40 Exoskeletons
Abstract
Spinal injury is a devastating event which currently has limited therapies. In the absence of restorative therapies, modalities to mimic motor function have been developed. These not only allow for independent motility, but can also provide much needed exercise. Exoskeletons are an example of this and heterogenous in their function. Broadly, they can be either active or passive. The simplest is external bracing and crutches in order to remain in an upright posture. More sophisticated units include feedback sensors and gait initiation sensors. In the future, even more sophisticated models may include the ability to climb stairs as well as have more normal stepping patterns.
Spinal cord injury is a debilitating event defined as a fixed neurological deficit originating in the spinal cord. There are 23 million men and women living with a spinal cord injury, and globally there are 180,000 annual new cases. 1 In 2103 this represented 273,000 Americans living with a chronic spinal cord injury with 12,000 new cases that year alone. 2 The dream of every paraplegic or quadriplegic is the recovery of locomotion, toileting and sexual function. Secondary, but also meaningful consequences of spinal cord injury, are bone density homeostasis, cardiac and pulmonary dysfunction, metabolic instability and skin breakdown. Many different exoskeleton types are in development to deal with these issues.
One hour of exercise three days a week maintains cardiovascular conditioning and decreases mortality rate by 20%. 1 For a healthy person this means a daily one-hour walk at three mph three days weekly. 3 , 4 The sedentary lifestyle of a spinal cord injured patient also can cause muscle atrophy and have a deleterious effect on bone mineral density and body weight. Lower extremity bone mineral density decreases 3–4% per month in the first year after spinal cord injury and patients gain an average of two kilograms each year. Two-thirds of them will become obese in time, but modest increases in activity can improve both bone mineral density and body fat composition. 3 , 4 Exoskeleton use can help with this if an individual is able to exercise for long enough to get the benefit.
Exoskeletons are classified as passive or active devices. The simplest ones are unpowered passive devices such as hip/knee/ankle orthoses. In the spine-injured individual, the ankle is fixed in position or spring-loaded to maintain the foot in dorsiflexion. The hip and knee have joints with limited degrees of freedom. A common configuration is flexion/extension of both the hip and knee without abduction or adduction at the hip, but these unpowered devices are difficult to use. 5 There are several reasons for this. The effort to stand requires use of the arms to maintain balance and provide forward motion, since there is limited assistance from the trunk. The legs are locked in position and swung forward to execute a step. The many elements to such movement require energy exertion that cannot be sustained long enough to confer a metabolic advantage. 1 , 6 , 7
With training, powered exoskeletons can meaningfully reduce the effort a chronically injured individual needs to be able to walk. 1 Exoskeletons diminish spasticity and improve well-being. Powered exoskeletons are classified as fully assistive or augmentative. Augmentative skeletons make use of functional electrical stimulation (FES). FES recruits local muscles by transdermal or percutaneous stimulation. A controller elicits muscle contractions to augment exoskeleton movement. FES based systems can also help with the metabolic benefits of locomotion, but there is a significant limitation. The muscular recruitment created is coarse, and there is a recruiting preference to fast twitch muscle fibers. These fatigue rapidly. 1 , 5 This can be painful for patients with incomplete injuries. While muscle activation can be achieved with FES, control of the limb can be quite limited. Delivery of current may induce spasms within the muscle as well as asynchronous muscle group engagement. The asynchrony can stress joints, causing pain, or it can induce resistance to the exoskeleton gaiting. Without feedback to the controller, the limb completing the movement could be hurt. Patients with chronic spinal cord injury have reduced recruitable muscle mass and the muscle that is present is often shortened causing contracture across joints. Because of high recruitment thresholds and inefficient energy usage, FES skeletons have limited useful function in sustained weight bearing walking in paraplegics. They do not eliminate the need for crutches, nor are they able to recruit abdominal wall or erector muscles in order to stabilize the torso when walking. However, FES systems can stimulate muscle tissue and generate trophic factors. This could increase muscle bulk and volume if they are incorporated into a static training regimen (▶ Fig. 40.1).

