Summary
Anesthesia support for skull base surgery is complex and is critical for the overall success of the procedure. The anesthesia care team must be aware of the positioning needs of the surgeon and must be able to protect the patient from neurologic injury caused by the abnormal positioning that may be required to access the tumor. In addition, the anesthetic technique must be tailored to ensure a hemodynamically stable patient and a still surgical field without the use of muscle relaxants. Anesthetic technique must also be adjusted to accommodate neurophysiologic monitoring so as to protect patients from neurologic injury during surgical resection. New and modern surgical techniques using minimally invasive technology raise new issues related to hemodynamic stability, fluid status, and overall monitoring of patient homeostasis. These endoscopic procedures offer advantages over open procedures but have their own set of problems that must be addressed by the anesthesiologist. Some of the common problems that anesthesiologists face during skull base surgery, along with methods for preventing and treating these injuries, are presented to help improve outcomes and morbidity after the procedure. Postoperative pain management is also described, including new and novel therapies with which to reduce postcraniotomy pain and headache—all designed to improve recovery and patient well-being after these complex surgeries.
5 Anesthesia and Intraoperative Monitoring of Patients with Skull Base Tumors
5.1 Introduction
Many issues surround the administration of anesthesia for patients undergoing surgery of the skull base. Not only do difficulties surround exposure of deeply seated anatomic structures, but other issues also involve positioning, prevention of iatrogenic nerve injury, and blood loss. This chapter describes many of the problems encountered with the delivery of anesthesia for skull base surgery and will describe some of the techniques and monitoring used to improve outcomes. The review of this subjective matter is meant to give some insight into the multiple and complex problems faced by the operative team during these procedures.
5.2 Patient Positioning
One of the challenges of skull base surgery concerns patient positioning. The exact approach depends on the patient’s anatomy and clinical status as well as on the tumor size. These approaches can be craniofacial, orbitocranial, infratemporal, suboccipital (transcondylar), and, in some instances, endonasal. Lateral approaches include the retrosigmoid, translabyrinthine, and orbitocranial zygomatic.1 Each of these approaches is associated with particular positions that have specific challenges and morbidities.
5.2.1 Supine Position
Anterior skull base lesions can be quite difficult to approach surgically, even though the patient is in the supine position. Access to the airway is simple, although the patient may be rotated 180° away from the anesthesia team. There are limited changes in the patient’s hemodynamic profile, but there could be significant changes in the pulmonary system, especially related to diaphragmatic elevation. This diaphragmatic position ultimately promotes atelectasis and ventilation-to-perfusion (V/Q) mismatching. The supine position is also used for endonasal endoscopic approaches to the anterior skull base, which includes the sella, cribriform plate, planum sphenoidale, and suprasellar cistern, as well as the clivus, pterygopalatine fossa, and adjacent parasagittal skull base locations.
Lateral approaches such as the retrosigmoid, translabyrinthine, and orbitocranial zygomatic can be accessed via the supine position but require the head to be laterally rotated and fixed. This flexion is sometimes impossible, especially in the elderly, and may be associated with venous obstruction.2 This positioning reduces the risk of air embolism but can produce brachial plexopathy on the side of the surgery, because the head is turned contralaterally and the ipsilateral shoulder is pushed down to allow access for the surgical approach. Closed claims analysis of brachial injury notes that they usually are associated with general anesthesia, with 10% related to patient positioning (head down, malpositioning of the arms, and sustained neck extension).3 Retrospective findings from our own surgical database demonstrated a 9% incidence of brachial plexopathy, with 61% ipsilateral to the surgical field. In most cases, these symptoms resolve within two to three days.1 Care providers should be focused on the initial positioning to protect the patient from overextension and flexion injuries.
5.2.2 Prone Position
The prone position is particularly useful for accessing lesions at or near midline and the fourth ventricle.4 This positioning is associated with numerous hemodynamic and respiratory changes that must be monitored closely. Significant V/Q mismatching is present in the prone position, and access to the airway is compromised. The anesthesiologist must be vigilant during positioning of the patient, because dislodgement of the endotracheal (ET) tube will require emergency reintubation in suboptimal conditions. Transferring patients from supine to prone positioning produces significant hemodynamic changes secondary to acute changes in preload from either abdominal compression or thoracic impedance. Cardiac dysrhythmias may occasionally occur from changes in preload as a result of this compression, so electrocardiographic (ECG) monitoring is essential.5 Several types of chest and abdominal rolls composed of fabric or gel, as well as padded square frames that have a large opening for the abdominal contents, have been routinely used to prevent this compression. Other than hemodynamic and respiratory problems, prolonged prone positioning has also been associated with significant facial and tongue edema, orbital/facial swelling, central venous retinal thrombosis, and posterior ischemic optic neuropathy, a disastrous condition that can result in permanent blindness.
5.2.3 Park-Bench Position
The park-bench position (lateral oblique position) is commonly used in skull base procedures involving the posterior fossa (Fig. 5.1). It is a semiprone lateral position with the head flexed and slightly elevated—5 to 10°. It allows both lateral and midline approaches to the posterior fossa.4 Flexion of the neck may impinge venous circulation, and obstruction might not always be recognized by examination of the external position.6 This position has less hemodynamic and respiratory effects than the full prone position, but neck flexion may be associated with brachial plexus injuries if care is not taken when assessing final position. Extreme flexion may compromise spinal cord perfusion and has been associated with quadriplegia.7 This position offers better surgical exposure and the potential advantages of improved hemostasis. However, the head elevated position brings a higher incidence of venous air embolism (VAE) and pneumocephalus.8 Open sinuses, noncollapsible bony sinuses, and large veins all heighten risk for VAE.

