5 Degenerative and Inflammatory Diseases of the Spine
The management of degenerative and inflammatory spine diseases is complex. Alterations of both bony and ligamentous spinal integrity are the predominant etiologic factors associated with such degeneration. The degenerative and inflammatory spine diseases presented in this chapter include primary degenerative diseases of the spine (e.g., spondylosis and Scheuermann’s disease), as well as inflammatory diseases of the spine (e.g., rheumatoid arthritis, ankylosing spondylitis, ossification of the posterior longitudinal ligament [OPLL], ankylosing hyperostosis, and related processes). This chapter focuses on the biomechanics of the cervical, thoracic, and lumbar regions as they are affected by these various pathologies. It, however, also obligatorily focuses on the clinical effects of the disease process and its biomechanical alterations. In this latter regard, the differential diagnosis and management of degenerative disease of the spine is not specifically addressed in this chapter.
5.1 Pathogenesis
Degenerative and inflammatory spinal disorders involve the disc interspace, facet joints, and intraspinal and paraspinal tissues. Degenerative changes of the intervertebral disc are typically portrayed by one or a combination of four imaging findings: (1) loss of disc interspace height, (2) irregularities in the disc endplate, (3) sclerosis of the disc interspace in the region of the endplates, and (4) osteophyte formation (Fig. 5.1). Soft tissue proliferation may accompany this process as an associated phenomenon or may be a primary process. Degenerative disc disease is defined by Kramer as biomechanical and pathological conditions of the intervertebral segment caused by degeneration, inflammation, or infection.1 Like the changes associated with disc interspace degeneration, facet joint degenerative changes are often associated with increased laxity of movement. As the degenerative process proceeds, however, an element of stability is often conferred. This is often referred to as the spine “restabilization” process. Restabilization results from a stiffening of the spine caused by one or more of the four previously mentioned processes. Hence, motion segment stiffness tends to decrease during the early phase of disc degeneration. It, however, increases as the degenerative process continues.2

Intraspinous and paraspinous tissue inflammation, calcification, and hypertrophy are seen with spondylosis, rheumatoid arthritis (e.g., bursa inflammation, pannus formation), and OPLL (e.g., calcification and hypertrophy of the posterior longitudinal ligament). Ankylosing spondylitis and related disesases are the only inflammatory or degenerative diseases associated with increased stability via diminished motion secondary to ankylosis (fusion).
The pathogenesis of degenerative disc disease varies according to the underlying disease process. Fundamentally, aberrant physiological responses to stresses placed upon the spine, and accelerated deterioration of the integrity of spinal elements, underlie the pathological process regardless of the disease entity or region of the spine involved. Before the degenerative process and accompanying pathology can be fully appreciated, the normal physiological processes associated with the disc interspace and related structures must be appreciated. This process is complex and multifactorial.3–13 It can be altered or potentially retarded by surgical intervention with fusion and potentially by medical means.
Gene therapy may play a management role in the future.14 In fact, hereditary influence and genetic risk factors for the acceleration of the spondylotic process have identified.15–17 Since juvenile degenerative disc disease is uncommon, but not rare,18 it is conceivable that hereditary and genetic influences prevail in this subset of the degenerative disc disease population.
5.2 Anatomy and Physiology of the Disc Interspace1,19
The disc interspaces account for approximately 20 percent of the height of the spine. The disc consists of an outer annulus fibrosus and an inner nucleus pulposus. It is bordered rostrally and caudally by a cartilaginous plate (endplate). The latter is part of the vertebral body and is composed of hyaline cartilage. The medullary bone of the vertebral body is connected to the endplate and provides it, as well as the disc proper, with nutrients via diffusion through fine pores (laminae cribosae).
The annulus fibrosus is composed of laminated bands of fibrous tissue (predominantly collagen) oriented in opposite directions, with consecutive layers situated in an alternating manner at approximately a 30 degree angle from the disc interspace. This 30 degree relationship of the annular fibers to the disc interspace provides a greater resistance to tension (rotation) than to bending. In fact, the rotation limiting effect is enhanced by distraction of the disc interspace (Fig. 5.2). This may have significant implications regarding torsional instability and mechanical pain. The inner bands of the annulus are attached to the endplate, whereas the marginal zone is attached to the ring epiphysis of the vertebral body and the osseous tissue of the vertebral body. These latter attachments (Sharpey fibers) are stronger than the more medial (inner) attachments to the cartilaginous plate. The annulus fibrosus is stronger and more abundant ventrally and laterally than dorsally. In fact, in youth the ventral annulus fibrosus merges into nucleus fibrosus. The fact that the dorsal fibers of the annulus fibrosus are weaker contributes to the manifestations of the disc degeneration process.

