5 Biomechanics of the Craniovertebral Junction
Stabilization of the craniocervical junction presents a unique biomechanical challenge. The combination of variable anatomy, complex pathologies, complicated biomechanics, and imperfect internal fixators makes in-depth understanding of this region mandatory for successful surgical management. This chapter focuses on biomechanical considerations for stabilization. The reader should be mindful, however, that optimal outcome also depends on other facets of treatment, such as indications, perioperative management, arthrodesis, and adequate decompression of neural elements.
Relevant Anatomy
Occipital Bone
The occipital bone comprises the most caudal portion of the calvarium ( Fig. 5.1 ). The broad, convex portion of the occipital bone below the superior nuchal line is referred to as the suboccipital region. Midway between the superior nuchal line and the foramen magnum is the inferior nuchal line, bisected by the external occipital crest. This region is most frequently used as a point of fixation for occipitocervical constructs. The center of the intracranial transverse sulcus, containing the transverse sinus, is a mean of only ∼0.9 mm above the superior nuchal line.1 The superior nuchal line therefore represents the uppermost landmark of the area potentially available for fixation. In addition, fixators placed at or above this area tend to be quite prominent and pose potential problems with wound healing and skin breakdown. Below the superior nuchal line, the suboccipital bone varies widely in thickness. In the midline, the presence of an internal occipital crest, corresponding to the external occipital crest superficially, contributes to a mean thickness of 13.8 mm at the external occipital protuberance and 8.3 mm at the level of the inferior nuchal line. More laterally, the occipital bone may be considerably thinner, with a mean thickness of only 5.7 mm halfway between the foramen magnum and inferior nuchal line.1

Relevant to the present discussion, the occipital bone forms the occipital condyles. The paired occipital condyles articulate with the superior articular surfaces of the atlas. The mean anteroposterior length of the ovoid occipital condyle is 23.6 mm, and the mean width and height are 10.6 and 9.2 mm, respectively. The mean anterior and posterior intracondylar distances are 21.0 and 41.6 mm, respectively,2 emphasizing the axial plane anteroposterior divergence of these structures. Variations in the shape of the occipital condyle are common. In 20% of specimens, the occipital condyles protrude into the foramen magnum. The intracranial orifice of the hypoglossal foramen is found at the junction of the second and third quarter of the occipital condyle in the majority of specimens.2
Atlas (C1)
The first cervical vertebra, or atlas, articulates with the occipital condyles above and the axis below via the odontoid process and the paired atlantoaxial joints. This unique, vaguely ring-shaped vertebra neither possesses a true vertebral body nor articulates with an intervertebral disk. The atlas has a mean anteroposterior and lateral diameter of 45.8 and 78.6 mm, respectively.3 The mean thicknesses of the anterior and posterior rings are 6.4 and 8.0 mm, respectively. Relevant to screw-based methods of fixation to the atlas, the width and height of the C1 lateral mass are 11.6 and 12.7 mm, respectively. The mean distance from the midline to the inner wall of the C1 lateral mass is 14.2 mm. The mean distance to the outer wall is 23.0 mm.4
Axis (C2)
The second cervical vertebra, or axis, articulates with the atlas above and the third cervical vertebra below. It possesses a unique projection, the odontoid process, which is critically important in constraining normal movements at the craniocervical junction. The axis has a mean total height of 39.9 mm and a mean odontoid height of 16.6 mm. At the tip, the odontoid has a mean diameter of 11.2 mm in the sagittal plane and 10.8 mm in the coronal plane. Both of these dimensions are decreased closer to the base, or isthmus. The odontoid is angled posteri-orly a mean of 16.6°. Significant variation in this angle is seen from patient to patient. The C2 pedicle has a mean height of 9.4 mm and a mean width of 8.0 mm.5 Pedicles are medially angled, with a mean angulation of 39°.6 The axis–C3 articulation is similar to other articulations in the subaxial cervical spine.