5.2.4 Lateral Position
The lateral position is frequently used for intracerebellar procedures involving lateral or cerebellar hemispheric lesions, tumors of the clivus, petrous ridge, and anterior and lateral foramen magnum. Typically the retromastoid, transtentorial, and transcondylar surgical approaches require this position (Fig. 5.2).

Again, the position has some significant hemodynamic and pulmonary implications. Significant V/Q mismatching takes place, albeit to far less a degree than for the prone position. Some of the disadvantages of this position include the potential for lateral popliteal nerve palsy in the dependent leg, compression of the dependent shoulder and axillary structures, and presence of the superior shoulder in the surgeon’s line of sight.4 Devices such as axillary rolls (designed to decompress dependent axillary neurovascular structures) must be correctly placed, and leg positions and bony protuberances must be padded with foam; in some instances the elbows may be wrapped to avoid ulnar neuropathy from pressure point contact at the ulnar groove. Metal edges should be isolated from the patient, and abnormal flexion or extension of the extremities should be checked for to avoid diminished blood flow and nerve injury. Padding should also be placed under the heels if supine and pillows placed between the knees to avoid peroneal and lateral thigh nerve injury.
5.2.5 Sitting Position
Of all positions used for skull base procedures, the sitting position has undergone the most scrutiny (Fig. 5.3). The theoretical advantage of the sitting position is that it allows for improved cerebral relaxation and promotes gravity drainage of blood and cerebrospinal fluid (CSF).8 The complications, however, are numerous and include hemodynamic instability, VAE with the possibility of paradoxical air embolism, pneumocephalus, quadriplegia (especially in the presence of extreme neck flexion), and compressive neuropathy.9 , 10 Another potential complication includes inadequate cerebral perfusion, but this may be balanced by a reduction in cerebral rate of metabolic oxygen consumption.11

Early physiologic studies reveal significant changes when a patient assumes the upright position. Cardiac and systemic vascular resistance are known to increase by 19% and 10%, respectively, and stroke volume and cardiac index may decrease by as much as 21% and 10%, respectively.12 In the presence of inhalational anesthetic agents, arterial hypotension may be profound, especially because these agents cause vasodilation, with venous pooling in the lower extremities, and cardiovascular depression.13 It is essential that the anesthesiologist carefully monitor systemic blood pressure as the patient assumes the sitting position. Interventions to maintain blood pressure may include fluid administration, decreasing inhalational agent concentration, compression of distal extremities, and short-term infusions of phenylephrine or other vasoactive drugs.
There is a perceived advantage of the sitting position from the point of view of the respiratory system. Ventilation is unimpeded, because diaphragmatic excursion is greater than in the horizontal position and consequently airway ventilation pressure is lower.14
It is important to keep in mind these potential complications when attempting skull base procedures in sitting positions. A vital role for the anesthesiologist who is taking care of the sitting patient is to ensure that the entire care team participates in the careful positioning of the patient. Recent reviews have gone as far as to list contraindications for using this position, including advanced age, hypertonia, chronic obstructive lung disease, and diagnosed patent foramen ovale.14
5.3 Complications during Skull Base Procedures
5.3.1 Venous Air Embolism
Venous air embolism (VAE) is one of the complication that concerns physicians most during skull base surgery. Because skull base procedures deal with venous structures that do not collapse and that are typically held open by bone, it is easy to see how venous air can be entrained via one or several of these open venous plexuses. The occurrence of VAE is especially concerning in patients who have a patent foramen ovale (present in 10–30% of the population), in which the potential for paradoxical venous air embolus is high (Fig. 5.4). Matjasko and colleagues have described four grades of VAE seen during sitting craniotomies.15 These grades (I–IV) relate to changes in precordial Doppler sounds, changes in end-tidal carbon dioxide (ETCO2) levels, ability to aspirate air, and the presence of hemodynamic instability:
Grade I: characteristic changes in Doppler sounds
Grade II: changes in the Doppler sound plus fall of end-expiratory CO2 concentration by more than 0.4%
Grade III: changes in Doppler sounds, fall in end-expiratory CO2 concentration, and aspiration of air through the atrial catheter
Grade IV: combination of preceding signs with arterial hypotension over 20% and/or arrhythmia or other pathological ECG changes
Factors that play a role in the development of VAE include a pressure gradient between the surgical field and the right atrium, the surgical technique employed, and the amount of air entrained. Moreover, many neurosurgical patients are hypovolemic, which reduces central venous pressure and further increases the risk of a VAE. The bone has been noted to be the source of VAE in 43% of all sitting craniotomies in which an air embolus has occurred. The incidence of neurosurgical skull base VAE has been noted to be 28% for sitting craniotomies, compared with 5% for supine and prone positions.16 Because many skull base resections could involve the jugular vein and cavernous or sigmoid sinuses, and considering that some positioning requires head elevation, the risk of passive air entrainment is increased. With the translabyrinthine or retrosigmoid approach, the patient is usually supine and no prophylactic measure to monitor or protect for VAE is necessary. Although VAE is frequently detected (a 72% incidence of VAE was detected by transesophageal echocardiography [TEE] in one study17), its overall morbidity is currently low, at less than 0.36%.

