6 Neuromonitoring for Brainstem Surgery
Abstract
Neuromonitoring during brainstem surgery is essential to ensure patient safety and good outcomes for patients who undergo these challenging procedures. Neuromonitoring can assist the surgeon in localizing important neural structures and can thereby provide a safe entry zone for an atraumatic approach to the lesion using mapping protocols. It also provides a continuous assessment of the functional integrity of neural pathways via the monitoring of important tracts and nuclei. Intraoperative neuromonitoring covers a large variety of methods that must be selected according to the pathology at hand. This chapter focuses on the most important and widely used monitoring techniques, such as somatosensory evoked potentials, motor evoked potentials, brainstem auditory evoked potentials, visual evoked potentials, and electromyography of cranial nerves, as well as the mapping of cranial nerve motor nuclei and their tracts. These techniques are explained and discussed with an emphasis on their limitations and relevance for specific pathologies.
Introduction and Indications
Surgery in and around the brainstem is extremely challenging because of the brainstem’s complex neuroanatomy and the high risk of injury to vital neurologic functions. Pathology in this area can further distort the anatomy, making it impossible to recognize typical anatomical landmarks. Even though neuroimaging has advanced tremendously, it is often not possible to predict the location of certain neural structures with regard to the pathology at hand. Advanced intraoperative neuromonitoring (IONM) can assist the surgeon in localizing important neural structures and can thereby provide a safe entry zone for an atraumatic approach to the lesion using mapping protocols. It also provides a continuous assessment of the functional integrity of neural pathways via the monitoring of tracts and nuclei. Cranial nerves (CNs) and their motor nuclei or fiber tracts can be located at their origin, at the floor of the fourth ventricle, or within the brainstem by direct stimulation. Continuous monitoring of sensory, motor, and auditory pathways can directly influence the operative strategy and can thereby prevent complications such as the impairment or loss of neural function. Neurologic deficits can be reduced, or even avoided, only if the evoked potentials mirror the current state of surgery (time equivalence), meaning that changes must correlate with surgical steps. The availability of new computer technology has made this technical accuracy possible. Specifically, the ability to average data and compare it to a baseline study (evoked potentials) have made modern monitoring of complex neurosurgical procedures possible. In this way, IONM can provide critical, decision-guiding information as it is needed.
IONM covers a large variety of different methods that must be selected according to the pathology at hand. 1 In this chapter, we focus on the most important and widely used monitoring techniques, such as somatosensory evoked potentials (SSEPs), motor evoked potentials (MEPs), brainstem auditory evoked potentials (AEPs), visual evoked potentials (VEPs), and electromyography (EMG) of CNs, as well as the mapping of CN motor nuclei and their tracts.
Methods
Anesthetic Considerations
An experienced neuroanesthesiologist is a mandatory member of the surgical team because IONM is highly dependent on anesthesia. Analgesic sedation must be sufficiently and precisely controlled with the aspiration of achieving a marginal but predictable effect on neuronal activity. If possible, the neuroanesthesiologist should create a stable anesthetic environment before the baseline signal is attained, and the technique should ideally not be varied during the procedure. Pharmacologic and physiologic factors can both affect SSEPs and MEPs. For example, evoked potential waveforms can be changed by a drug that alters axonal impulse conduction. However, all monitoring modalities are not equally sensitive to anesthetic technique. Sensitivity is enhanced in long pathways with more synapses. SSEPs are, in general, less sensitive to anesthetics than MEPs. 2 Also, a signal from an upper extremity is easier to obtain than a signal from a lower extremity. Thus, lower-extremity MEPs are the most difficult signals to obtain.
Controversy exists over which anesthetic regimen should be used during surgery with intraoperative monitoring. Inhalational anesthetics and, to a lesser extent, intravenous anesthetics both decrease waveform amplitude and increase latency. Both agents also depress signal obtainment. However, at equal minimum alveolar concentrations, inhalational anesthetics result in greater depression. 3 In sensory evoked potential monitoring, the use of inhalational agents, compared with intravenous agents, results in a more severe reduction of amplitudes and delay of latency. Furthermore, at an inhalation concentration above 0.5 of minimum alveolar concentration, the motor threshold becomes significantly elevated. Thus, we recommend the use of total intravenous anesthesia using propofol in combination with an opioid. Bolus injections should be avoided completely, if possible, because these injections carry the risk of an acute loss of neuromonitoring signals. Inhalational anesthetics can be used, if necessary, but only with a minimum alveolar concentration below 0.5. Short-acting muscle relaxants, such as pancuronium, should be used during the opening and approach to the brain-stem lesion. However, there should be no residual effect of the muscle relaxant at the start of brainstem mapping or monitoring, and muscle relaxants are prohibited from this point onward in the surgery. In any event, communication between the anesthesiologist and neurosurgeon regarding change in anesthetic technique or bolus administration is important for accurate interpretation of the signals obtained. Signal depression from an anesthetic agent would be global in nature, whereas true injury may be specific to the surgical area.
