5.2 Instability Severity measures
1 Introduction to posttraumatic stability as related to classification systems
Biomechanical observations
A spinal injury classification system should provide information as to the extent of injury in structural and neurologic dimensions to aid in selecting the most appropriate treatment. Based on available outcomes in the literature, integrity of neurologic function is a prevalent parameter towards the final result. Similarly preservation of neurologic function by correctly predicting structural stability is of high clinical concern. Therefore, a classification system should be able to predict the severity of neurological injury or risk of incurring a secondary neurologic deficit using observable postinjury measures. A widely used technique to measure the extent of spinal trauma is derived from imaging the canal space postimpact and assessing the degree to which the midsagittal canal diameter has been occluded. However, it has been difficult to directly correlate the degree of neurotrauma with the observed geometric occlusion [1–5], even though occlusion should correlate with cord compression. A possible explanation for this apparent lack of correlation may be due to the elastic nature of the spine during impact. As the maximum load is reached, the spine deforms and the cord is occluded, but as the load is released the spine recovers some of its deformation, regaining height loss and reducing canal occlusion. Therefore the maximum occlusion of the canal, and the effect, transiently on the cord may be significantly greater than that observed by postinjury radiography. Similarly the static images obtained in a recumbent fashion with CT scan and MRI fail to take into account the potential of an injured spine segment to undergo secondary deformation under physiologic loads.
Biomechanical testing
The potential for dynamic vertebral deformation was tested in a fresh cadaveric experiment, shown in Fig 5.2-1 , with vertical compressive or compression/flexion loads applied. Volumetric and pressure changes to the spinal canal could be measured with a malleable plastic tube and a saline pump system attached [6]. The results shown in Fig 5.2-2 indicated a significant difference between occlusion measured during impact, immediately postimpact, and by postinjury CT scanning. During impact, the maximum canal occlusion (decrease in the midsagittal diameter) was 71.5%. Immediately post impact (while still in the test frame), it averaged 46.7%, demonstrating the amount of initial elastic recovery of the spine, and after removal of specimens and CT scanning, the average canal occlusion had decreased to 21.5%. The decrease from transient to postimpact canal midsagittal diameter was 70%. Therefore, most of the canal occlusion had disappeared postimpact. These findings, from the perspective of laboratory testing, demonstrate why it may be difficult to relate post-traumatic measured canal compromise with the actual occlusion that had occurred during the traumatic event. It stands to reason that a similar observation could be made for sagittal angular deformation, with a transient major bending deformation partially reducing by “springing back”. In addition, a spontaneous traumatic deformity reduction may be expected with recumbent patient positioning. Further variables to consider in measuring sagittal angular deformation can be expected with patient body habitus, mobility of spine segments, and type of support structure, such as a rigid board versus a soft mattress. In this context determination of injury severity by measuring static post-traumatic images in a number of dimensions may well under appreciate the actual deformation that the spinal column underwent at the point of impact.


2 Treatment consideration
A spinal injury classification system should also be a guide to the surgeon as to how to best stabilize the injured spine mechanically to prevent further damage to the neurological structures. We hypothesized that different spinal fracture patterns result in different patterns of damage and that some of the load carrying structures (the facets for example) may remain intact after specific types of trauma. In a different set of in-vitro tests we set out to identify the residual mechanical stability related to the type of fracture produced in the thoracolumbar spine. Mechanical stability of the spine pre and postinjury was defined in terms of the neutral zone concept described by Panjabi [7]. The neutral zone is an area in which the spinal motion segment can be positioned without applying significant load, and in which the spine will remain after the load is released. Every normal joint has a range of motion in which it displaces without requiring the application of significant load. When the joint is injured, this range increases. The neutral zone provides an easy to visualize method for describing the remaining spinal joint stability. For this series of experiments, thoracolumbar spinal fractures encompassing compression injuries, flexion-distraction injuries, as well as burst fractures were created by impact. Once damaged, loads were applied to specimens in flexion, left lateral bending, extension, right lateral bending, and torsion and in combinations of these loads. For each measurement, the load on the specimen was applied and then released, and the position of the center of the vertebra measured. The measured positions described an area in the horizontal plane, which correlated to the particular type of fracture induced. The outer boundary of the area is the limit of the unstable region, since load is required to move in any direction outside the boundary of the neutral zone.
