Abstract
Cerebral oximetry (PbtO2 monitoring) is the direct measurement of the partial pressure of oxygen within brain parenchyma. This chapter reviews the physiologic rationale for intracranial oxygenation monitoring, summarizes the clinical evidence evaluating its effectiveness, and discusses the placement, troubleshooting, and evaluation of intracranial brain oxygenation monitors.
6 Brain Tissue Oxygenation: Procedural Steps and Clinical Utility
6.1 Introduction
Following a neurologic insult, secondary brain injury may occur despite normal intracranial pressure (ICP) and normal cerebral perfusion pressure. 1 The goal of PbtO2 monitoring is to directly assess the metabolic state of the injured brain to determine if treatment is needed to improve cerebral blood flow and oxygenation. In states of generalized brain injury, measurements of mean arterial pressure (MAP), ICP, and resultant cerebral perfusion pressure (CPP) become important guiding points for therapy. However, augmentation of MAP to maintain adequate CPP comes with systemic risks. Since the overarching goal of maintaining appropriate CPP is to provide sufficient blood flow to meet the metabolic demands of the brain, instead of simply measuring ICP and augmenting CPP, direct measurement of brain tissue oxygenation theoretically could be a more useful guiding parameter.
6.2 Relevant Anatomy and Physiology
6.2.1 Anatomic Considerations
Monitor placement requires opening the calvaria and meninges. Optimally, an area of cortex that is relatively noneloquent and easy to access is the target. The frontal lobes, anterior to the coronal suture, are an ideal place for intracranial monitors. Choosing a point in the midpupillary line, approximately 2 to 3 cm behind the hairline, serves as a good start. If the patient’s hairline is not clear, palpation of the coronal suture should help guide entry site. Approximately 3 cm anterior to the coronal suture is usually a safe region.
6.2.2 Physiologic Principles
At rest, the brain accounts for 25% of the metabolic demand of the human body. Many observational studies have borne out that low PbtO2 correlates with increased risk of death and poor outcome in traumatic brain injury. PbtO2 values less than 20 mm Hg appear to be harmful. 2 , 3 , 4 , 5 , 6 , 7 Despite the well-established correlation between low PbtO2 and adverse outcome, it is unclear whether PbtO2 goal-directed treatment protocols can alter the disease course of traumatic brain injury. There have been a handful of cohort studies comparing treatment protocols for traumatic brain injury based upon monitoring of ICP in conjunction with PbtO2 versus monitoring of ICP in isolation. The results of these have been mixed. Several studies have shown that PbtO2 monitoring improved outcomes. 8 9 One study demonstrated that outcomes were better in the ICP monitoring group without PbtO2 monitoring, 10 and others have shown no difference. 11 , 12
6.2.3 Devices
A number of PbtO2 monitoring devices are commercially available, including the Licox (Integra, Plainsboro, NJ, USA), the Neurovent (Raumedic AG, Münchberg, Germany), and the Oxylab (Oxford Optronix Ltd, Oxford, UK). For all such devices, a twist drill is used to facilitate insertion of a probe into the white matter of the brain. The Licox probe contains a Clark polarographic electrode composed of a silver electrode (anode) and a platinum electrode (cathode) with a membranous covering. As oxygen enters, it is reduced at the cathode and the anode-to-cathode electron flow results in production of water. The rate at which this reaction occurs is directly proportional to the amount of oxygen present; more oxygen means more reduction and more electron inclusion. This creates a Galvanic current (direct current) and can be converted into a partial oxygen pressure. The Neurovent and Oxylab probes contain luminescent ruthenium which emits light. The ruthenium luminophore is deactivated by oxygen. This process is known as luminescence quenching. A photodetector measures the amount of light emitted which can then be used to calculate the PbtO2 13 (see ▶ Fig. 6.1). Both methods are highly reliable and accurate. Response time and precision tend to be slightly better in the optical luminescent model; however, clinical significance is negligible. There is concern for drift as with any intracranial monitoring device, that is, unintended change in calibration over time. The basic components of each of these devices consist: (1) fiber-optic or electrical lead/wire, (2) anchoring bolt for cranial securement, and (3) external monitor to which the lead/wire connects.

6.3 Indications
Theoretically, PbtO2 monitoring can be a powerful tool in the management of patients with suspected compromise of cerebral perfusion. At the present time, there is no high-quality evidence demonstrating that treatment algorithms based upon PbtO2 measurements improve clinical outcomes. PbtO2 monitoring is employed most frequently in the setting of traumatic brain injury. The optimal insertion site for a PbtO2 monitor is unknown. To obtain a global assessment of brain oxygenation, it may be placed in the frontal white matter contralateral to the injury. Alternatively, the “penumbral” brain tissue surrounding the site of maximal injury may be a logical site for the device to be inserted. Currently, there is no evidence to support one approach over the other.
In clinical practice, PbtO2 monitoring is believed to offer particular benefit in poly-trauma patients with both brain and lung injuries. In such cases, PbtO2 measurement can help to determine an appropriate balance between neurologic and pulmonary protective treatments.
Another possible application for PbtO2 monitoring is the detection of vasospasm in subarachnoid hemorrhage. While theoretically promising, a prospective observational study concluded that ischemic events detected by this method could not be corrected rapidly enough to improve outcomes. 14
6.4 Contraindications
The use of invasive PtO2 monitoring is contraindicated in patients who are at substantial risk of complications that would outweigh the clinical benefit of the real-time oxygenation data. This includes patients with an increased risk of hemorrhage, as well as those with infection of the tissue into which the device will be inserted. Magnetic resonance imaging (MRI) compatibility is device-dependent, and certain devices may be contraindicated in an MRI environment.