The nucleus pulposus, a remnant of the notochord, is located in the dorsal portion of the intervertebral disc. It consists of reticular bands of closely packed nuclei surrounded by a liquid mucoid ground substance. It has been implicated as a source of nerve root compression, as well as inflammatory irritation.20 The water content of the nucleus pulposus decreases from about 90% at birth to about 70% by age 70. The water, however, is not free. It is reversibly bound to macromolecules via their intense hydroscopic properties. In fact, the water content changes from morning to afternoon, which implies changes in response to weight-bearing.21 In fact, pressure-dependent fluid movement in and out of the intervertebral disc leads to measurable changes in a person’s height from the awakening supine position to the late afternoon erect position. Multiple authors have observed this fact, which is summarized nicely by Kramer.1
The latter point implies that water escapes and enters the disc through a semipermeable membrane. Other small molecules, such as waste products and nutrients, must also pass through this membrane. The changes in the water content of the disc in response to weight-bearing imply a hydrostatic pressure effect on disc interspace physiology. The hydrostatic pressure within the intervertebral disc in the erect position is many times greater than that within surrounding tissue. For the disc to retain water, fluid movement must occur against this very steep pressure gradient. The mechanism through which this occurs is an osmotic pressure-driven counterforce to the hydrostatic pressure. The macromolecules in the interior of the disc take up fluid as a result of their hydroscopic capacity. In equilibrium, the following equation is manifest:
Equation s. Kap.
Whenever one side outweighs the other (e.g., because of weight bearing), equilibrium is disrupted and fluid moves across the semipermeable membrane. Increased weight bearing causes intradiscal fluid to escape via hydrostatic forces. This increases the concentration of the macromolecules within the disc interspace and results in an increase in the intradiscal oncotic pressure. This, in turn, increases the absorption capacity and nutrient delivery to the disc.22 In addition to the biomechanical effects, this fluid movement allows the passage of nutrients and waste products across the membrane. Therefore, the greater the activity of the subject, the more active this form of fluid movement. Traction (distraction) is an obvious mechanism by which the intradiscal pressure can be reduced, thus causing an increase in intradiscal water content and an increase in disc height. The aforementioned points are summarized in (Fig. 5.3).

The facet joint, being a synovium-lined diarthrodial joint, is subject to the ravages of inflammatory disease processes. This is particularly so regarding seropositive inflammatory diseases (e.g., rheumatoid arthritis).
5.3 Biomechanics of the Intervertebral Motion Segment
During pure axial loading of a disc interspace, intradiscal pressure is symmetrically distributed. Eccentrically placed loads, however, result in the transient asymmetric distribution of pressures within the disc. This, in turn, causes the nucleus pulposus to move within the disc from a region of high pressure (high load) to a region of low pressure (low load) during this transient phase of disequilibrium. For example, forward flexion results in the dorsal migration of the nucleus pulposus. Conversely, the annulus fibrosus responds to asymmetric force application to the disc interspace by bulging on the side of the disc with the greatest stress applied; that is, the annulus bulges on the side opposite the direction of migration of the nucleus pulposus (Fig. 5.4).

