6 Extra-operative and Intra-operative Electrical Stimulation
The surgical resection of epileptic tissue is an established treatment for patients with intractable focal epilepsy and has become an important field in epilepsy and neurosurgery.1 Pediatric epilepsy surgery is no longer a treatment of last resort.1 There is growing evidence from expert centers that children with surgically remediable focal epilepsy syndromes should be referred and selected early for presurgical evaluation and subsequent operation to optimize seizure control and longterm psychosocial outcome.2
Unique pediatric aspects necessitate a specific pediatric approach for referral, diagnosis, and management, which has been outlined in the recent recommendations of the International League Against Epilepsy (ILAE) subcommission on pediatric epilepsy surgery.3 Similarly the pediatric presurgical evaluation requires specific pediatric epilepsy expertise of experienced and knowledgeable pediatric epilepsy centers.4 An accurate description of the phenomenology of epileptic seizures, classification of seizure types, the specific epilepsy syndromes, an etiologic diagnosis, and an optional assessment of impairments and co morbidities along the lines of the ILAE classification guidelines will be the basis of early referral and optimized management of infants and children for surgical evaluation and treatment.5
Pediatric Aspects of the Presurgical Evaluation
The presurgical evaluation should be considered as a multimodal diagnostic approach, with the goal of localizing the epileptogenic zone, defining the epileptogenic substrate, and delineating neighboring functional cortex to reduce risk for neurological deficits with surgical removal.6 Outcome studies suggest that the cortical regions that underlie epileptogenicity must be excised entirely because residual epileptogenic tissue increases the risk for persisting postoperative seizures.7 Compared with adults, medically resistant partial epilepsies in children are more heterogeneous in terms of anatomical localization, types and extent of pathologies, and electroclinical functional characteristics. The epileptogenic lesions may be discrete structural changes that are very amenable to surgical removal or extensive and widespread macroscopic or low-grade microscopic developmental or acquired lesions.4 The adult temporal lobe epilepsy syndrome is a well-defined, electroclinical syndrome caused by hippocampal sclerosis as its dominant etiopathological finding.8 By contrast, seizures of temporal lobe origin in childhood are a particular diagnostic challenge because they typically involve the neocortical regions and may extend beyond the margins of the temporal lobe rather than being confined to the mesial temporolimbic structures.9
In addition, the seizure semiology is highly age dependent with prominent motor features (tonic, myoclonic, spasms) that are more typical of extratemporal onset and not suggestive of temporal limbic localization.10
Invasive electroencephalography (EEG) and functional mapping may be indicated to properly tailor a cortical resective procedure after the ictal onset zone and eloquent cortex have been clearly localized with these procedures.11 The high incidence of extratemporal neocortical epilepsy in pediatric patients makes this an important consideration.4
The anatomical landmarks of cortical areas subserving important functions, such as sensation, movement, language, and vision, have been well delineated. However, there is sufficient interindividual variation that may be accentuated with associated developmental or acquired pathological conditions through a process of intrahemispheric or interhemispheric reorganization. Thus careful and individualized presurgical investigation with functional cortical mapping is essential for each patient on a case-by-case basis.
A Historic Note
After extensive studies in animals, cortical stimulation in humans was performed by Victor Horsely in London, Fedor Krause in Berlin, Harvey Cushing in Boston, and Ottfried Foerster in Breslau.
In the 1950s, the first pediatric patient was probably evaluated with cortical stimulation by Penfield and Jasper in Montreal. This 4 year old had tuberous sclerosis and epilepsy arising from the central region.12 Penfield and Jasper performed intraoperative electrocorticography (ECoG) and found a well-localized spike focus in the right central region. With cortical stimulation, they reproduced the left clonic seizures of the patient before resecting that area. They also reported a 16-year-old girl who had active spontaneous spikes over the first temporal gyrus and midtemporal region and reproduced her habitual aura of fear by stimulating the anterior insula close to the junction with the uncus.12,13 The appearance of “dreamy states” on stimulation of the uncus and other clues from animal studies and ECoG led Penfield and Jasper to believe that the mesial and inferior parts of the temporal lobe were the origin of many epileptic attacks. Resection of these regions subsequently improved the outcome significantly.