Monitoring for VAE has been extensively described and usually consists of one or more of the following devices: in descending order of sensitivity, TEE, precordial Doppler (in the right third to sixth intercostal space), pulmonary artery pressure, ETCO2/end-tidal nitrogen, right atrial pressure, ECG, and esophageal stethoscope.14 Although many clinicians have used a transesophageal echo for detection of VAE, the high sensitivity produces too many false positives, reducing specificity as a monitor for detection of air entrainment.18 In addition, TEE is expensive and requires that trained personnel be available for interpretation. TEE has also been associated with vocal cord paralysis from recurrent laryngeal nerve palsy after prolonged use (Table 5.1).
Recommended therapeutic measures in the event of air entrainment include bilateral compression of the jugular veins, flooding of the wound with saline on the part of the surgeon, discontinuation of nitrous oxide, aspiration of air from a central catheter, and downward tilt of the surgical table. An atrial catheter is usually placed to allow efficient aspiration of trapped air in the heart. Accordingly, it is beneficial for the right atrial catheter to be multiorificed in nature. Bunegin-Albin air aspiration catheters can be difficult to place, for the catheter tip must be in the right atrium, a position that is hard to confirm without use of radiographic imaging or ECG tracing (biphasic P-wave morphology). A trial of placement of right atrial air aspiration catheters noted that the intravenous ECG P-wave morphology that correlated with the right atrial superior vena cava junction, identified by TEE, was the largest monophasic negative P wave without any biphasic component.19 Another common practice after air entrainment is to attempt to change the patient’s position from sitting to left lateral recumbent, but this has been refuted by Geissler and colleagues,20 who noted that body position had no effect on hemodynamics, nor any on outflow obstruction. Hypotension and a decrease in coronary perfusion pressure appeared to play more of a role in explaining the cardiovascular effects of air emboli.
The role of positive end-expiratory pressure (PEEP) has also come into question. Schmitt and colleagues17 noted that when PEEP was released, there was a significant occurrence of air emboli as documented by TEE. The group postulates that a sudden decrease of moderate PEEP might decrease right atrial pressure and subsequently increase venous return from cerebral veins. This would result in an increase in air entrainment and possibly an increase in the detectable number of VAE.
5.3.2 Pneumocephalus
Another complication associated with skull base surgery is pneumocephalus, defined as the presence of intraventricular air. In a retrospective review that compared sitting, park-bench, and prone positioning, 100% of the patients in the sitting position, 73% of those in the park-bench position, and 57% of those in the prone position had evidence of intraventricular air.21 This has been attributed to the large amount of CSF drained as a result of the gravitational effect. A patient in the sitting position is subject to the effects of gravity more than in other positions. As a result, more CSF is ultimately drained, leading to the high incidence of pneumocephalus seen in sitting patients.
Not every case of intraventricular air results in tension pneumocephalus. Historically, approximately 3% of sitting posterior fossa cases were noted to have developed tension pneumocephalus.9 Postoperative care and length of surgery play a role in the development of tension pneumocephalus, but single contributing factors such as preexisting ventriculoperitoneal shunts, the use of nitrous oxide, and intraoperative diuretics do not appear to play a solitary role.21
5.3.3 Macroglossia/Facial Swelling
A rare but potentially catastrophic complication of skull base surgery includes the development of macroglossia. Several case reports describe the occurrence of macroglossia in the immediate postoperative period, but overall incidence is around 1%.22 Venous drainage of the face, tongue, larynx and orbits enters the internal jugular system, potentially kinking and leading to partial or complete obstruction of the system when the neck is maximally flexed. In the worst case scenario, this may lead to thrombosis of the internal jugular vein.23
Other theories regarding the etiology of macroglossia include arterial compression, a neurogenic event, reperfusion injury, and impaired lymphatic drainage.6 , 24 Lam and colleagues6 believe that there is a role for reperfusion injury, for many cases are not associated with cerebral swelling and edema, a condition that might be expected with presence of internal jugular venous obstruction. Obesity, neck flexion, local compression, and long surgical duration have been identified as risk factors and should be kept in mind when patients present for skull base procedures. Regardless of the specific etiology of macroglossia, careful positioning of the head and neck is essential. As a rule, we ensure a space of approximately two fingerbreadths between the mandible and clavicle to prevent venous occlusion. Leaving the patient intubated postoperatively and using steroids will protect the airway and possibly quicken resolution. In cases of severe macroglossia, tracheostomy may be necessary.
5.3.4 Cerebrovascular Complications
Fortunately cerebrovascular accidents and complications are rare during skull base procedures. Injury to the carotid artery is one of the most feared complications and may lead to stroke and other brain injuries. Carotid repair may be required and may include saphenous vein bypass graft from the extracranial carotid artery to the petrous carotid artery and superficial temporal to middle cerebral artery bypass.25 Usually, when the carotid artery is affected by tumor, a balloon-occlusion test will be performed preoperatively to help identify those patients who would tolerate the sacrifice of the carotid artery if it were to become necessary during the procedure.