Bispectral index monitoring can be used to measure the depth of anesthesia. This noninvasive continuous measurement is provided by a strip-like sensor that is positioned on the patient’s forehead. The range of measurement that is derived from electroencephalogram (EEG) data reaches from 0 (equivalent to EEG silence) to 100 (equivalent to fully awake) with a desired bispectral index value between 40 and 60 during neurosurgical procedures. A significant reduction in anesthesia awareness during surgery can be achieved by using bispectral index monitoring. 4
Monitoring
Evoked Potentials
Evoked potentials are a specific response that follows an electrical stimulation of a certain part of the nervous system. In the case of brainstem monitoring, these evoked potentials are usually somatosensory, motor, or auditory. The latency and size of the amplitude of these potentials carry important information about the monitored system. Consequently, evoked potentials present a functional testing of the respective neuronal pathways and allow conclusions to be made about their integrity. A precise analysis of these specific responses has only been made possible through the introduction of monitoring computers that sum and average evoked potentials, eliminate additional “noise” from baseline EEG and muscles, and amplify the naturally low nerve potentials.
Somatosensory Evoked Potentials
SSEPs enable the reliable monitoring and control of the somatosensory system. Depending on the location of the procedure, different peripheral nerves may be stimulated. Typical stimulation sites include the posterior tibial nerve at the middle ankle, the common perineal nerve behind the knee, the median and ulnar nerves at the wrist, and the ulnar nerve at the cubital tunnel at the elbow. Stimulation is performed using a bipolar probe, which is directly placed over the corresponding nerve. Detection of the action potential, spreading along the ascending fibers of the dorsal column into the primary sensory cortex, is performed using electrodes in the scalp over the contralateral postcentral gyrus (C3, C4 international 10–20 EEG system) 5 , 6 ( Fig. 6.1 ). 7 Stable potentials with an adequate signal-to-noise ratio are expected by averaging 250 single potentials. The stimulation intensity can be as high as 50 mA. Prolongation of the single stimulus and reduction of the frequency of stimulation can be used to increase the quality of the evoked potentials, if desired. Depending on the location of surgery, additional recording e lectrodes can be placed at peripheral locations (popliteal fossa and Erb’s point) and along the cervical spine (C2, C7).

Factors that may influence changes in SSEPs independent of surgical manipulations are a decrease in body temperature or blood pressure, pneumocephalus, and changes in anesthesia.
Motor Evoked Potentials
MEPs enable the reliable control of the motor system. Potentials are evoked by direct electrical or magnetic stimulation of the exposed motor cortex or by transcranial stimulation. Transcranial electrical stimulation with a train or multipulse stimulation technique has become the standard procedure for intraoperative use. Stimulation is best performed using corkscrew electrodes over the precentral gyrus (C3 and C4 international 10–20 EEG system). The detection of MEPs descending along the pyramidal and corticospinal tract is best performed using subdermal needle electrodes in the corresponding muscles on the side contralateral to the stimulation. Typical muscles for intraoperative use are the abductor pollicis brevis and flexor muscles of the forearm for the upper extremity, as well as the abductor hallucis brevis and anterior tibial muscle for the lower extremity. A stimulation intensity of 100 mA is generally adequate. The intensity can be as high as 200 mA but should not exceed this level to avoid adverse effects, such as burns and epileptic seizures. In the case of direct stimulation of exposed motor cortex, an intensity of only 10–20 mA is adequate. Changes in latency, amplitude, or motor threshold suggest structural damage of the motor system. Factors strongly influencing MEPs in a negative manner are halogenated anesthetics (e.g., enflurane, desflurane, and isoflurane) and muscle relaxants. Other factors include blood pressure changes and compression of peripheral nerves in cases of poor patient positioning.
Corticobulbar Tract MEP Monitoring
The exact anatomy of the corticobulbar tract (CBT) is not known. According to magnetic resonance imaging–based studies, 8 , 9 the CBT follows the corticospinal tract to the brainstem where multiple CBT branches connect with the cranial motor nuclei (CMN). In contrast to brainstem mapping, which maps the CMN and the peripheral CNs, CBT-MEP monitors the entire CN motor pathway from its origin at the cerebral cortex all the way to the targeted muscle. It is a modification from transcranial MEP monitoring of the corticospinal tract using the same method, electrodes, and stimulation sites, while the target muscles correspond to brainstem monitoring. The orbicularis oculi muscle, however, is not suitable for CBT-MEP because it is too closely situated to the stimulation site. The main methodologies for CBT-MEP monitoring and standard MEP monitoring do not differ. During brain-stem resection, transcranial stimulation is performed every 1 to 2 minutes with a train of stimuli. Therefore, the required electrodes are placed at C3 and C4 (international 10–20 EEG system). The surgeon uses CBT-MEP to obtain online feedback about the preservation of the motor function of CMNs without the need to interrupt the surgical flow. However, limb muscle MEPs should be monitored in addition to CBT-MEPs, because CBT and the corticospinal tract can be damaged separately.