The pioneering work of Penfield and Jasper led to the mapping of the anterior and posterior language areas and visual and cortical areas and further refined the cortical map of sensory and motor representation.12–14 Subsequently, many studies have described the utility of cortical stimulation either used extraoperatively or intraoperatively in adults and children to delineate cortical functional areas in relation to areas of epileptogenesis before surgical removal.14
Goals of Stimulation in Presurgical Evaluation
Extraoperative stimulation is achieved with direct electrical stimulation of the cerebral cortex via subdural or depth electrodes.11,15–17 This technique has been in use for more than 40 years, and two effects commonly observed have been described in the extensive literature. Cortical stimulation may activate cerebral function producing positive phenomena such as tonic or clonic movements, and special sensations, or cortical stimulation may inhibit function producing negative phenomena such as speech arrest or arrest of motor function. However, in the pediatric population, mapping of cortical function with direct stimulation may be less reliable because of limited patient cooperation and the absence of or inconsistent cortical responses of the immature cortex at lower stimulation thresholds compared with adults.11,18,19
Once interictal discharges and sufficient seizures have been recorded from intracranial electrodes, cortical stimulation is performed in a systematic fashion, usually over several days, depending on the number of electrodes, the area that needs to be mapped, and the patient’s degree of cooperation. Because there is a potential to induce seizures, ECoG monitoring is essential to detect after-discharges that may herald increased epileptogenicity under the stimulated cortex. To reduce the risk of stimulation-induced seizures, anticonvulsants that may have been reduced or stopped to activate seizures for video-EEG recording and analysis should be restarted before initiating the cortical stimulation studies. In addition, temporary benzodiazepine coverage during the procedure may be useful.
Physiology of Cortical Stimulation
The neurophysiological effects of extracellular neuronal stimulation has been studied extensively and was reviewed by Ranck in 1975.20 The voltage distribution in neural tissue after electrical stimulation depends on the current density (which is a function of stimulus frequency and wave forms) applied, as well as the stimulation-induced membrane polarization. The electrical field within brain tissue produced by stimulation of a subdural electrode has a complex three-dimensional shape, and underlying neural processes may be subject to depolarizing or hyperpolarizing events from the stimulation, which may depend on stimulation parameters, the cellular geometry of cortical pyramidal neurons, and the position of the neuron in relation to the stimulating electrode.16,21
The effect of the applied stimulus on local neuronal cells near the stimulating electrode may be considered to result in a direct effect of the applied electrical field on the local cell or the indirect stimulation induced transsynaptic excitation and inhibition, which results from activation of a large number of axon terminals leading to increased synaptic activity on the dendritic tree of the local cells. Depending on the types and numbers of synaptic receptors activated, the transsynaptic activity induced by electrical stimulation may be excitatory, inhibitory, or a blend of both.
Generally, with appropriate stimulus conditions, the maximal current density is achieved beneath the stimulated electrodes so that the stimulus-related responses usually represent cortical function in the crown of the gyrus, whereas the banks of the sulcus are not investigated with this method. Potentially, there may be distant current spread to produce positive responses from remote areas.