Unassisted powered exoskeletons are subdivided into the type of feedback system present. While all powered systems have some feedback system, an EMG sensing system is unique. It gets feedback from the wearer giving him volitional control to the gait. 5 , 6 Triggering of the EMG sensing system produces a preprogrammed gait response. For this to happen, some volitional control of the muscle group is needed to trigger the cycle. A potential shortcoming of this sort of system would be inappropriate generation of a gait cycle from a spontaneous EMG stimulus. This could arise from chronic muscle denervation or spasm. These EMG sensing systems could help chronically deconditioned but uninjured elderly users who are unable to walk for meaningful distances. This could improve the quality of their lives and maintain their independence. Unassisted powered exoskeletons have feedback systems that can initiate a gait cycle using simple gesturing. The most intuitive is one that actuates when the user leans to the side desired in order to start a gaiting cycle. Regardless of the type of powered system, the majority of units also require upper extremity involvement to maintain an upright posture. The REX bionics exoskeleton is a stand-alone unit in production that will do this, but it is not FDA approved in the United States. The unit uses hand control gesturing to initiate a gait cycle. There are three exoskeletons that are FDA approved. These are the ReWalk exoskeleton, Ekso GT and Parker Indego.
Of importance in any exoskeleton is its controller. This device determines the degrees of freedom (DOF) conferred on each active and passive joint. The hip is usually allowed passive rotation and the ankle is allowed passive dorsiflexion and plantar flexion. 7 Included in the software design is a gait cycle program that is either generated from the recorded gaits of uninjured wearers or extrapolated from a gait atlas. 7 The latter produces a more natural joint trajectory. An easier to implement and simpler gait program is a direct trajectory program based on a reference gait. Such a program can be difficult to implement since the joint trajectories tend not be natural. Also included in some controller functions are programmed positions for sitting and standing. Exoskeleton stair climbing has not yet been implemented in commercially available models. In the future, this along with slip detection and correction using a closed loop system are all desired goals.