“Blowout injury,” another carotid vascular complication, is caused when the carotid artery is inadvertently lacerated. Blood loss may be brisk at the time of the injury, and interventions include packing and urgent angiography with balloon occlusion or vascular stenting.26 Delayed blowout injury can also occur if the carotid artery is exposed in the nasopharynx, but this might be preventable through muscle flap coverage.25
Vasospasm has also been reported and may result in stroke. It tends to be seen in younger patients and is thought to be caused by a myogenic reaction in the vessel wall. Vasospasm may result from arterial contact with fresh blood or arterial traction. Treatment usually consists of topical vasodilators such as papaverine, although systemic drugs may also have a role.27
5.3.5 Arrhythmias
Because many skull base procedures are in the area of the trigeminal and vagus nerves, as well as the brainstem, arrhythmias during these surgical procedures may be common. Direct stimulation of the vagus may lead to negative chronotropy and inotropy manifested as sinus bradycardia, bradycardia terminating in asystole, asystole with no bradycardia, and arterial hypotension. When the trigeminal nerve is involved, sensory nerve endings send signals to the sensory nuclei of the Gasserian ganglion. These signals ultimately continue along the short internuncial nerve fibers to connect with the motor nuclei efferent pathway of the vagus nerve, producing a cardioinhibitory effect.28 In this situation, the anesthesiologist should alert the surgeon and may request that the surgeon release traction or choose pharmacologic intervention with a vagolytic substance such as glycopyrrolate or atropine. Atropine, because of its quick onset, may be the drug of choice, but its duration of action is shorter than that of glycopyrrolate. Over time, the reflex tends to decrease in intensity.
This trigeminocardiac reflex (TCR) has garnered much interest in recent years. There seems to be a relationship between light anesthesia (cerebral state index [CSI] > 60) and TCR occurrence.29 The severity of the response with larger mean arterial pressure (MAP) drops and occurrence of asystole suggests a more severe reflex with a light plane of anesthesia. The best-known anesthetic risk factors for TCR are the fast-acting opioids, such as fentanyl.30 The effect of propofol, an anesthetic that is currently often used in skull base surgery, has not been fully analyzed. However, studies examining its excitatory postsynaptic potential on cardiovascular neurons in the nucleus ambiguous showed no relative change.31 At present, a trend toward higher anesthetic doses’ having an inhibiting effect on the TCR seems to be real. The prevalence of TCR in a CSI > 60 group was consistently higher than in a CSI 40 to 60 group and a CSI < 40 (deep anesthesia) group. Furthermore, there is a strong trend for light anesthesia to be a risk factor for a more intense asystolic reflex as compared with deeper anesthetic levels. There also seems to be a significant correlation between propofol and the occurrence and intensity of TCR. Evidence suggests that propofol has an inhibitory effect on either the afferent trigeminal neurons or the efferent cardiac vagal neurons.31 The use of propofol infusions as part of the anesthetic technique will help reduce the incidence and severity of the TCR while producing better anesthetic conditions for other monitoring modalities such as somatosensory evoked potentials (SSEPs) and motor evoked potentials, if they are used.
In most cases, an anticholinergic agent such as glycopyrrate—or, more likely, atropine—will be used to treat the effects of TCR. However, atropine does not totally prevent TCR.32 In addition, atropine itself can cause severe arrhythmia. Some practitioners now recommend using low-dose epinephrine 10 to 50 µg IV to stabilize cardiovascular function after TCR.
If skull base surgery involves resection of a glomus jugulare or vagale tumor, then one must be aware of the tumor’s catecholamine-secreting potential. Glomus jugulare tumors may be considered arteriovenous malformations, may be giant in size, and may be associated with multiple paragangliomas. The incidence of catecholamine secretion is around 4% and can be associated with tachyarrhythmias, hypertension, sweating, myocardial infarction, and cardiovascular collapse, which reflects catecholamine excess. The anesthesiologist should be prepared to treat any hypertensive crisis arising from manipulation of a catecholamine-secreting tumor. Appropriate invasive arterial monitoring is essential, and use of fast- and short-acting vasodilators, such as nitroglycerin, nitroprusside, and, to a lesser extent, phentolamine, is essential. The resection of glomus jugulare tumors may also result in new cranial nerve (CN) injuries, with the highest being of CNs VII and IX.33
5.3.6 Blood Loss
Blood loss during resection of skull base tumors may be significant, and the taking of appropriate preoperative measures is essential. Many such tumors are highly vascular, and preoperative assessment is necessary to delineate involvement of the cavernous sinus and jugular bulb. Notably, meningiomas have been shown to produce tissue plasminogen activator, which may lead to increased fibrinolysis during resection.34
Preoperative embolization of feeding vessels may lead to decreased blood loss via a decrease in blood flow and pressure within the tumor.25 Recent studies have evaluated the use of tranexamic acid, a synthetic derivative of the amino acid, lysine, that reversibly blocks lysine-binding sites on plasminogen molecules, preventing conversion to plasmin and inhibiting fibrin clot dissolution.35 Tranexamic acid may be particularly advantageous in skull base procedures, because even small amounts of bleeding near eloquent cranial base structures could be problematic. The drug has a favorable safety profile but has been noted to increase the risk of cerebral ischemia.36 It has also been shown to increase the risk of seizures when given in high doses.37 In several studies, its use with skull base surgery reduced the frequency of transfusion of allogenic blood products, with no apparent increase in seizures or thrombotic complications. The use of this drug may prevent some of the complications of administering large amounts of blood products, including transfusion-associated circulatory overload and transfusion-associated lung injury.
