5 Instrumentation
Abstract
In this chapter, we describe instrumental advances in endoscopic endonasal surgery and their application in pediatric neurosurgery. After reviewing the technical innovations of endoscopes, we will describe the advances in instrumentation following their use at different surgical phases.
5.1 Introduction
Over the last decade, endoscopic endonasal surgery (EES) progressively became the gold standard for approaching the anterior skull base in adults. As surgical instrumentation and techniques continue to evolve, the array of pathologies treatable through this route will continue to broaden. This surgery was mainly developed in adults, given the higher prevalence of midline skull base tumors and the wide aeration of the sinuses; however, with increasing experience and the development of smaller, specially designed instrumentation, application to pediatric EES is increasing. 1 The main principles of EES remain the same in both adults and children; however, potential anatomical and physiological limitations such as piriform aperture, sphenoid pneumatization, clival intercarotid distance, and maxillary crest growth plate preservation must be considered in pediatric endonasal skull base surgery. 2
In this chapter, we will review the advances in instrumentation that allowed adaptation of endoscopic surgery to pediatric patients. These advances will be discussed according to the logic of their use during surgery, starting with the improvements of the endoscopes.
5.2 Endoscopes and Associated Tools
5.2.1 Endoscopes
Successive improvements in endoscopic technology progressively revolutionized skull base surgery. First generations of endoscopes required cumbersome large lenses, and provided low-definition images with poor illumination. These limitations were gradually surpassed by continuous technological advances, allowing currently available endoscopes and high-definition cameras to render an unprecedented wide, high-definition image of the operative field. Endoscope diameter can be as small as 2.7 mm, which can be extremely useful in young children given the small size of the nostrils and sinus cavities. 3
Currently, most endoscopes used for skull base surgery are rigid rod-lens type and are 18 or 30 cm in length and 2.7 or 4.0 mm in diameter. As light is better transmitted through large-diameter endoscopes because of the larger lens, the 4-mm-diameter endoscopes are the most frequently used. Anatomically, when compared to adults, nasal aperture is significantly narrower in children younger than 7 years. This can significantly limit the endoscopic route in younger patients. 4 In these situations, the narrower 2.7-mm endoscope can be of use. Nonetheless, some authors have reported effective use of the 4-mm endoscope in infants whose body weight was in excess of 2.2 kg. 5 Given the small size of the nasal passages, when using a 4-mm endoscope, the instruments are generally passed solely through the contralateral nostril and not in the same nostril as the endoscope, as is often done in adults. The longer length (30 mm) of the endoscope is helpful in situations where an endoscopic holder is used. The holder arm usually attaches to the shaft of the endoscope, and the long length keeps the bulk of the holding apparatus away from the nares. This allows the surgeon adequate room to maneuver the surgical instruments without hindrance from the holder arm.
The lack of depth perception while using classical 2D endoscopes is now mendable using 3D endoscopes. 6 The visualization of spatial relationships between anatomical structures improves surgical dexterity while shortening the learning curve for endoscopic surgeons. 6 The first-generation 3D endoscopes suffered multiple limitations: large shaft diameter, lack of angled lenses, and decreased resolution. Currently, innovative technology is overcoming these limitations with new generation of endoscopes capable of rendering unprecedented 3D view of the surgical field. 6 The better depth perception facilitates anatomical understanding and safe maneuvering of instruments inside the nasal cavity, which is crucial in pediatric patients with unusual anatomic landmarks, and limited intranasal room. The Visionsense 3D-endoscope, (Visionsense, Philadelphia, PA) has been the most frequently used for endonasal surgery given its 4-mm diameter. However, a new 3D endoscope designed by Karl Storz offers a similar diameter with a dual-lens technology that may provide improved color and optics (▶ Fig. 5.1).