Cortical excitability in children is known to be different from adults, and electrical stimulation at maximal stimulus intensity, as will be discussed later, may not elicit a positive response after stimulating functional cortex. This may result in yielding a false-negative response and the risk of removing potentially functional cortex.11,18,19
Safety Issues and Complications in Pediatric Patients
The use of subdural or depth electrodes is an invasive procedure that may be complicated by infection, hemorrhage, edema, mass effect, or infarction. The probability of complications associated with intracranial electrodes has been reported by various centers at 2 to 4%. Patients who have undergone prior high-dose brain irradiation may be at particular risk for developing reactive cerebral edema necessitating emergent removal of subdural electrodes. This has only been reported in adult patients.22 In the pediatric age group, the complication rate of subdural electrode implantation is also reportedly low and comparable to the adult experience.11
The energy applied to the surface of the brain via electrical stimulation per se may add an additional risk to damaging the cortex surface. Microscopic studies, however, have not shown gross structural damage, but mild inflammatory responses have been demonstrated in the pathology of the resected tissue.23,24
The use of magnetic resonance imaging (MRI) compatible platinum electrodes over stainless steel electrodes allows for coregistration imaging of the electrodes with MRI.25 There is, however, no reported safety data on higher field strength MRI and subdural electrode (SDE) compatibility, and practitioners need to proceed with caution at this age. There is no evidence that cortical stimulation produces kindling because the after-discharge thresholds do not progressively decrease with repeated stimulation over a cortical region, although the thresholds may be variable with repeated stimulation.
General Principles and Techniques
The standard stimulation paradigm for extracortical cortical stimulation via subdural grid electrodes uses biphasic rectangular pulses delivered at a rate of 50/s in trains lasting 3 to 5 seconds.11,17,18 The pulse duration is held constant at 0.3 milliseconds, whereas the stimulus intensity is increased in a stepwise fashion to a maximum of 15 mA or to a stimulus intensity that elicits a clinical response or after-discharge less than the maximal threshold of 15 mA.
The following stimulation parameters are used routinely in adult patients at the Cleveland Clinic Epilepsy Center: A stimulus is applied for a duration of 2 to 5 seconds to an active electrode with a frequency of 50 Hz as a biphasic square wave, a constant current of 300 microseconds duration with incremental steps of 1 to 2 mA over a range of 1 to 15 mA.16 As the stimulus intensity is gradually increased to 15 mA, either positive responses are elicited or after-discharges occur. Positive motor responses are elicited at the primary and supplementary motor area; sensory responses are elicited at the primary and secondary sensory area; negative motor responses are elicited at the primary and secondary supplementary negative motor areas; and language dysfunction is elicited over Broca’s, Wernicke’s, and the left basal temporal language area.15,17,26–28 Special symptoms resulting from cortical stimulation of the dominant parietal cortex may include agraphia, acalculia, finger agnosia, and left-right confusion seen in Gerstmann syndrome.29 A variety of auras have been reproduced by cortical stimulation.30
A distant reference electrode over a noneloquent region of the cortex serves as a nonactive current sink. The active electrode is switched systematically from electrode to electrode across the entire grid allowing the function of the cortical area underlying each electrode to be investigated.16
There is a paucity of studies and reports about stimulation parameters in the pediatric population. However, conventional stimulation paradigms based on fixed pulse duration as described previously for adults rarely elicit responses in infants and young children.11,18,19 Various pediatric centers have developed and published stimulation paradigms that rely on increments in both stimulus intensity and pulse duration. These will be discussed in more detail.
Once eloquent cortex has been identified, a resection map based on the interictal and ictal epileptiform activity, which together define the epileptogenic zone and the geography of the surrounding eloquent cortex, is designed to allow maximal resection of the epileptic and associated lesional cortex with sparing of surrounding eloquent regions.
In general, resection of primary eloquent cortex, which includes the primary motor, sensory, language, visual, and memory areas, results in neurological deficits. However, some secondary or accessory eloquent regions may be resected without significant permanent neurological deficits. These include the basal temporal language area, negative and second sensorimotor areas, and even the primary motor face area, which is bilaterally innervated.
