6 Trauma, Tumor, and Infection
6.1 Introduction
This chapter focuses predominantly on trauma. Tumor 1 , 2 and infection, 3 – 6 however, often have biomechanical effects on the spine that are similar to those of trauma. Tumor- and infection-related fractures, dislocations (translational deformities), and rotational injuries can occur, particularly when tumor or infection is associated with superimposed trauma—however slight or seemingly insignificant. For this reason, tumor, infection, and trauma are discussed together as one entity, with trauma presented as the focus of discussion.
The most common and illustrative types of spine injury are presented to describe the associated mechanisms of injury and pathologic anatomy. The radiographic diagnosis of instability and pathologic anatomy relies heavily on plain radiography (anteroposterior and lateral radiographs). Computed tomography (CT), however, plays a significant role, particularly when dorsal bony element disruption is in question. 7 Magnetic resonance (MR) imaging may be particularly useful in identifying soft tissue injury and neural element compromise (see Chapter 3).
6.2 Loss of Structural Integrity of the Upper Cervical Spine
The upper cervical spine is prone to traumatically induced injuries because of (1) the unique anatomy of that region, (2) the substantial spinal movements allowed, and (3) the high incidence of exposure of the upper cervical spine to significant pathologic stresses from trauma (usually in association with head trauma). The unique anatomy and mobility of this region of the spine are addressed in Chapter 1 . The importance of the third factor, the high incidence of exposure to significant pathologic stresses, is enhanced by the substantial forces often applied during head trauma.
Previously reported observations indicate that most upper cervical spine injuries result from blows to the head. 8 – 15 Another noteworthy cause of such injuries is sudden deceleration of the torso, combined with restriction of movement of the cervical spine. This creates a flexion–distraction mechanism of injury that results from an applied bending moment. 8 Spinal involvement with tumor or infection obviously lowers the tolerance for such injuries.
Very violent movements of the head can disrupt strong protective ligaments of the upper cervical spine. The kinetic energy absorbed by the calvaria in these cases may be sufficient to cause death by head injury. The spine injury incurred, however, may also be fatal. 9
6.2.1 Relevant Anatomy
The C1 vertebra is essentially a ring with an interconnecting and transecting transverse ligament (transverse ligament of the atlas) and articulating facets (both rostral to the occiput and caudal to the axis) on both sides. A multitude of ligaments secure fixation to the surrounding vertebral and cranial bony elements. These ligaments may fail under an excessive load. The orientations of the articulating facets make the ring of C1 prone to injury from axial loading, and the location of the dorsal arch of C1 makes it prone to hyperextension and hyperflexion loading injuries (Fig. 6.1).

The pedicles of C2 are located more ventrally and medially than those at other spinal levels. They essentially form a dorsolateral extension of the vertebral body, connecting the vertebral body proper with its superior articulating process (lateral mass). The pars interarticularis of C2 has a more rostrocaudal orientation. This affects the way in which loads are transmitted through the occiput–C1–C2 complex and the type of injuries sustained when loading to failure occurs; when an axial load is borne, the lateral masses accept the load. These anatomical variables also affect the C2 screw placement decision-making process (Fig. 6.2).

6.2.2 Factors Determining Type of Injury
The orientation of the force vector applied to the cervical spine is the predominant factor dictating the type of injury. The applied-force vector must also result from a deceleration of the torso. The relative intrinsic strengths of C1 and C2, as well as the surrounding spinal elements (including the adjacent vertebrae, calvaria, and supporting ligaments), secondarily dictate the type of injury by “setting the stage” for dissipating the energy of the applied-force vector. 16 , 17 The kinetic energy imparted predominantly dictates the magnitude of the injury. 18
The “stage-setting” aspect of the relative intrinsic strengths of the spinal elements is particularly obvious when more than one injury can theoretically result from the application of a single force vector. For example, an axially applied load can result in a burst fracture of the atlas, a C2 burst-pedicle fracture, or a subaxial cervical spine burst fracture. The relative intrinsic strengths of the ring of C1, the body and pedicles of C2, and the subaxial cervical spine vertebral bodies dictate the type of injury incurred if a failure-producing force is, indeed, applied. Usually, the ring of C1 or a subaxial cervical spine vertebral body is the weakest link, and a C1 burst fracture (Jefferson fracture; see Fig. 6.1) or a subaxial cervical spine fracture is incurred. Occasionally, however, C2 is the weakest link (see the following).
6.2.3 Applied-Force Vectors
In most cases, the kinetic energy imparted to the upper cervical spine is directed to this region through the odontoid process via the ventral arch of C1 or the transverse ligament of the atlas, unless a true axial load is applied (Fig. 6.3). The direction (orientation) of the applied-force vector essentially dictates the location of the fault line (location of the fracture site or ligamentous disruption) (see Fig. 6.4). 17 The location of the fault line is also influenced by the intrinsic strengths and weaknesses of C1 and C2 and the surrounding bony and soft tissue elements.