5.3.7 Peripheral Nerve/Cranial Nerve Injuries
Protection from peripheral nerve injuries is of major concern during skull base procedures. All positions, from supine to seated, have at one time or another been associated with nerve injuries. Varying degrees of injury have been noted, with Seddon’s classification describing three broad classifications of injury: neurapraxia, axonotmesis, and neurotmesis. Neurapraxia is a mild insult that results in conduction failure across an affected segment; this reversible injury tends to be that most seen during surgical procedures. Axonotmesis occurs when the axon is physically disrupted but the epineurium and perineurium are preserved; recovery depends on the speed of neural regeneration. The worst injuries are those in which neurotmesis has taken place: there is complete disruption of the nerve and support structures, and the prognosis for recovery is exceedingly poor.38
Although brachial plexus injuries have been often described due to the contralateral rotation of the head and traction of the ipsilateral shoulder downward,39 other injuries have been noted, including common peroneal nerve leading to foot drop.40 Ulnar nerve injuries may also occur, but the complete etiology of their occurrence has yet to be determined. The role of abnormal extension, rotation, obesity, and preexisting disease states, such as diabetes mellitus, have been mentioned as possible contributing factors for ulnar nerve injury. Whatever the etiology, meticulous attention to appropriate intraoperative padding and awareness of the existence of preexisting neurological defects is essential when taking care of these patients.
There has been an increased awareness of neurologic injury to the upper extremities after positioning for these surgical procedures. SSEPs are a proven method of monitoring the spinal cord and brain to prevent neurologic injury and have also been used to monitor the arms and brachial plexus during skull base procedures in which the head is turned contralaterally and the ipsilateral shoulder is pushed down to open the approach for retrosigmoid/translabyrinthine craniotomy. Many studies have found this monitoring modality to be a valid intraoperative tool for monitoring positionally related peripheral nerve injuries in real time.41 , 42 It offers 78% sensitivity for detecting upper extremity sensory deficits and 100% sensitivity for detecting combined sensory and motor deficits, with a 98% specificity for predicting normal postoperative function.43 SSEPs are obtained after the patient is asleep and in a neutral position and are used as a baseline for another set of SSEP waveforms obtained after positioning. If major changes occur, the positioning is readjusted, especially as concerns the affected extremity. Another set of SSEP values is determined; if improved, the surgery proceeds.
It is plausible that nerve compression and ischemia can be further complicated and more often observed in diabetic patients or those with large body habitus, especially because the most severe injuries are noted in larger individuals. We have extensively studied the technique to monitor for nerve injury during skull base procedures, and based on our results, increased patient body mass index appears to correlate with an increased likelihood of experiencing postoperative peripheral nerve injury due to malpositioning.44 We believe that this monitoring technique should be used for routine assessment of the brachial plexus during these procedures or at least obtained for high-risk obese patients.
5.4 Monitoring and Anesthesia
Monitoring for skull base procedures depends on the type of procedure to be performed, the vascular and nerve structures involved, and the position in which the patient will be placed for the surgery. In all instances the patient will have standard routine monitoring such as ECG, noninvasive blood pressure, pulse oximetry, capnography, and temperature. Other monitors are added as the complexity, blood loss, surgical trauma, and comorbidities of the patient are factored in. Monitoring for skull base procedures must ensure adequate central nervous system perfusion, maintenance of cardiovascular stability, and the integrity of the neurologic pathways that are being manipulated. Arterial line placement is standard for most intracranial and extracranial procedures of the skull base. Invasive blood pressure monitoring allows closer control of blood pressure and better titration of hyperventilation and blood pH. In addition, hemoglobin levels and electrolyte abnormalities can be easily detected by following serial arterial blood samples obtained from this catheter. Central access with either large-bore single lumen or double lumen catheters is contingent on the length of the surgery, anticipated blood loss, need for estimation of central vascular volume, and position of the patient.
Depending on tumor type and location, neurophysiologic monitoring may also be employed to detect disruption of neural tracts or trauma to CNs that may be near the site of surgery.
Cranial nerve monitoring has markedly decreased postoperative morbidity after skull base surgery. Electromyography (EMG) of the facial, vagus, or trigeminal nerve is used during surgical resection to identify the nerve and preserve neurologic integrity, especially if the nerve is surrounded by a tumor.45 EMG of CNs provides early recognition of surgical trauma, facilitates tumor excision, identifies nerve dysfunction, and confirms nerve function after the tumor is removed. At any given level of neuromuscular blockade, a facial muscle response is more resistant to neuromuscular blocking agents than a peripheral muscle.46 This is due to larger motor unit size and the increased number of neuromuscular junctions in the facial muscles. Several studies have demonstrated that neuromuscular blockade, titrated to a T1 of 25%, still allows adequate response from compound motor action potentials of facial muscles to adequately monitor nerve function.47 Nerve irritation and tumor infiltration, however, can lead to reduced or blocked conduction. In addition, external or mechanical noise artifacts could mask muscular contraction, and if high-dose inhalational agents are used, muscle activity with nerve stimulation can be further reduced.
