41 Visual Prostheses
Abstract
The field of electrical implants for vision restoration in the blind is rapidly advancing, though there are many blind individuals who will not be suitable for retinal electrical prostheses. The lateral geniculate nucleus and visual cortex are alternate implantation sites which bypass the retina and optic nerves. Neurosurgeons are involved in the development and implantation of bionic vision prostheses to these sites. This chapter describes how these prostheses are being designed and applied with a focus on cortical vision prostheses.
41.1 Introduction
Electrical stimulation of the visual pathways partially restores visual function by generating phosphenes (spots of light). The aim of a bionic vision device is to create reproducible and rapidly changing patterns of phosphenes in the visual field to enable the blind person to regain sufficient vision to improve their daily activities. These activities include object recognition and safe, reliable navigation. Facial recognition is the ultimate goal. Blind individuals use their remaining senses very effectively to undertake daily activities. Visual prostheses complement these skills. Potential targets in the central nervous system for electrical stimulation to restore vision are the retina, optic nerve, lateral geniculate nucleus (LGN), and the visual cortex.
In blind individuals with retinal or optic-nerve degeneration, an approach bypassing the proximal visual pathways is required. For these patients, neurosurgeons are able to surgically access the LGN and the visual cortex. The last human trial of an implantable cortical vision prosthesis was in the year 2000. 1 , 2 , 3 Since then, there have been significant technological advances in micro-electronics, electrodes, wireless power, data transmission and human studies demonstrating that electrical stimulation of the visual cortex produces highly localized phosphenes. 4
41.2 Sites in the Central Nervous System for Implantation of Visual Prostheses
(See ▶ Table 41.1)
The retina: When photoreceptor cells are lost through inherited disease such as retinitis pigmentosa (RP), light no longer stimulates the retina and the individual becomes blind. Electrical stimulation of the residual neural components of the retina (amacrine and ganglion cells) produces phosphenes, 5 There are epiretinal, subretinal and suprachoroidal devices. Placing electrode arrays on or near to the retina provides access to a large visual field, avoids cranial surgery and allows unilateral device design. Use of such devices for severe macular degeneration is being investigated.
Cause of blindness | Retinal Implant | Cortical/LGN Implant |
Glaucoma | x | |
Diabetic Retinopathy | x | |
Bilateral traumatic vision loss | x | |
Large pituitary/parasellar tumors | x | |
Eye tumors (e.g. bilateral retinoblastoma) | x | |
Optic atrophy/optic neuritis | x | |
Retinitis Pigmentosa | x | x |
Aged Macular Degeneration | maybe | x |
Optic nerve: Electrodes can be placed as a cuff on the surface of the optic nerve or, with penetrating electrodes, in the optic nerve head or the optic nerve. However, electrodes placed on the dural surface require higher currents and the spatial resolution is inferior compared with penetrating electrodes. Patients with RP could be trained to achieve some pattern recognition, shape orientation, object localization. 6 , 7 The indications for optic nerve electrode implantation are the same as for retinal implants. The surgical approaches to the optic nerve are by craniotomy or an intraorbital approach. 8
Lateral geniculate nucleus (LGN): The LGN is a small nucleus (about 250-mm3 volume and up to 10-mm long) located in the posterior/inferior part of the thalamus. The central 10 degrees of vision occupies the posterior half of the nucleus. The LGN has six layers of neurons in humans and is suitable for multi-electrode stimulation because it has retino-topic representation. The LGN remains intact after the retinas and optic nerves have lost function and this widens the number of possible indications (see ▶ Table 41.1). 9 , 10 The LGN could also potentially be used as a stimulation target if the visual cortices have been injured or lost (cortical blindness).
Pezaris and Eskander described their concept of a microelectrode bundle splaying out from the end of the electrode sheath into the LGN and with electrodes spaced 1 mm apart in 3 dimensions, 250 electrodes for each hemisphere could be placed on each side giving a total of 500 electrodes. Brush-like electrodes with 8 contacts have been inserted in the monkey LGN for stimulation experiments. 10 The stereotactic techniques required to place electrodes precisely in the LGN are similar to those used to place deep brain stimulation electrodes Parkinson’s disease and other movement disorders. 9 Once placed, these electrodes would be stable in position. A subcutaneous lead connecting to a subclavicular or cranial stimulator is planned for the human device. 9
Visual cortex: Electrical stimulation of the human visual cortex to restore vision in blind individuals has been evolving since the 1950s when electrodes were implanted on the surface of the visual cortex of a blind subject. 3 , 11 , 12 This allowed subjects to perceive phosphenes, identify shapes, navigate the environment, and to read Braille. These electrodes were implanted on the cortical surface, and since then, much work has been done in developing implants that have microelectrodes that penetrate the cortex (▶ Fig. 41.1). 1 , 2 , 3 , 12 , 13 Penetrating cortical microelectrodes (intracortical microstimulation) produce more localized phosphenes (discriminable when stimulated by electrodes 700-µm apart) at currents in the µA range. 3 , 14 , 15 This is more than a 100-fold reduction in necessary current output compared to surface stimulation and significantly lowers seizure risk.