References
[1] Miller LE, Zimmermann AK, Herbert WG. Clinical effectiveness and safety of powered exoskeleton-assisted walking in patients with spinal cord injury: systematic review with meta-analysis. Med Devices (Auckl). 2016; 9:455–466 [2] Lajeunesse V, Vincent C, Routhier F, Careau E, Michaud F. Exoskeletons’ design and usefulness evidence according to a systematic review of lower limb exoskeletons used for functional mobility by people with spinal cord injury. Disabil Rehabil Assist Technol. 2016; 11(7):535–547 [3] Karelis AD, Carvalho LP, Castillo MJ, Gagnon DH, Aubertin-Leheudre M. Effect on body composition and bone mineral density of walking with a robotic exoskeleton in adults with chronic spinal cord injury. J Rehabil Med. 2017; 49(1):84–87 [4] Miller LE, Herbert WG. Health and economic benefits of physical activity for patients with spinal cord injury. Clinicoecon Outcomes Res. 2016; 8:551–558. eCollection 2016 [5] Arazpour M, Samadian M, Bahramizadeh M, et al. The efficiency of orthotic interventions on energy consumption in paraplegic patients: a literature review. Spinal Cord. 2015 [6] Ha KH, Murray SA, Goldfarb M. An Approach for the Cooperative Control of FES With a Powered Exoskeleton During Level Walking for Persons With Paraplegia. IEEE Trans Neural Syst Rehabil Eng. 2016; 24(4):455–466 [7] Yan T, Cempini M, Oddo CM, Vitiello N. Review of assistive strategies in powered lower-limb orthoses and exoskeletons. Robot Auton Syst. 2015; 64:120–136 [8] Contreras-Vidal JL, A Bhagat N, Brantley J, et al. Powered exoskeletons for bipedal locomotion after spinal cord injury. J Neural Eng. 2016; 13(3):031001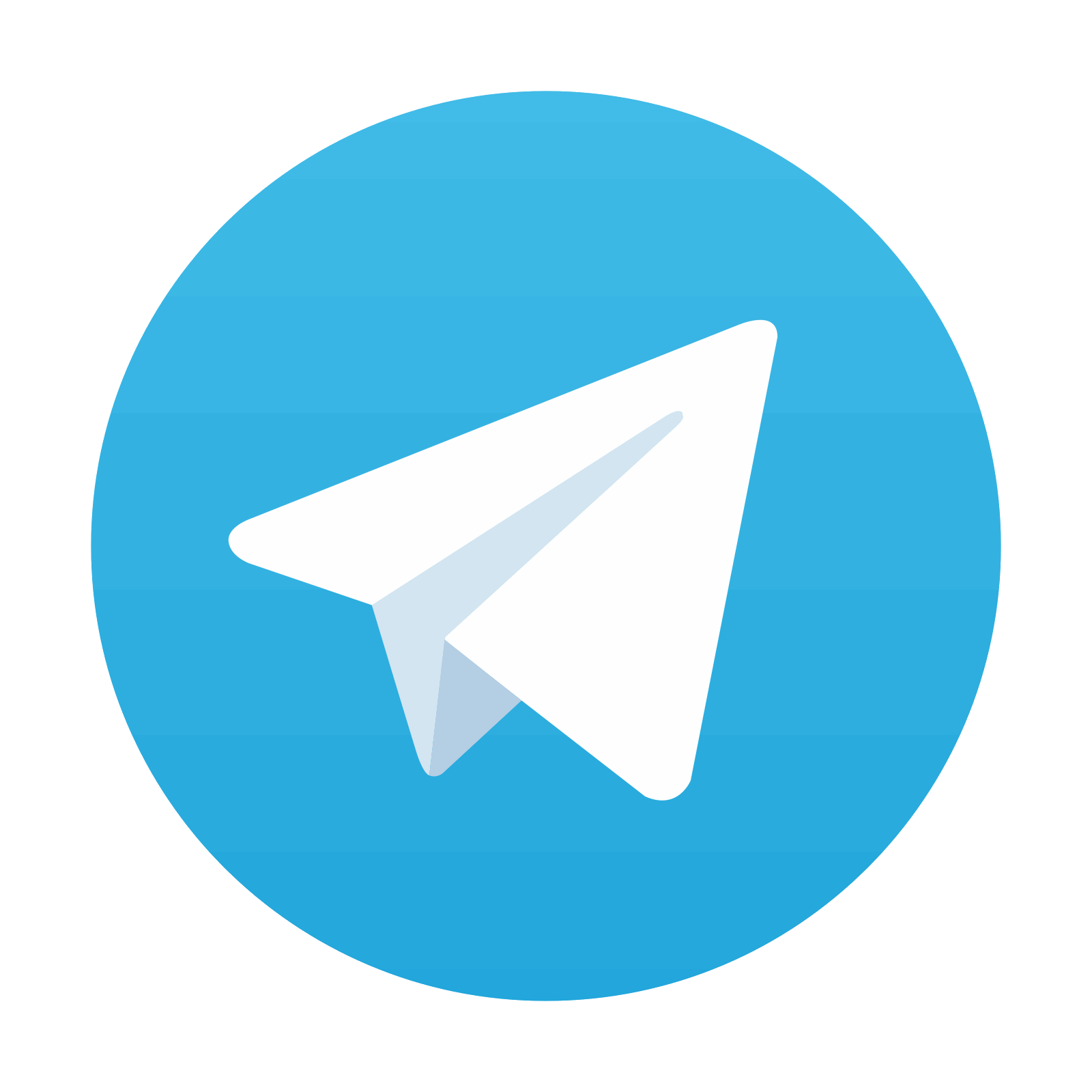
Stay updated, free articles. Join our Telegram channel

Full access? Get Clinical Tree
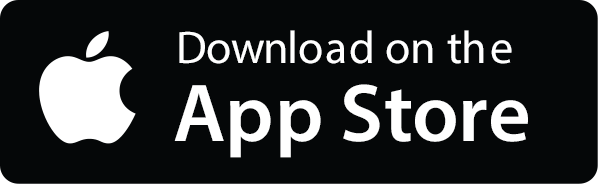
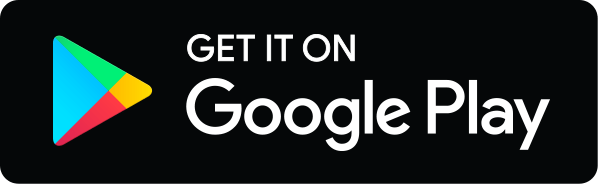