If muscle relaxants must be used to facilitate surgical resection, alternative methods for monitoring the facial nerve may be used. The first method stimulates the nerve at the stylomastoid foramen.48 Antidromic responses are recorded in the operative field. However, this method is awkward to use and does not provide the information obtained by continuous recording. Nerve action potentials can also be recorded at the stylomastoid junction, but this technique is an evoked response. There is no audible feedback compared with EMG and no information concerning this method’s sensitivity in detecting injury.
The final method for determining facial nerve integrity in the presence of muscle relaxants is the brainstem facial evoked response49; this nerve monitoring method is based on cross-auricular responses to sound that controls ear movement. The facial nerve response is recorded at the mastoid after sound stimulation of the contralateral ear. This technique is technically challenging because of the need for digital computer filtering.
EMG monitoring is important for improved outcomes after skull base surgery. The test relies on measurement of compound muscle action potential (CMAP) generated either by facial muscles or by those of the oropharynx or vocal cords. The motor unit potentials, when summed together, result in the measured CMAP. An adequate assessment of nerve conduction with EMG requires stimulation proximal to the potential site of injury. Nerves suffering from mild to moderate trauma will exhibit reductions in amplitude with increasing amounts of current to elicit a response. After ensuring careful electrode placement for facial nerve electromyographic monitoring (FNEMG) or proper ET tube placement with the nerve integrity monitoring ET tube, both nerves VII and X can be monitored. Prass and Luders described two types of CMAP activity depending on the type of nerve irritation.50 The first type is the burst potential, which consists of a polyphasic response due to activation of multiple motor units. These are caused by direct contact of the nerve with surgical instruments and are fatigable with repeated nerve contact. The second type of continuous free-running CMAP is a train potential. This could last seconds to minutes and is generated by multiple asynchronous responses from different motor units. Train potentials may be caused by mechanical injury (pressure or stretch) or thermal changes to the nerves. With greater injury to the nerve, a greater intensity and longer duration of nerve potential will be evident. Thermal injury from electrocautery or laser may only become evident on EMG in a delayed manner and may be noted as a gradual increase in baseline but may also be associated with electrical silence. Other causes of nontraumatic train potentials include drill vibration energy transmission to the nerve, temperature irritation from hot or cold irrigants, and hypertonic saline solutions. Even aspiration of CSF from the cerebellopontine angle could produce a drop in temperature, triggering a train response.
Besides assessing for injury, nerve stimulation EMG can be used to identify the nerve within the tumor to help salvage facial function and nerve integrity during the resection. The stimulating probe is touched to or near the nerve, generating a true EMG-stimulated machine gun–sounding response (Video 5.1, Video 5.2, and Video 5.3). Stimulating current levels are normally adjusted from 0.05 to 2 mA. False positives can occur with stimulation-triggered EMG from a phenomenon known as current jump, which happens when volume conduction of current through nearby tissue leads to nerve stimulation. False negative errors can also occur when the presence of CSF or blood in the field produces electrical current shunting away from the nerve of interest. The use of intraoperative EMG has improved outcomes, reducing both facial nerve and vagal injury and reducing morbidity after skull base surgery.
The ability to monitor the adequacy of an anesthetic is important, because it aids in the titration of anesthetic drugs during maintenance periods and, in particular, will prevent movement during stimulation, which can be particularly problematic in unparalyzed patients undergoing skull base procedures. Some have noted bispectral index (BIS) monitoring as an important modality to monitor depth of anesthesia. However, BIS monitors higher-level brain activity and hypnosis and might not be an effective monitor with which to predict post incisional or other movement under anesthesia. FNEMG is usually performed during many skull base procedures, because it helps identify the nerve and reduces iatrogenic injury during tumor resection. We have also noted FNEMG to be an effective monitor of anesthetic depth and a good monitor for predicting and preventing patient movements.51 FNEMG was highly reliable as a monitor with which to predict movement, having a negative predictive value of 95%. Lack of FNEMG activity is an assurance that the patient will not move. We continuously use FNEMG as a monitor of anesthetic depth during skull base procedures and believe that the use of an opioid infusion as part of the anesthetic technique will prevent movement in nonparalyzed patients undergoing skull base resection.
Brainstem auditory evoked potentials and SSEP monitoring may also be used during resection of skull base tumors, especially if there is possible vascular compromise or ischemia due to temporary occlusion of vascular structures or manipulation of the brainstem. In general, anesthetic effects on Brainstem Auditory Evoked Responses (BAERs) are not dramatic. Slow shifts may be seen as the concentration of inhalational agents increase. Improvement or preservation of hearing may also be accomplished with the use of BAERs. Since these recordings are of small amplitude, thousands of responses must be recorded to acquire an adequate average. Frequently the responses are abnormal and smaller than normal due to the effects of the tumor. Also the time interval required to acquire sufficient responses may reduce the sensitivity of this technique in determining neural injury during tumor removal. Short-latency BAERs are usually resistant to both intravenous and inhalational agents. Increasing blood levels of barbiturates and ketamine will increase interpeak latency. In fact, another hypnotic agent propofol given at 2 mg/kg bolus, followed by an infusion, will increase the latency without changes in amplitude.52 Inhalational agents such as isoflurane, sevoflurane, and desflurane also increase the latency of the waveform without an appreciable change in amplitude. Conversely, midlatency AEPs are predictably affected in a dose-dependent manner by an increase in latency with a predictable decrease in amplitude.53 , 54 Hypercapnia does not change BAERs, but severe hypoxia will depress waveform amplitude.55 Body temperature will also affect BAERs, with reduced temperature prolonging latency of the observed waveform.56 In most instances in which changes in waveform resulted from anesthetics, the observations will occur bilaterally. Unilateral decreases in waveform would be indicative of nerve damage secondary to surgical manipulation.