The visual cortex is a relatively large area to place electrodes for partial restoration of central and peripheral vision compared with other potential targets. The total surface area of the primary visual cortex (V1) varies between 1400 and 6300 mm2, depending on the method of estimation, with approximately 67% of that area buried along the calcarine fissure. 16 , 17 , 18 If this cortex is not included in the stimulation, an hour-glass shaped visual field results. Head scanning which places the camera at different angles will help the individual ‘fill in the gaps’ and create a more complete picture of the visual scene. 19 Placing electrodes within the calcarine cortex may also require varying length electrodes and different carrier designs which would be more of a challenge to develop. Placement of electrodes in secondary visual cortex may cover some of the missing visual field. 12 Finally, insertion of electrodes into the medial surface of the hemisphere covers the peripheral vision and central vision but the orientation of these electrodes would be orthogonal to a transmitting coil on the scalp and wireless data transfer would be restricted unless there is some internal wired connection of the electrode carriers.
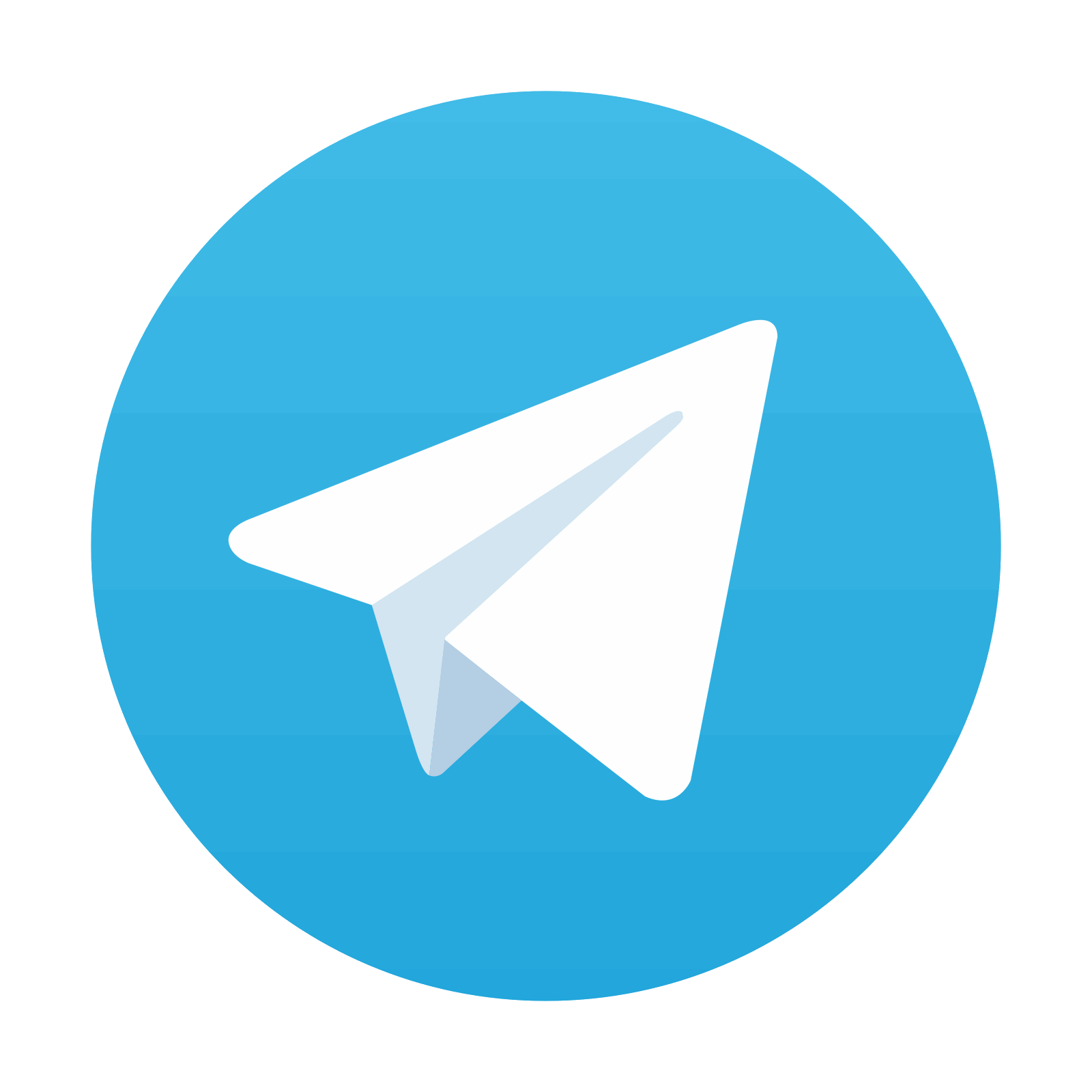
Stay updated, free articles. Join our Telegram channel

Full access? Get Clinical Tree
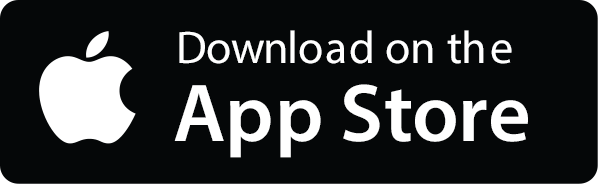
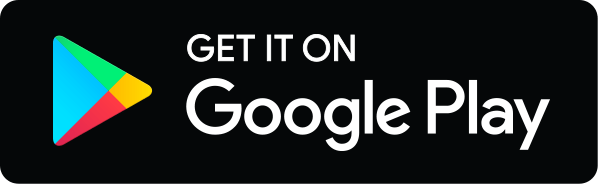