6.5 Equipment
6.5.1 Pre-Op Checklist and Equipment/Supplies
Nursing staff and assistant if available
Semi-sterile room
Drapes
Personal protective equipment (PPE) including gown, gloves, mask, and hat
Extra packages of sterile gauze, saline, and dressing supplies
Review of patient’s imaging and blood work
Intracranial access supplies and PbtO2 monitor supplies (usually part of kit)
Ensure all team members know the key procedural steps and know where additional supplies are in case replacements are needed
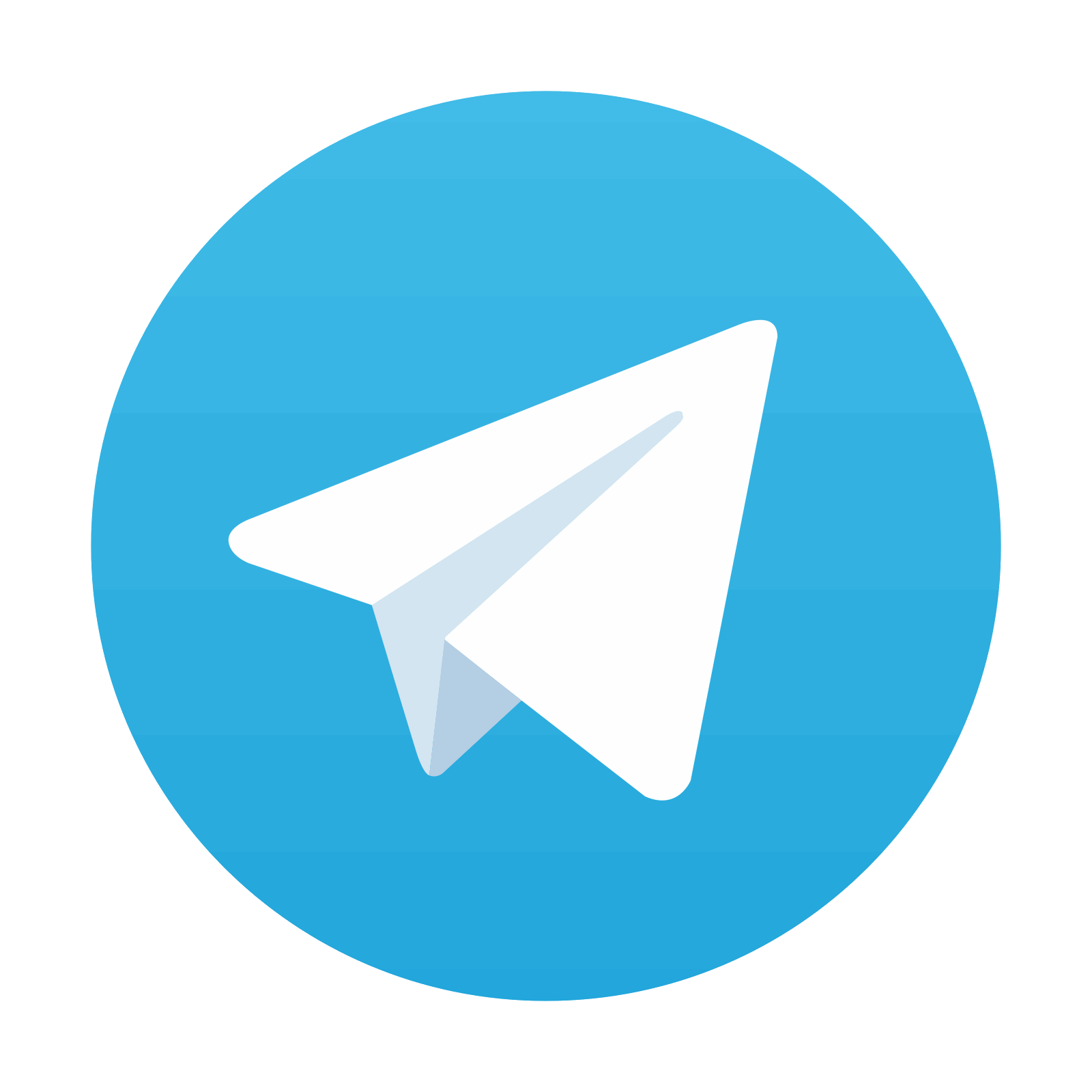
Stay updated, free articles. Join our Telegram channel

Full access? Get Clinical Tree
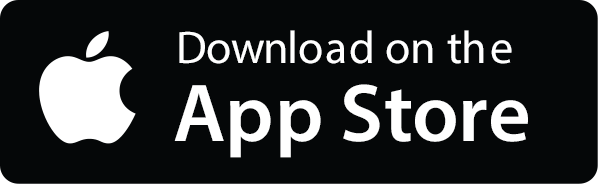
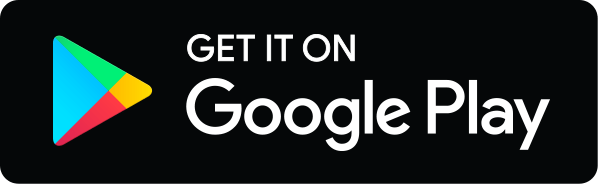