Bone responds to loading by reinforcing itself along lines of stress. The columnar-like trabeculation of bone in the vertebral bodies is illustrative of such (Fig. 5.5). Hence, bone remodels itself to meet the demands of the stresses applied to it. In the case depicted in (Fig. 5.5), the remodeling takes the form of bolstering the ability to bear axial loads by forming columns that are in line with the load bearing forces. Conversely, the intervertebral disc does not adapt to loads by functionally remodeling. Instead, it degenerates with time and in response to repetive loading. With aging, loading patterns within the disc change and, in fact, become more dysfunctional. This is illustrated by prior observations with stress profilometry. Stress profilometry, introduced by McNally and Adams,22,23 provided insight into the mechanics associated with the degenerative process (Fig. 5.6). This procedure is performed in loaded spine. A needle (and pressure sensor) is inserted into the disc, across its entire diameter. It is then gradually withdrawn as the pressures are recorded. In youth, the disc interspace is associated with a uniformly high (normal) disc interspace pressure (Fig. 5.6 a). In mid-life, the pressure remains high in the degenerating annulus fibrosus region, but drops internally as the nucleus pulposus dessicates and loses competence (Fig. 5.6 b). As the degenerative process continues, end stage degeneration results in persistently high pressures in the now completely degenerated fibrocartilaginous scar that once was the annulus fibrosus, while the central region of the now very degenerated nucleus is completely incompetent with low pressures (Fig. 5.6 c). With advanced degenerative changes, the loads are borne peripherally (Fig. 5.6 c), whereas in youth, they are borne uniformly across the entire disc interspace (Fig. 5.6 a). With severe degenerative changes, the pressures can dip below zero in the unloaded position. Under such conditions (negative pressure within the disc interspace), gas may form within the disc (vacuum disc) (Fig. 5.6 d).


5.4 Pathophysiology of Disc Degeneration and The Spondylotic Process24–26
Spondylosis is defined as “vertebral osteophytosis secondary to degenerative disc disease.”27 Spondylosis is not to be confused with inflammatory processes that are associated with osteophyte formation or bony overgrowth. Inflammatory processes are associated with osteophyte formation and are grouped together as arthritides. The osteophytes of spondylosis are associated with degeneration of the intervertebral disc, which is an amphiarthrodial joint (i.e., one where there is no synovial membrane). Seropositive arthritides (e.g., rheumatoid arthritis), on the other hand, classically involve the synovial membranes of diarthrodial joints (joints lined with synovium; e.g., the facet joints). The presence of spondylosis is defined, therefore, by the presence of non-inflammatory disc and motion segment degeneration. The process of disc degeneration is complex and involves many alterations of normal physiology, as well as the process of aging. Regardless of the cause or the variety of treatments, disc degeneration is initially associated with segmental instability,20 which then proceeds over time to restabilization in most cases.
5.4.1 Intradiscal Hydrostatic and Oncotic Pressure
Persistent elevation of intradiscal pressures accelerates the degenerative process, which eventually results in narrowing of the disc interspace. This results in annulus fibrosus and facet joint capsule distortion and stretching. This, in turn, results in further motion segment instability. Endplate damage further accelerates this process.28 The degeneration process itself should be considered simply a manifestation of the normal aging process; but its pathological acceleration, or the deterrence of same, is of obvious clinical significance.
The water content of the disc interspace, as previously mentioned, decreases gradually throughout life. In addition, the vascularity of the disc also decreases, ranging from a well-vascularized disc at birth to essentially no vascular supply by age 30. This and other factors contribute to changes in the chemical and anatomic makeup of the disc. Fibroblasts produce inferior-quality fibers and ground substance. The disc becomes desiccated and less able to function as a cushion. Fissures occur in the cartilaginous plates with defects resulting in internal herniations (Schmorl nodes).29 Gas accumulates in the disc (vacuum phenomenon) (Fig. 5.6 d). Mucoid degeneration results in instability. This in turn can lead to further degeneration and other sequelae, such as annulus fibrosus bulging and torsional instability.
5.4.2 Disc Deformation
The bulging of the annulus fibrosus causes the periosteum of the adjacent vertebral bodies to be elevated at the attachment site of Sharpey fibers. Bony reactions (subperiosteal bone formation) occur, resulting in spondylotic ridge (osteophyte) formation (Fig. 5.7 a-c). This process most commonly results in spinal canal encroachment in the cervical and lumbar regions, relatively sparing the thoracic region (Fig. 5.7 d). This is caused by the natural lordosis in the cervical and lumbar regions, which results in a dorsally oriented concavity of the spinal curvature (lordosis) and, hence, the tendency of annular bulging toward the spinal canal. This process is predicated on motion as the inciting factor. The spondylotic process is lessened or eliminated by immobilization or fusion.30 In fact, osteophytes may involute in response to complete immobilization associated with fusion.