Cortical Stimulation, Brain Maturation, and Special Considerations in Children
Stimulation results in the pediatric age group are highly subject to ontogenetic and maturational features. When performing cortical stimulation in children, the language and motor tasks and paradigms need to be adapted to the patient’s age and neurodevelopmental status to include considerations of the individual child’s ability to cooperate and performance limitations caused by attention and comprehension. This may be more time-consuming, and several sessions may be needed to obtain workable stimulation results.11,19
An efficient and safe paradigm for eliciting responses from immature cortex based on physiological principles of stimulation and neural maturation has been elegantly studied and described by Jayakar et al.9,18 The response characteristics of neural tissue in relation to stimulus parameters is best described by a strength-duration (SD) curve plotting the current intensity needed to produce a response as a function of pulse duration. The minimum intensity required to elicit a response at a very long pulse duration is termed the rheobase, and the pulse duration required to elicit a response using stimuli at twice the intensity of the rheobase is defined as the chronaxie. The chronaxie represents the safest point on the SD curve for eliciting a response and is significantly affected by myelination being considerably longer in unmyelinated fibers. The SD curve thus shifts to the left as axons myelinate and the chronaxie shortens. By increasing the stimulus intensity and the pulse width from the usual 0.3 milliseconds used in adults to 1 millisecond, the longer chronaxies in children can be more effectively stimulated, and positive responses or after-discharges can be elicited.9,18 A graphic computation of the energies delivered at all values of current intensity between 1 and 15 mA and pulse duration between 0.3 and 1.0 milliseconds has been published, and the points on the graph corresponding to progressively higher levels of energy sequentially traced.18 A stimulation paradigm following this tracing would have the least energy increment at each step but would be extremely cumbersome for routine clinical use. The authors therefore selected three sequences of alternating increase and decrease of intensity and pulse duration that approximates the outline of the tracing. This dual-increment paradigm starts with an intensity of 1 mA and pulse duration of 0.3 milliseconds, and with each subsequent trial, the intensity and pulse duration are adjusted by 1 to 2 mA or 0.1 to 0.2 milliseconds, respectively, until clinical responses or after-discharges are obtained. In three patients aged 1,3 and 4.5 years, positive cortical responses were elicited using the dual-increment paradigm after the standard fixed pulse duration paradigm failed to elicit clinical responses or after-discharges. Thus the technique of dual-increment stimulation rather than the standard fixed-duration paradigm should be used in young children to accurately define critical cortex in the immature brain, facilitating safe excision of adjacent epileptic tissue.
There are few studies defining detailed data of sensorimotor functional maps of the developing brain in children.11,18,31 This is partly because of the higher medical risks associated with implantation of SDE, difficulties with patient cooperation, and increased necessity for anesthesia. However, there is good evidence that the stimulation threshold to activate normal and functionally abnormal cortex is higher in infants and young children.
Clinical motor responses are frequently obtained at or above the after-discharge threshold so that the stimulation paradigm may need to “override” the after-discharge to obtain eloquent responses. Other authors have reported that they were able to elicit responses in young patients younger than 5 years of age by increasing the stimulus duration after failing to obtain any responses at the maximal fixed duration stimulation.32
Motor responses with tongue movement are difficult to achieve in children younger than the age of 2, and the motor responses from the lower face tend to be bilateral rather than contra- and unilateral when the lower rolandic cortex is electrically activated.19
Individual finger movements are usually first noted after the age of 3 years, and clonic movements appear subsequent to tonic movements in response to electrical stimulation of the central cortical hand area. This reflects maturational processes involving motor neuronal pathways in the cortical areas 4 and 6. Besides the effect of maturation on cortical stimulation results, there is ample evidence for atypical functional networks in children and adults with developmental and early lesions. This will be discussed later.