Finally, somatosensory evoked responses may also be used to assess the integrity of the brainstem and other subcortical structures during surgical techniques involving the skull base. SSEP represents reproducible electrical activity of cortical and subcortical structures time-locked to a peripheral nerve stimulus. SSEPs can assess the sensory system from the peripheral nerve through the spinal cord and brainstem to the cerebral cortex. A decrease in amplitude of 50% or greater and an increase in latency of 10% or greater constitutes a significant change that should be investigated.57 Because SSEPs have a central component to their measurement, they are very sensitive to the effects of anesthetic agents. All volatile anesthetics produce a dose-dependent increase in SSEP latency, an increase in central conduction time, and a decrease in amplitude (Table 5.2).58 Satisfactory monitoring of early cortical SSEPs is possible with 0.5 to 1.0 minimum alveolar concentration (MAC) isoflurane, desflurane, or sevoflurane, the latter two of which agents permit slightly higher concentrations with satisfactory results.
How volatile anesthetics differ quantitatively is still not entirely known, but sevoflurane and desflurane are associated with less amplitude reduction than isoflurane at a MAC range of 0.7 to 1.3.59 Intravenous anesthetics generally affect SSEPs less than inhaled anesthetics do. SSEP waveforms are preserved even at high doses of narcotics and barbiturates. Intravenous agents only modestly affect early and intermediate SSEP components. Most authors report clinically unimportant changes in SSEP latency and amplitude with the administration of opioids given in either anesthetic or analgesic doses. This makes opioids useful as part of the anesthetic when intraoperative physiologic monitoring is used. Propofol affects the SSEP waveform by increasing latency by 8% at a dose of 2.5 mg/kg while having no effect on amplitudes.60 Total intravenous anesthesia with propofol and sufentanil prolonged cortical latency 10 to 15% and reduced the amplitude by 50%. However, SSEP waveforms stabilized and after 30 minutes were compatible with intraoperative monitoring.61 Propofol affects synaptic transmission more than axonal conduction. When used as a sedative hypnotic along with opioids, propofol reduces SSEP amplitudes less than N2O or midazolam. Anesthetics that result in latency prolongation or amplitude depression may confuse the interpretation of SSEP changes and potentially risk either not detecting a critical event or providing excessive false negative interpretations.
In some instances, etomidate or ketamine can be added to the anesthetic regimen to facilitate SSEP waveform measurement. Both can dramatically increase cortical amplitude up to 400% above preinduction baseline in some patients.62 Subcortical amplitude is reduced by 50%.
The effects of anesthesia on evoked potentials can be greater in neurologically impaired patients than in those without preoperative deficits, so the anesthetic regimen must be adjusted to carefully limit the concentration of volatile anesthetics to less than 1 MAC or to avoid N2O. If neurophysiologic monitoring will include electrocochleography or SSEP, volatile anesthetics with N2O should be limited to 0.5 MAC concentrations. Without N2O, 1-MAC concentrations may be used.
The use of continuous infusions of intravenous anesthetics (propofol) and opioids with low concentrations of background inhalational anesthetics is ideal and recommended for intraoperative neurophysiologic monitoring during skull base procedures. Inhalational agents such as sevoflurane and desflurane have low blood gas partition coefficients and reduced fat solubility. These agents produce rapid induction and emergence with minimal accumulation of anesthetic, even after prolonged anesthesia. In addition, short-acting opioids such as remifentanil are extremely potent and have minimal accumulation when given as an infusion. The half-life is 3 to 8 minutes, and metabolism is produced by nonspecific esterases.
Remifentanil infusions for large skull base procedures may not be an ideal option. The drug produces a rapid emergence from anesthesia with minimal hangover effects and can be easily titrated to reduce acute hemodynamic responses to intense stimulation. It does not accumulate and is ideal for long procedures, especially in patients who have renal compromise. Remifentanil has also been noted to be an ideal agent with which to lessen the risk of movement in the absence of muscle relaxation, a state that occurs in many instances during skull base surgery. Remifentanil, however, also increases the probability of bradycardia, especially at higher doses. This can be problematic during skull base procedures in which the risk of bradycardia or asystole is high from possible TCR. The other significant issue with the use of remifentanil is blood pressure management during the early recovery period. Many patients experience hypertension and tachycardia after discontinuation of remifentanil, most likely due to early analgesic requirements: the half-life of remifentanil is short, and patients go from a state of intense analgesia to no analgesic effect within 5 minutes. It is likely that the use of remifentanil-based anesthesia will result in lower incidence of emergence hypertension if analgesia for early recovery is provided before emergence.63
An advantage to remifentanil is that little or no interaction between residual effects of maintenance opioids and opioid administrated for emergence would be expected. In addition, remifentanil has been associated with hyperalgesia after discontinuation. This may complicate postoperative pain management, though the overall incidence of this complication is unclear. Although we still use remifentanil for some skull base procedures, we do not use the drug when there might be a higher risk of postoperative bleeding due to resections of large tumors that have large vascular beds or feeding vessels. In these situations, longer-acting opioids (fentanyl or sufentanil) are used as background infusions to supplement the inhalational agent. The anesthetic technique that produces the best operating conditions for surgery and CN monitoring uses an anesthetic induction in which propofol and fentanyl are coupled with a short-acting muscle relaxant to facilitate intubation. Maintenance anesthesia is provided with low-dose desflurane or sevoflurane administered in a 50:50 air/O2 mixture with a background infusion of either fentanyl 2 µg/kg/hour or remifentanil 0.25 to 0.35 µg/kg/minute. No further muscle relaxants are administered.
