the brainstem is more vulnerable to this tumor type than it is to conventional pilocytic astrocytomas without anaplasia. 33 The tumors may have had a WHO grade I pilocytic astrocytoma precursor, coexistent pilocytic astrocytoma, pilocytic astrocytoma documented by previous biopsy, or exhibited characteristic pilocytic features in an otherwise anaplastic astrocytoma. A clinical history of neurofibromatosis type I or radiation therapy may be present. P53 abnormalities may highlight the anaplastic portions of these tumors. High mitotic rates and necrosis appear to be the best predictors of a poor prognosis. Notwithstanding these disconcerting features, which might otherwise equate with a diagnosis of glioblastoma, such lesions should be designated “anaplastic” or WHO grade III.
Ganglioglioma, WHO grade I
WHO grade I gangliogliomas consist of mixed glial and neuronal cell populations. The identification of gangliocytic dysplasia is of paramount importance, since other otherwise-pure astrocytomas may invade gray matter to the extent that the native preinvasion neuronal cytoarchitecture is not apparent. Binucleated forms and irregular cytoarchitectural orientations as defined by a haphazard array of cell processes are reliable findings ( Fig. 4.8a ). These structures may be accentuated by antineuronal antibody immunostaining, particularly for microtubule-associated protein 2 (MAP2), which helps reveal the haphazard and disorganized arrangement of neoplastic ganglion cells and multiple nuclei by their negative staining ( Fig. 4.8b ). The astrocytic portion of gangliogliomas, often highly reminiscent of pilocytic astrocytomas, is estimated to occur in approximately two-thirds of gangliogliomas. In lesions with strong contrast enhancement, prominent vasculature as is seen in pilocytic astrocytomas may also be found.

Subarachnoid spread is quite common in superficially located tumors, which does not necessarily portend a higher grade. Necrosis and mitotic activity are distinctly rare unless the astrocytic component warrants a worsening in grade, and according to current criteria, WHO grade II lesions are not formally recognized. Anaplastic examples warrant a WHO grade of III, which is determined by malignant changes in the glial component, such as increased cellularity, pleomorphism, and increased mitotic activity. 34
BRAF Mutations in Gliomas
Since the therapeutically targetable BRAF c.1799T>A (p.V600E) (BRAF V600E ) mutation is a key gene altered in most pediatric low-grade gliomas, it is relevant to the pathology of brainstem gliomas. Brainstem pilocytic astrocytomas showed a rate of BRAF V600E mutation in 10% of tumors, compared with 33% of diencephalic examples. 35 Another study showed that 1 of 8 (13%) brainstem pilocytic astrocytomas were BRAF V600E mutant. 36 Gangliogliomas in all locations show a relatively high rate of BRAF V600E mutation compared with other gliomas, second only to pleomorphic xanthoastrocytomas and epithelioid glioblastomas. In the brainstem, pediatric gangliogliomas also show BRAF V600E mutation in 54% of patients (7 of 13), 2 of which had equivocal immunohistochemical results, but those results were confirmed by an RNA-sequencing approach. 36
Rosette-Forming Glioneuronal Tumor
Clinical Features
Rosette-forming glioneuronal tumors typically show symptoms of obstructive hydrocephalus owing to the characteristic involvement of the midline location in the fourth ventricle and/or aqueduct, with possible cervical pain. They may extend into the adjacent brainstem, cerebellar vermis, pineal gland, or thalamus. Incidental findings on MRI occasionally are seen, 37 Separate supratentorial locations have also been described, as well as dissemination throughout the ventricular system. 37 Radiographically, they are usually relatively well-circumscribed with focal contrast enhancement.