Fig 5.2-3a demonstrates the difference in neutral zones between an intact vertebral motion segment and the same one after compression fracture. The specimen maintained almost complete mechanical integrity in flexion/extension/lateral bending as indicated by how the intact and injured specimen neutral zone boundaries overlap. However, the torsional neutral zone was increased significantly. In contrast, as Fig 5.2-3b shows, a burst fracture has a much wider neutral zone boundary in flexion and right and left lateral bending.
Currently the concept of a “neutral zone” has become a well recognized biomechanical term utilized widely in research endeavors. As the graphs show it is possible to express spinal stability increasingly well in a graphic fashion in an in-vitro setting. At this point, we currently still lack a translational application which would allow a neutral zone diagram to be applied to any given patient’s fracture based on expected biomechanical performance of the injured segment in order to allow the treating physician to choose the most appropriate care from a variety of nonsurgical or operative options. However, these results do indicate generally how different fracture patterns affect remaining mechanical stability of the spine after injury.

3 Summary
In summary, the current attempts at identifying ‘spinal stability’ remain somewhat arbitrary subjective, and are largely empirically based. Under-appreciation of spinal injury severity based on reliance on static recumbent postinjury films remains a real danger in management aspects. Ultimately preservation of neurologic function and optimization of its recovery potential in case of injury with restoration of a pain-free and physiologically aligned spinal column remain the parameters used for identifying a structurally stable spine. Hopefully translational research will enable application of visual decision-making aids in the future to help in determining stability needs of patients.
4 References
1. Denis F (1983) The three column spine and its significance in the classification of acute thoracolumbar spinal injuries. Spine; 8:817–831. 2. Fontijne WP, de Klerk LW, Braakman R, et al (1992) CT scan prediction of neurological deficit in thoracolumbar burst fractures. J Bone Joint Surg Br; 74:683–685. 3. Hashimoto T, Kaneda K, Abumi K (1988) Relationship between traumatic spinal canal stenosis and neurologic deficits in thoracolumbar burst fractures. Spine; 13:1268–1272. 4. Kang JD, Figgie MP, Bohlman HH (1994) Sagittal measurements of the cervical spine in subaxial fractures and dislocations. An analysis of two hundred and eighty-eight patients with and without neurological deficits. J Bone Joint Surg Am; 76:1617–1628. 5. Kilcoyne RF, Mack LA, King HA, et al (1983) Thoracolumbar spine injuries associated with vertical plunges: reappraisal with computed tomography. Radiology; 146:137–140. 6. Carter JW, Mirza SK, Tencer AF, et al (2000) Canal geometry changes associated with axial compressive cervical spine fracture. Spine; 25:46–54. 7. Panjabi MM (1992) The stabilizing system of the spine. Part II. Neutral zone and instability hypothesis. J Spinal Disord; 5:390–396. 8. Ching RP, Tencer AF, Anderson PA, et al (1995) Comparison of residual stability in thoracolumbar spine fractures using neutral zone measurements. J Orthop Res; 13:533–541.1 Frymoyer Classification of Degenerative Segmental Instability
Frymoyer JW, Selby DK (1985) Segmental instability. Rationale for treatment. Spine; 10:280–286.
SCALE DESCRIPTION
Degenerative segmental instabilities classified based on clinical, radiographic and biomechanical considerations:
Type I—Axial rotational
Type II—Translational
Type III—Retrolisthetic
Type IV—Postsurgical
Interpretation:
Descriptive of segmental instability. One type not necessarily more severe than the next.