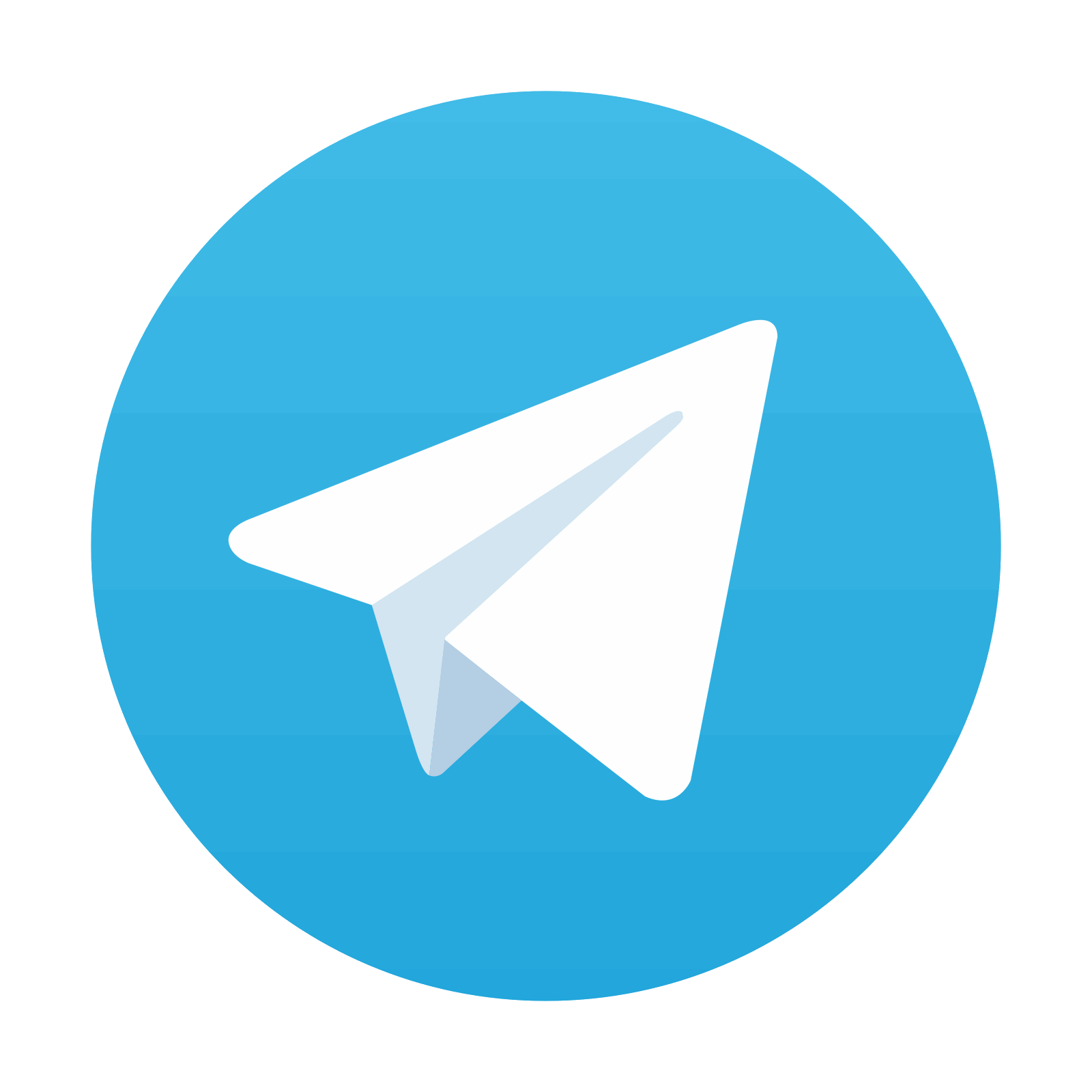
Stay updated, free articles. Join our Telegram channel

Full access? Get Clinical Tree
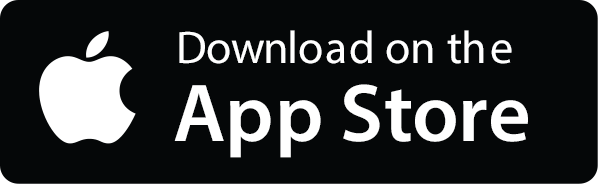
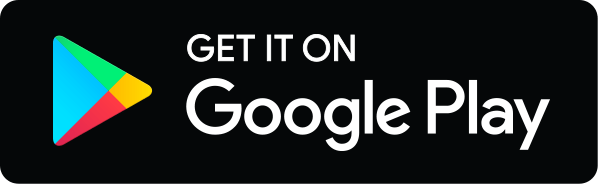