Osteophyte formation occurs on the concave side of a curve, where annulus fibrosus bulging is similarly most pronounced (Fig. 5.8). Hence, osteophytes are commonly seen on the concave side of a scoliotic curvature. The concave side of a spinal curvature, however, is usually not the side of the spine that harbors the predisposition for disc herniation. This discrepancy warrants further attention. Osteophyte formation represents, in a sense, a mechanism to stabilize the spine. If osteophytes are removed, strain distribution shifts and the chance for further spine deformation is enhanced.31

As noted flexion and lateral bending cause annulus fibrosus bulging and promote osteophyte formation along the concave side of the curve. Conversely, the thin dorsal annulus fibrosus and relatively weak posterior longitudinal ligament (particularly laterally) combine with the migratory tendencies of the nucleus pulposus to encourage dorsolateral disc herniation (Fig. 5.9). This is most common in the lumbar spine.

Many factors play roles in inducing disc degeneration and dorsolateral disc herniation. These include the aforementioned migratory tendencies of the nucleus pulposus, the relatively weak lateral portion of the posterior longitudinal ligament, the thin dorsal portion of the annulus fibrosus and the morphology of the iliolumbar ligament.32 A familial predisposition has, in fact been suggested.33
Laboratory investigations that attempt to determine the mechanism of disc herniation are lacking—a fact that has hampered investigations in this area for years. Most disc herniations do not occur, or do not become manifest, immediately following trauma. Adams and Hutton, however, determined that a high percentage of lumbar discs in the laboratory could be encouraged to herniate if (1) the disc was degenerated and (2) a specific force pattern was delivered acutely to the motion segment. This force pattern includes (1) flexion (causing posterior nucleus pulposus migration), (2) lateral bending away from the side of disc herniation (causing lateral nucleus pulposus migration), and (3) application of an axial load (causing an increase in intradiscal pressure).34 As shown in (Fig. 5.9), this complex loading pattern causes (1) the application of tension to the weakest portion of the annulus fibrosus (dorsolateral position—the location of the herniation), (2) migration of the nucleus pulposus toward this position, and (3) an asymmetric increase in intradiscal pressure. A degenerated disc is a requisite for the occurrence of this process. These factors, in general, have been corroborated by others.35 These factors, plus the increasing frequency with which annulus fibrosus tears are observed with advancing age and observation of peak nucleus fibrosus pressures in the 35 to 55 age group, give rise to a higher incidence of disc herniation in midlife (Fig. 5.10). Finally, endplate shape has been implicated regarding the tendency to develop degenerative disc disease and disc herniation.36 This, along with the stiffening of the annulus (as it degenerates and evolves into a fibrocartilagenous scar), results in a circumferential constraint on the nucleus pulposus. This can contribute to an accelerated degeneration of the nucleus pulposus.37