Several angled endoscope lenses are commercially available. The standard set usually includes 0-, 30-, and 45-degree objective lenses. The 0-degree lens provides a frontal view of the operative field and is the most commonly used. The 30-degree lens is helpful to operate around corners, and rotating the lens brings a larger surface area of the operative field into view. The 45- and 70-degree endoscopes are mostly helpful for inspection. Operating at such acute angles is not only technically challenging, but also very disorienting for most surgeons. The development of adjustable viewing angle endoscopes, such as the EndoCAMeleon (Karl Storz, Tuttlingen, Germany), allows the surgeon to quickly change the lens angle from 0 to 120° by dialing an adjustment knob, providing instantaneous panoramic visualization of the surgical field (▶ Fig. 5.2).

For illumination, endoscopes are usually coupled to the light source via a fiberoptic cable. Different types of light sources are available (tungsten, halogen, xenon), although currently xenon is the preferred light source for endoscopic skull base surgery, as its spectral characteristics allow for a whiter light than the classic yellow halogen light. 3 The use of fluorescence imaging through the endoscope is possible to visualize fluorescein, 5-aminolevulinic acid (5-ALA), and indocyanine green (ICG). Fluorescein is often used to appreciate cerebrospinal fluid (CSF) leaks, 7 , 8 , 9 while 5-ALA and ICG have been used experimentally to distinguish tumor from the normal gland. 10 , 11 , 12
5.2.2 Endoscope Holder
The use of endoscope holders is optional, as many centers prefer manual endoscope manipulation. However, a stable view of the operative field can be advantageous to facilitate bimanual microsurgical dissection. Therefore, endoscope holders can be a useful adjunct for the endoscopic equipment. 3 They must ensure a stable and secure hold, while having the ability to be easily and quickly adjustable throughout the surgery.
The mechanical holding arms, which are currently most widespread, may exhibit a tendency to drift after placement in their final desired position. However, their low profile makes setup easy (▶ Fig. 5.3). They are also considerably cheaper than the latest generation of pneumatic and electronic holding arms, which even though bulky, help ensure more stable positioning and smooth drive. 3 The most common is the Mitaka Arm (Mitaka, Inc., Denver, CO), which can either be mounted to the bed or rest on the floor (▶ Fig. 5.4).


The more common option is to have the endoscope held by an assistant during surgery. The dynamic movement of the 2D endoscope can help the surgeon receive feedback regarding the anatomy and depth of the operative field. The obvious drawback is assistant (holder) fatigue during long cases, as well as the clustering of hands (surgeon and assistant) near the nostrils. We have found it useful to use the endoscope free hand during the approach portion of the case, and then have it fixed during the delicate intracranial dissection of the pathology.
5.2.3 Irrigation Systems
The close proximity of the endoscope lens to the surgical field exposes it to frequent soiling by blood, fluid, and bone dust. Multiple endoscope irrigation systems are commercially available that flush the lens to solve the problem of frequent blurred endoscopic vision, without having to remove the endoscope from the nostril. One of the available tools is the suction-irrigation sheath (CLEARVISION II/K-ENDOSHEATH) that allows simultaneous intraoperative irrigation and suctioning, controlled by a motorized pedal-activated pump.
A cheaper alternative is to flush the scope with a saline syringe and IV tubing attached to the sheath. Constant negative pressure is applied to the syringe (by gently drawing back on the plunger) to prevent water droplets from accumulating on the lens, and intermittent irrigation at varying intensity and duration can be controlled by the assistant to keep the lens clear. This technique also helps irrigate the surgical field, particularly when the bony surface becomes highly reflective and hot during skull base drilling. Another option is to drip fluid down the outside of the endoscope, which can also clean the scope, but less reliably.
5.3 Preoperative Planning and Surgery
5.3.1 Neuronavigation and Virtual Reality Systems
Pathology at the developing pediatric skull base can frequently distort the anatomy and obscure endonasal surgical landmarks. A thorough preoperative evaluation of the anatomy is therefore necessary with thin slice CT and/or MRI (with angiography). Currently available software tools are able to merge information from CT, MRI, and angiogram for a comprehensive understanding of the encountered anatomy. Preoperative rehearsal of neurosurgical procedures has been shown to help with aneurysm surgery and may also be useful in endoscopic skull base procedures. 13 , 14 Augmented reality may also be useful for intraoperative planning and surgical navigation. 15
Standard frameless neuronavigation is often extremely helpful during these approaches. It is particularly important in a pediatric setting given the less-predictable developing anatomy and the frequent necessity of extensive skull base drilling due to lack of sinus pneumatization. Registration of CT angiography (CTA) images is perhaps the most helpful when drilling out a nonpneumatized sinus to determine the location of the adjacent internal carotid artery (ICA) and the trajectory to the pathology. Real-time navigation of the drill or other surgical instruments can also be helpful.