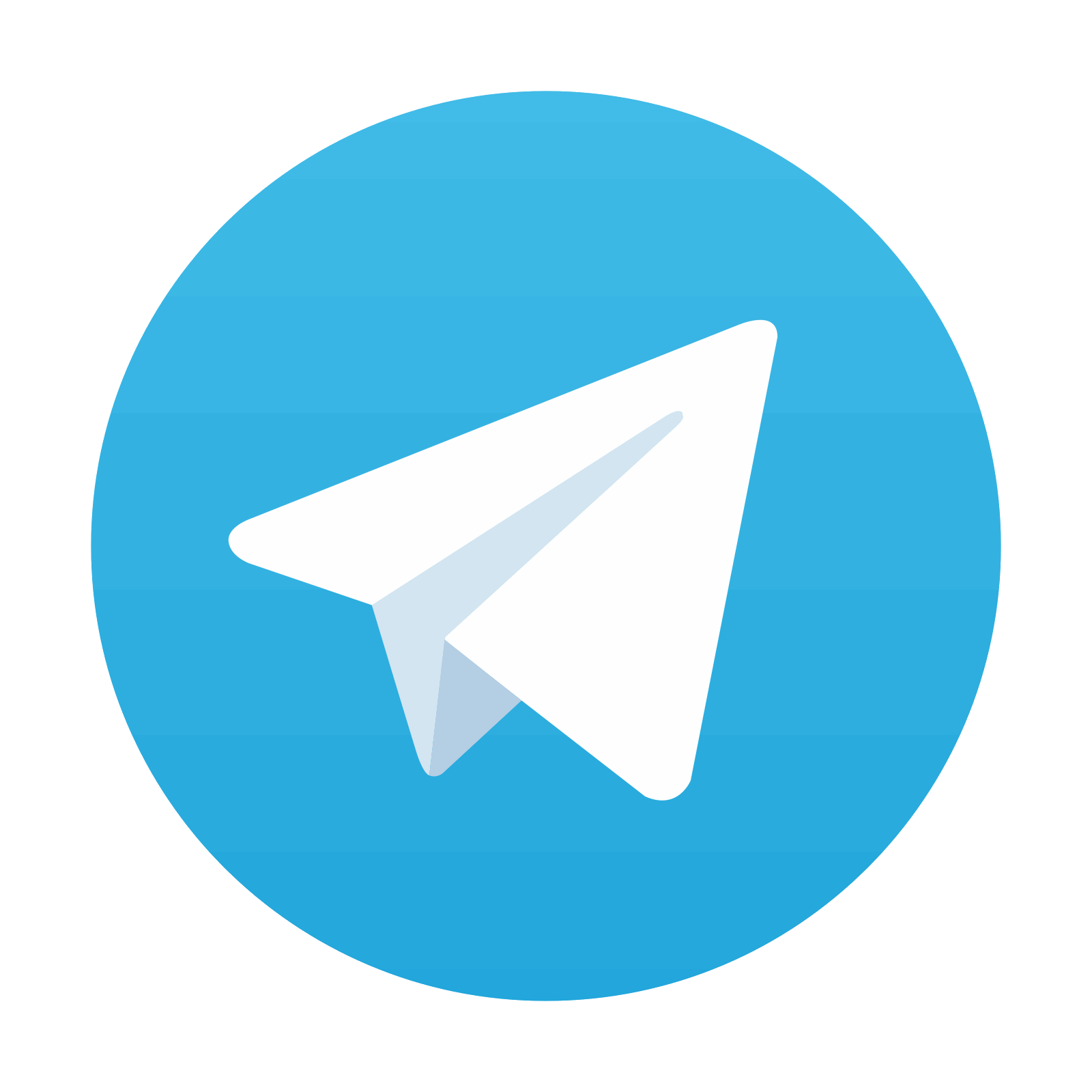
Stay updated, free articles. Join our Telegram channel

Full access? Get Clinical Tree
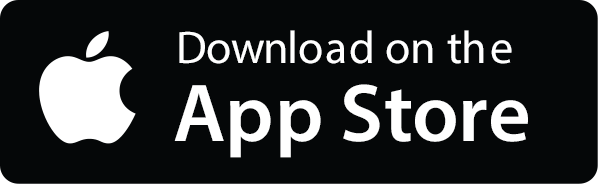
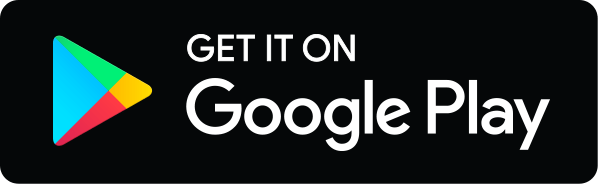