It is possible that motor CNs are activated directly during CBTMEP monitoring if transcranial stimulation is performed with high-current intensities. This might lead to false-positive results and must be avoided. EMG responses following a train of stimuli combined with silence after a single stimulus is a strong indication that the EMG responses are conducted through the CBT. 10
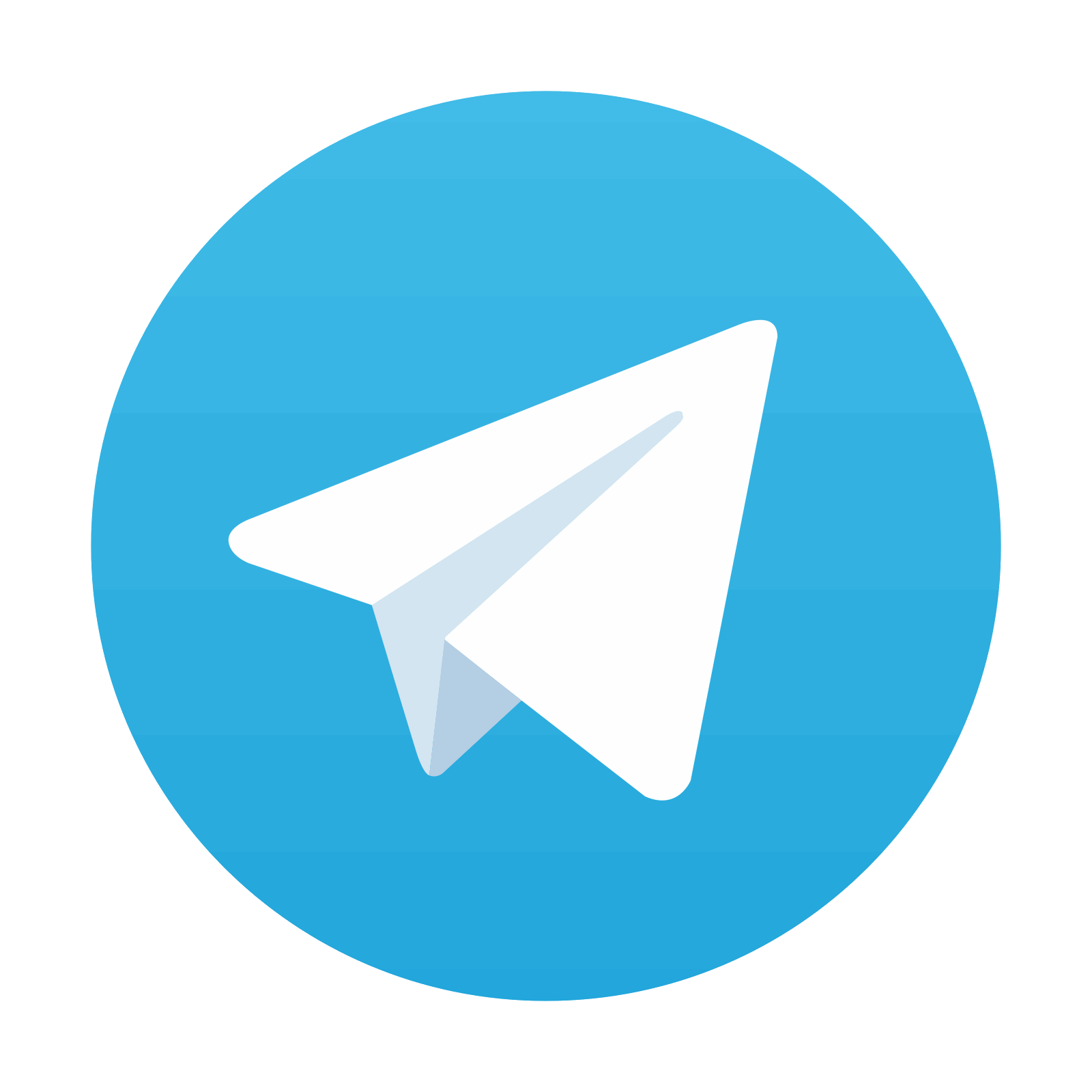
Stay updated, free articles. Join our Telegram channel

Full access? Get Clinical Tree
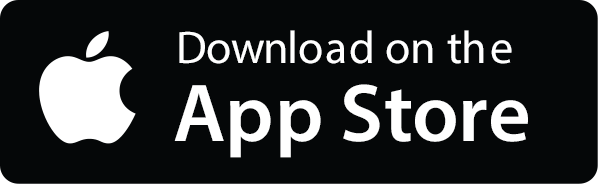
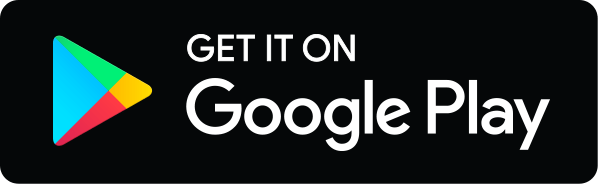