5.3.2 Neuromonitoring
Intraoperative neuromonitoring (IONM) is often used in open skull base surgery to identify and preserve functionality in proximal cranial nerves. As EES gradually extended beyond the sella to encompass diseases craniocaudally from the cribriform plate to the cervicomedullary junction, and laterally to the cavernous sinus, Meckel’s cave, and infratemporal fossa, comprehensive IONM has also become a helpful adjunct in extended EES. 16 Surgeons should be familiar with the currently available neuromonitoring techniques to better tailor the monitoring montage to the planned surgery and for more effective communication with the neurophysiologist (▶ Table 5.1). Straight-shafted monitored microdissecting instruments are now available that allow the surgeon to continually monitor cranial nerve activity while actively dissecting the tumor from the cavernous sinus or petrous apex.
5.3.3 Microdrills
Exposure and resection of skull base lesions often requires drilling in close proximity of critical neurovascular structures. Lightweight and ergonomic microdrills are now available for EES. Tapered hand pieces are particularly helpful as they offer better maneuverability through the nostril while allowing excellent visibility of their tips. Endonasal drills are now available with straight and angulated tips and can be used in conjunction with neuronavigation (▶ Fig. 5.5).

Drilling usually starts with cutting burrs in the nasal cavity, then switches to diamond burrs in the sphenoid sinus as the dura and ICA are approached. Cutting burrs are aggressive and can be used for rapid bone removal; however, they provide no hemostasis. Diamond burrs provide good bony hemostasis; however, they can be tedious to use and generate excessive heat. To avoid risk of direct soft-tissue damage from cutting burrs and diffused heat from diamond burrs, some authors advocate the use of coarse (or hybrid) diamond burrs. 17 , 18 This might be a good option in the pediatric population, where lack of sinus pneumatization may predispose the surgeon to drill through soft conchal bone. In our practice, we favor the use of Kerrison rongeurs to progressively extend the skull base exposure, once the midline dura is safely exposed and the surrounding bone egg shelled.
An alternative to the drill for removing bone is the Sonopet ultrasonic bone curette. 19 The Sonopet offers the ability for slow bone removal with less risk of damage to underlying dura or neurovascular structures. However, the progress of bone removal is often quite slow when removing thick bone.
5.3.4 Doppler Ultrasonography
In young children, the intercarotid distance is smaller compared to adults, and incomplete sphenoid sinus pneumatization might necessitate extensive drilling, resulting in the absence of classical anatomical landmarks. All these features expose the ICA to injury with potential catastrophic complications, emphasizing the importance of accurate ICA localization before dural opening. 20 , 21 Intraoperative micro-Doppler is a quick, easy, and reliable tool, as it offers accurate real-time data about the proximity of ICAs or any other major vessels. 20 The real-time feedback is particularly helpful in cases where tumor debulking can lead to shifts in neuroanatomy, rendering the neuronavigation less accurate.