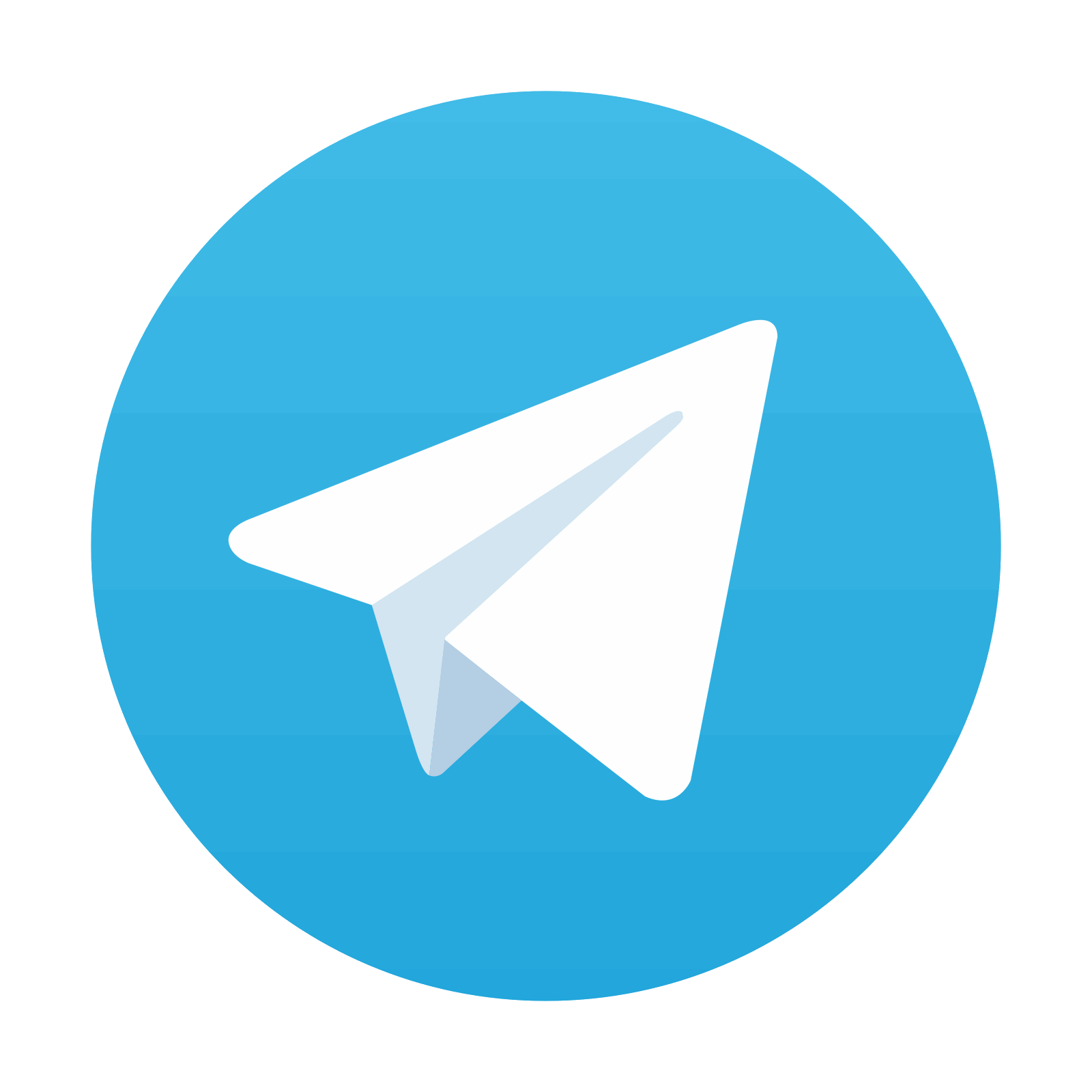
Stay updated, free articles. Join our Telegram channel

Full access? Get Clinical Tree
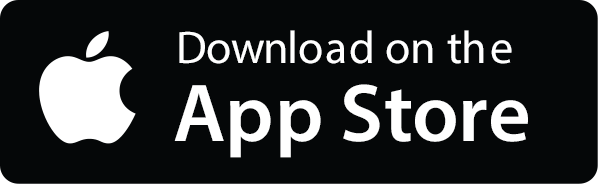
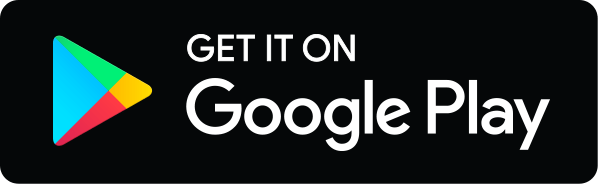