Ligaments
Transverse Ligament
The transverse ligament attaches to the dorsal surface of the odontoid and the lateral masses of the atlas, restricting flexion as well as anterior displacement of the atlas.7 It is frequently involved in pathological processes involving the craniocervical junction. This ligament has a mean length of 21.9 mm.8
Alar Ligament
The alar ligament attaches to the ventral and lateral surfaces of the odontoid process and occipital condyles, frequently with a secondary attachment to the lateral masses of the atlas. It restrains axial rotation,7 side bending,9 and flexion/extension10 of the upper cervical spine. This ligament has a mean length of 10.3 mm and is oriented 70° from the sagittal plane.8 It is elliptical in cross section, 3.6 by 6 mm.9 Rupture of the alar ligaments appears to be less common than failure of the bony attachments.11
Failure of the alar ligament results in significant alterations in kinematics. Unilateral alar ligament transection results in an increase in the neutral zone at the occipital–C1 articulation in lateral bending on the contralateral side, without an increase in the range of motion (ROM). In addition, transection of the alar ligament increases occipital–C1 flexion but not extension. Unilateral and bilateral alar ligament transaction results in significant increases in flexion and extension at C1–C2.10
Capsular Ligaments
The C1–C2 capsular ligaments function primarily in axial rotation. In the presence of intact transverse and alar ligaments, unilateral transection of the capsular ligament results in increased ROM in rotation on the opposite side. It has been theorized, however, that damage to one or both capsular ligaments is not sufficient to produce C1–C2 dislocation.12
Kinematic Biomechanics
Occiput–C1
The kinematics of the occiput–C1 segment are determined largely by bony elements. Sagittal plane rotation is the primary movement occurring at the occiput–C1 articulation. Approximately 23.0 to 24.5° of rotation is allowed, limited by impingement of the tip of the dens on the foramen magnum in flexion and tension on the tectorial membrane in extension.13,14
Under normal circumstances, axial plane rotation and coronal plane bending are both < 10° per side, both resisted by the occipital–C1 articulation and the alar ligament.13,14 Axial rotation at the occiput–C1 is associated with up to 10° of lateral bending in the same direction as axial rotation.15 Translational movements at the occipital–C1 articulation are reported to be minimal, though sagittal plane rotation is associated with sagittal plane translation, whereas axial plane rotation is associated with lateral translation.16 The instantaneous axis of rotation in axial rotation is located anterior of the foramen magnum.15
C1–C2
The kinematics of the C1–C2 segment are determined largely by ligamentous elements. Axial plane rotation is the primary movement occurring at the C1–C2 articulation. Approximately 23.3 to 38.9° of rotation per side are allowed,13,14 limited by the C1–C2 articulation, ipsilateral transverse ligament, contralateral alar ligament,9 and capsular ligaments. Approximately 77% of cervical axial rotation occurs at the C1–C2 segment, with only 4% occurring at the occiput–C1. Axial rotation at C1–C2 is associated with up to 11° of lateral bending in the opposite direction. Interestingly, axial rotation at C1–C2 is also associated with opposite direction axial rotation (though of less magnitude) at the occiput–C1. The instantaneous axis of rotation in axial rotation is located in the central portion of the dens at C1–C2.15 Sagittal plane rotation is limited to 10.1 to 22.4°13,14 by the transverse ligament in flexion,15 tectorial membrane, and bony anatomy of the C1–C2 articulation.17 The instantaneous axis of x-plane rotation at C1–C2 has been observed to lie near the posterior cortex of the odontoid, midway between the base and tip.16 Lateral bending at C1–C2, other than that associated with axial rotation, is limited to 6.8°,14 primarily by the alar ligament.9
Anteroposterior translation at C1–C2 is frequently found in pathological states. Most frequently, this is assessed by measurement of the atlantodens interval on plain radiographs. Although it is generally accepted that an atlantodens interval ≤ 3 mm is normal,16,18 a computed tomography study by Rojas et al. suggests that 95% of normal individuals have an atlantodens interval ≤ 2 mm.19 Anterior translation is resisted by the transverse ligament,19 with lesser contributions from the alar ligaments, accessory atlantoaxial ligaments, and capsular ligaments.17 Posterior translation is resisted by abutment of the dens on the arch of C1. Translation in other planes is minimal under normal circumstances.