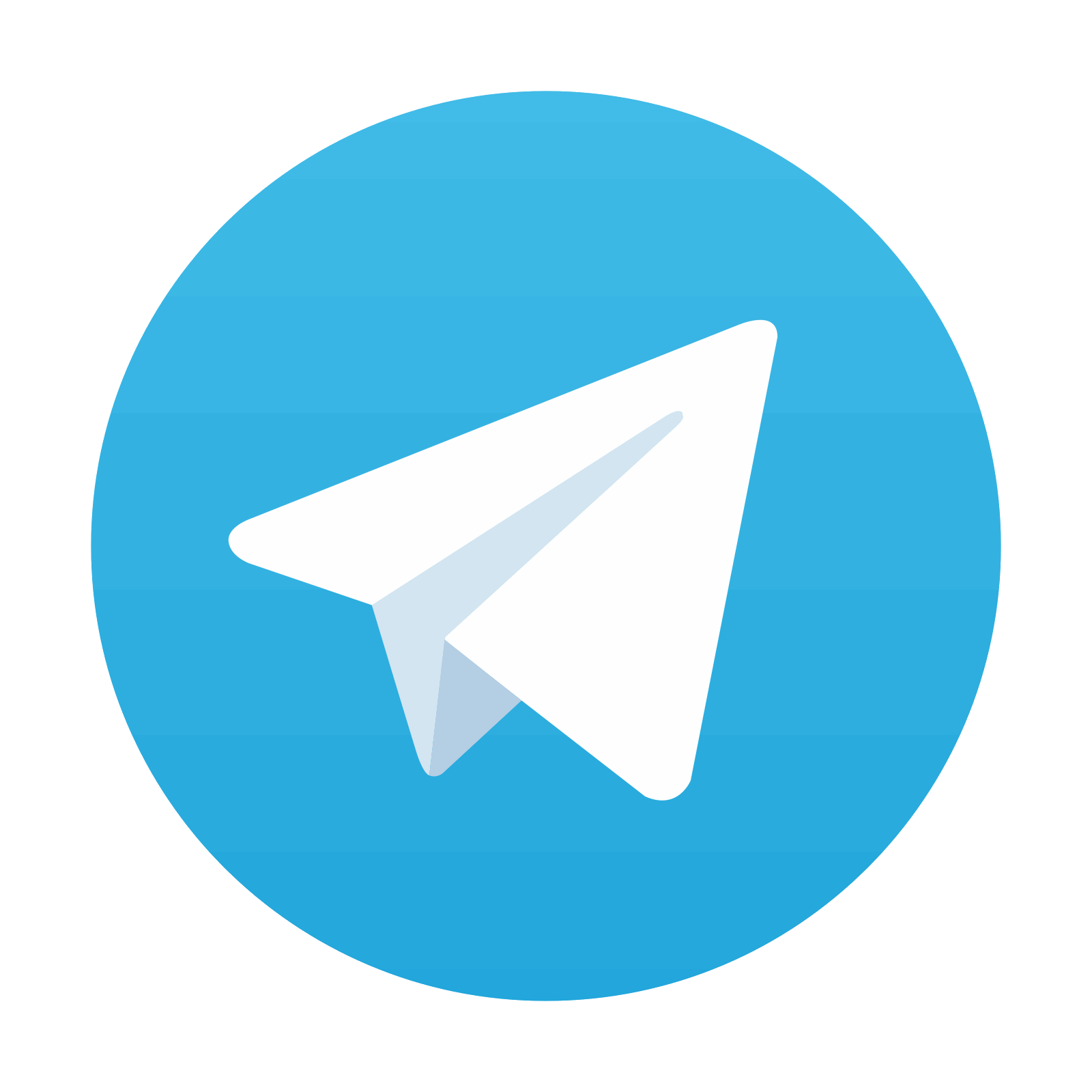
Stay updated, free articles. Join our Telegram channel

Full access? Get Clinical Tree
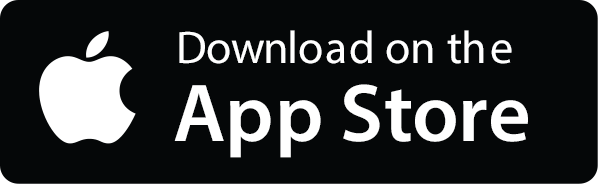
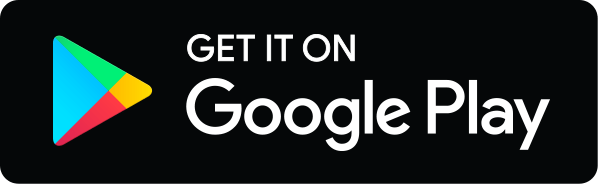