Much discussion has focused on disc herniation. Disc migration and herniation, however, is not an obligatory one way street. Disc resorption or involution occasionally occurs as well.38
The terminology for reporting lumbar disc herniation is often inconsistent. A common language regarding this process has been provided by Milette.39 Of note in this regard is that lateral disc herniations are uncommon and fortunately usually respond to non-operative strategies.40 The surgical management of herniated discs via nucleotomy further alters the mechanics of the motion segment. A decrease in disc interspace pressure and endplate deformation results.41 Hence, at least initially, discectomy is associated with a favorable mechanical change.
The disc degeneration process is so predictable and so common that routine pathological examination of operatively resected degenerated disc material not necessary on a routine basis. The surgeon must weigh carefully the cost and advantage to the patient of submitting disc specimens for pathological examination. It appears that unless the surgeon suspects an atypical process on the basis of clinical history, examination, or by gross inspection at the time of surgery, the routine examination of surgically resected intervertebral disc specimens is not warranted.42–44 This is corroborated by the observation that there is no correlation between histology and clinical findings.45
5.4.3 Torsional Instability
Torsional instability, secondary to degenerative disease, may have significance clinically. If a disc interspace has lost height, or if the annular fibers have become lax for any other reason, rotation of a vertebral body can occur about the long axis of the spine. The angular orientation (30°) of the annulus fibrosus fibers is optimal for the prevention of this type of rotation. This, however, is only so if the fibers are taut. Lax fibers secondary to disc interspace narrowing permit rotation, as does fiber lengthening or weakening (Fig. 5.2). It has been hypothesized that this type of torsional instability may be related to mechanical back pain.46 Of note in this regard is that radial and transverse tears of the annulus fibrosus result in a diminished ability to resist torsional motion.47
5.4.4 Extradiscal Involvement
The spondylotic process includes soft tissue pathological processes in addition to disc and facet joint degeneration. In this regard, the hypertrophy and buckling of the ligamentum flavum is a major contributor to the development of myelopathy in the patient population afflicted with cervical spondylotic myelopathy.48 Lateral recess involvement is more common in the lumbar spine. It is often intermittent (dynamic) depending on posture. Extension tends to exaggerate central canal and foraminal narrowing.49 Finally, ligamentous laxity contributes to the degenerative process. With aging, laxity increases. This is predominantly related to a loss of elasticity.50 Such increased laxity results in an augmentation of the stresses applied to all components of the motion segment, with an associated acceleration of the degeneration process. Facet joint opening, as a result of motion segment instability, presents an imaging indicator of such instability. Neutral zone enlargement has been observed to be associated with increased facet joint volume and facet joint opening.51 The assessment of the interspinous ligament by MRI may also provide a useful method of assessing and grading the degenerative process.52
5.4.5 Osteoporosis
With aging (and trabecular abnormalities), a decrease of bone formation with associated continued bone resorption leads to a decrease in bony integrity and deformities.25 This may lead in turn to the collapse of the vertebral body. Unlike the Schmorl node, this collapse is not circumscribed. The thoracic kyphosis predisposes the spine to ventral vertebral body collapse in this region (Fig. 5.11). This applies to trauma in general (see Chapter 7).