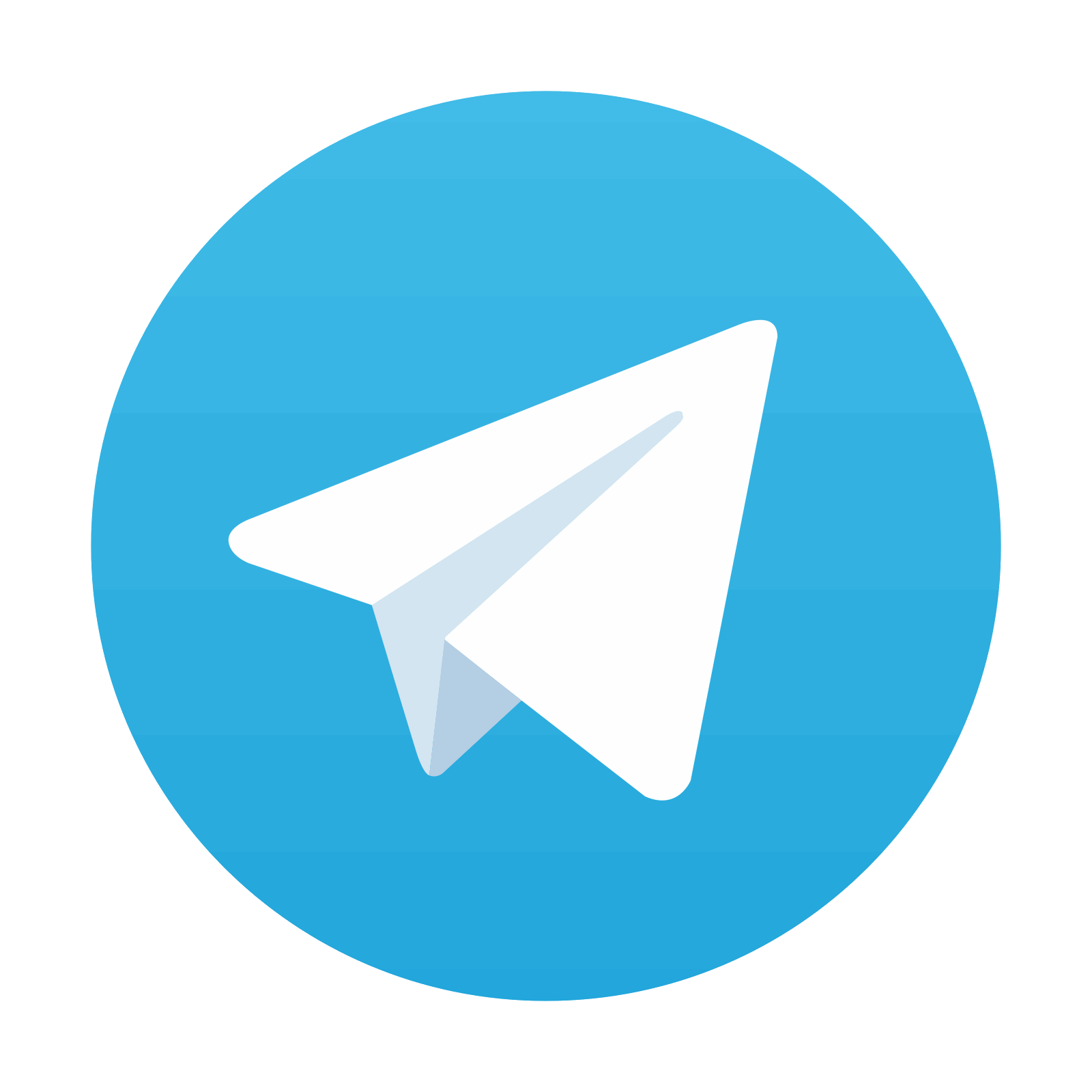
Stay updated, free articles. Join our Telegram channel

Full access? Get Clinical Tree
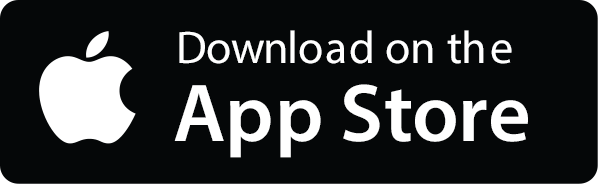
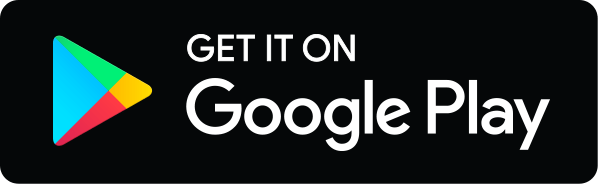