Although overall cervical ROM tends to decrease with age, axial and sagittal plane rotation at C1–C2 tends to remain the same or increase slightly with age.20
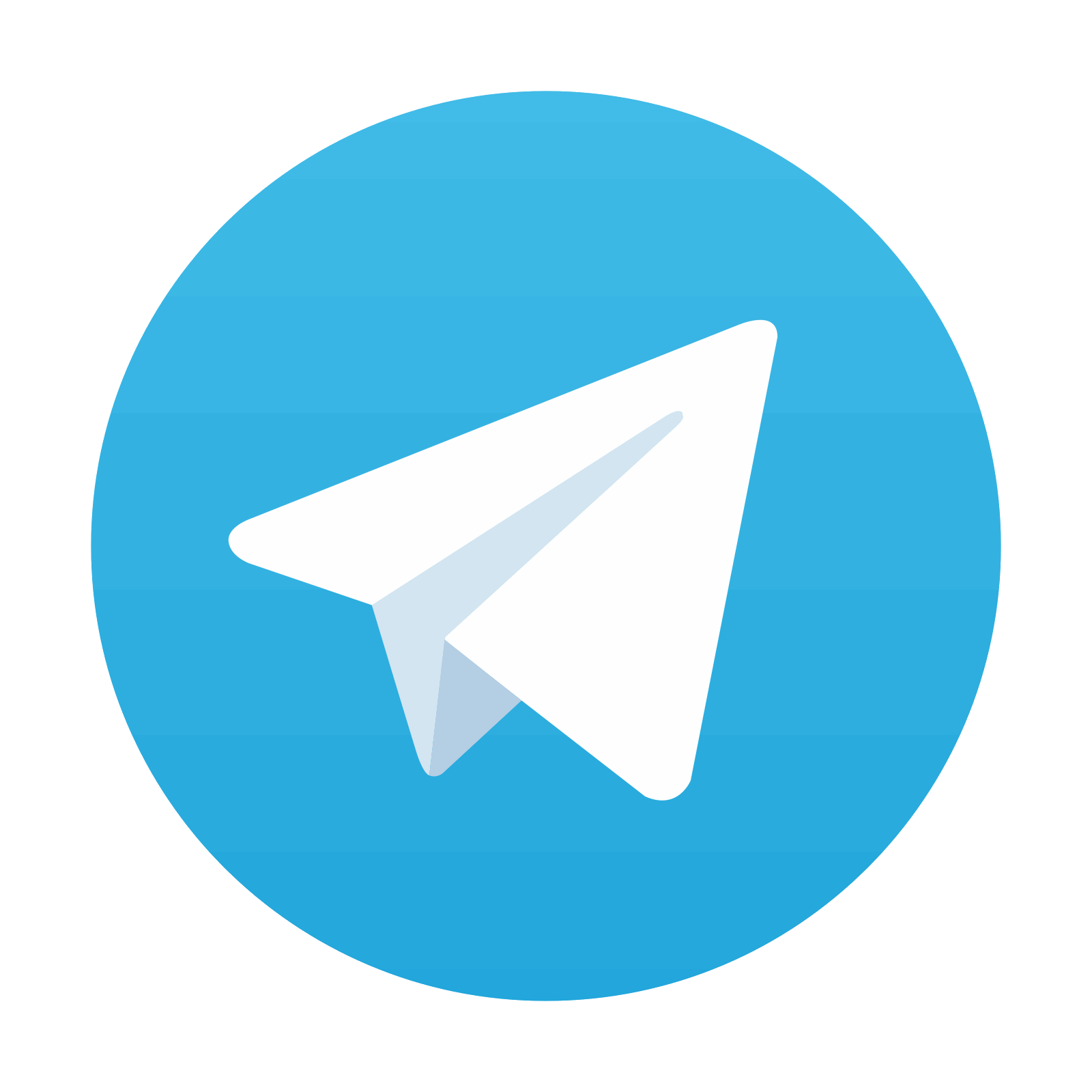
Stay updated, free articles. Join our Telegram channel

Full access? Get Clinical Tree
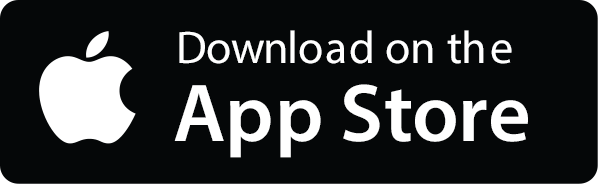
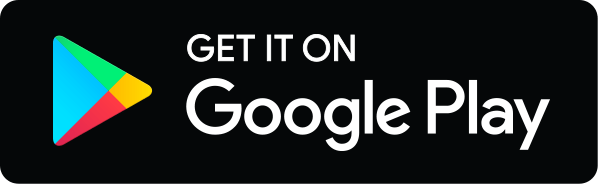