5.4.6 Scheuermann Disease
In the developing spine, the intradiscal pressure is normally relatively high. This can result in focal sites of penetration of the endplate (Schmorl nodes) with resulting destruction of the growth plate. The preexisting thoracic kyphosis, which is associated with asymmetrically high ventral intradiscal pressures, may lead to the exaggeration of focal endplate penetration in this circumstance. This phenomenon is known as Scheuermann disease (osteochondrosis). It is associated with a disproportionate loss of ventral vertebral body height, Schmorl’s nodes (predominantly ventrally located), irregularities of the vertebral endplates, and narrowing of the disc interspaces (predominantly ventrally).53,54 As stated by Kramer, “The development disorders of Scheuermann disease are secondary changes and are caused by increased pressure of the developing disc tissue on the ventral aspects of the intervertebral segments in the kyphotic region of the spine.”1
Because of the increased focal pressures exerted, degeneration of the disc is accelerated. Fibrous, and ultimately bony fusion occurs, usually in a kyphotic posture. This can cause significant lifestyle and, hence, clinical management dilemmas.55
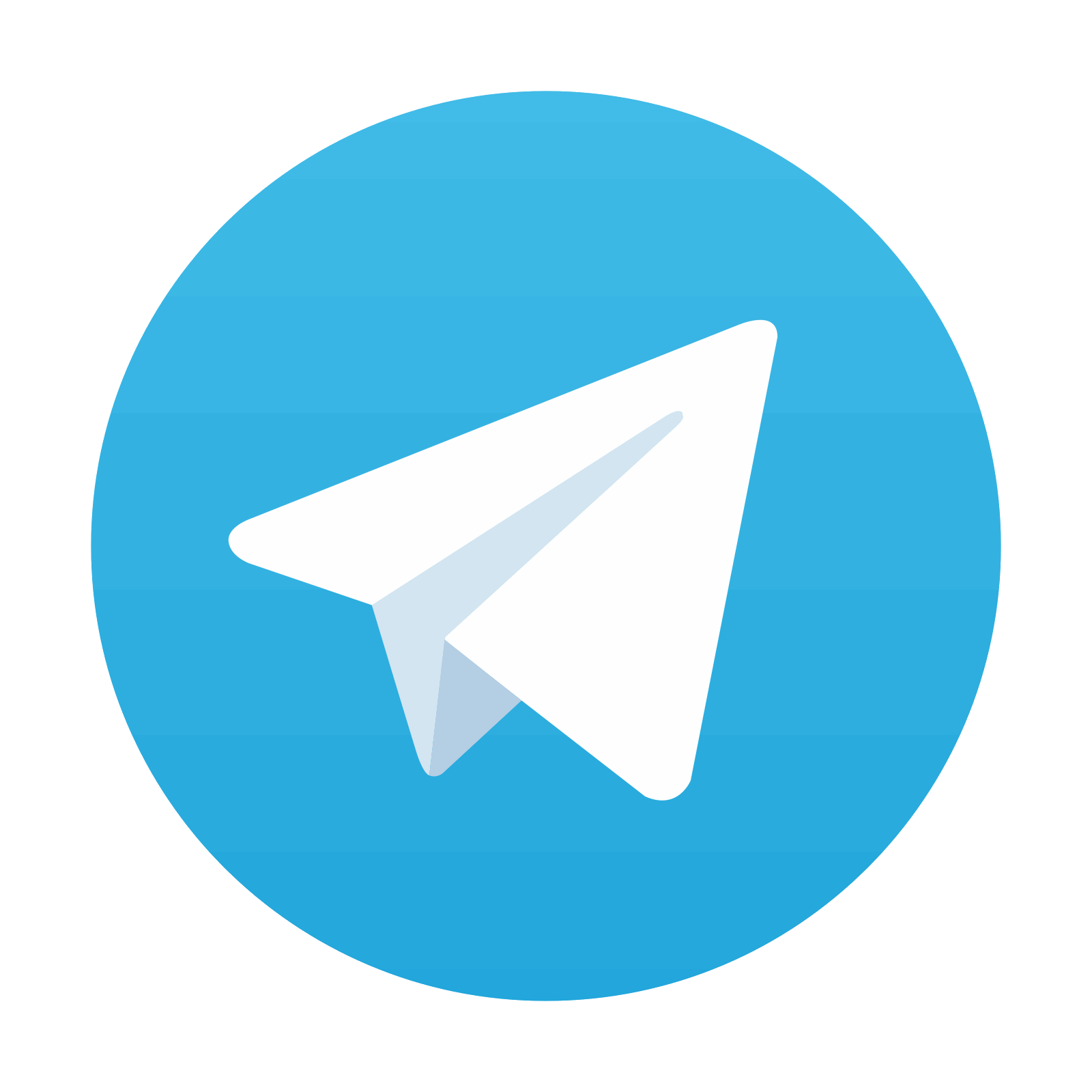
Stay updated, free articles. Join our Telegram channel

Full access? Get Clinical Tree
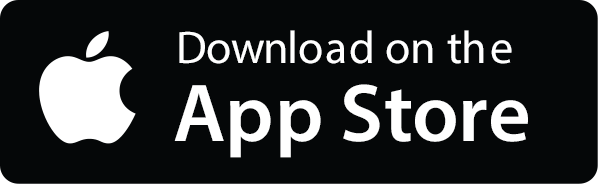
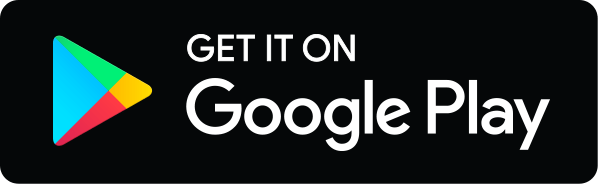