Although most failure-producing forces applied to the upper cervical spine are applied via the odontoid process, a true axial load injury, in which the superior articulating processes (lateral masses) of C1 and C2 accept all of the load applied to the upper cervical spine, is an exception. If structural failure of the upper cervical spine occurs following the application of an axial load, a bursting of C1 (or less frequently a bursting of C2 or an occipital condyle fracture) may occur. 17 , 19
6.2.4 Types and Mechanisms of Injury
The definition of mechanism of injury in upper cervical spine fractures and dislocations has been complicated by the assumption that the injury types must be relatively few in number. The variety of observed mechanisms of injury, however, causes confusion. Fig. 6.5 illustrates the force vectors that cause the various C1 and C2 fractures and dislocations. Several of the injury types that result from these force vectors have been underrecognized. Their structural characteristics, and what is known about their respective mechanisms of injury, are discussed individually.

The injury types are discussed in the order of force vector application, beginning with the judicial hangman’s fracture and proceeding in a clockwise manner (Fig. 6.5a). Then, lateral (coronal) injuries (Fig. 6.5b) and finally rotatory injuries are discussed.
The schema presented here is subject to change with the advent of new clinical and biomechanical observations.
Judicial Hangman’s Fracture
The combination of distraction and capital hyperextension results from judicial hanging with the noose placed in the submental position (see injury mechanism A, Fig. 6.5a). Falls with a rostrally oriented force vector applied to the submental position can occasionally cause the same injury. 20 , 21 The evolution of judicial hanging is associated with a relatively rich history and scientific study. Such was eloquently recorded by Rayes et al. 22
Dorsal Dislocation of C1 on C2
Dorsal C1–C2 dislocations (Fig. 6.6) are rare. 23 They are purported to be caused by injury mechanism A (see Fig. 6.5a). This results in the ventral arch of C1 riding over the dens, with the result that it becomes “locked” behind the dens.

Traumatic Spondylolisthesis of the Axis (Hangman’s Fracture)
The sudden hyperextension of the head, without an associated distraction component, causes the commonly observed hangman’s fracture (traumatic spondylolisthesis of the axis) (see injury mechanism B, Fig. 6.5a). 11 These fractures have been classified by Effendi and colleagues. 11 They are often associated with a C2–C3 dislocation (subluxation). The extent of both the bony (pars interarticularis) and soft tissue (C2–C3 disc) injury determines the Effendi grade (Fig. 6.7), as well as the extent of stability disruption and the need for surgery. Injuries without significant displacement (< 6 mm) can be managed with a cervical collar. Displacement may require a halo brace (see Chapter 22). Rarely is surgery necessary, although controversy exists. 24 Severe dislocations (Effendi type III) are such cases. 9 , 25

Vertical Coronally Oriented Dorsal C2 Body Fracture with C2–C3 Extension–Subluxation (Type I C2 Body Fracture with C2–C3 Extension–Subluxation)
A slightly smaller capital extension, combined with a small axial load component (see injury mechanism C, Fig. 6.5a), may result in an injury slightly different from traumatic spondylolisthesis of the axis. In this case, the bony fault travels through the dorsal C2 vertebral body instead of the pars interarticularis of C2, which is typical for traumatic spondylolisthesis of the axis as defined by Burke and Harris 10 and Effendi and colleagues. 11 However, it is not atypical and is not a spondylolisthesis of the axis (hangman’s fracture). It has an appearance similar to that of the type I C2 body fracture (Fig. 6.4).
Vertical Coronally Oriented Dorsal C2 Body Fracture with C2–C3 Extension–Subluxation and Ventral Teardrop (Type I C2 Body Fracture with C2–C3 Extension–Subluxation and Ventral Teardrop) 8 , 26
A force vector applied to the high forehead region may result in the application of an axial load and capital hyperextension forces to the upper cervical spine (see injury mechanism D, Fig. 6.5a). The direction and magnitude of the force applied result in disruption of the disc interspace and hypertension of the spine at the C2–C3 level. This causes an opening of the ventral disc interspace and a teardrop avulsion fracture of the ventral caudal aspect of the C2 vertebral body. The vertically oriented axial load causes significant compression of the C2–C3 disc interspace, with a shearing mechanism applied to the ventral and dorsal aspects of the vertebral body because of the variable resistance encountered (the perimeter of the disc interspace is more rigid). This is termed a dorsal C2 body fracture and subluxation with a ventral teardrop (type I C2 body fracture). It resembles other type I C2 body fractures, except for the addition of the ventral teardrop component (see Fig. 6.3).
Type I C2 Body Fracture Variants: Horizontal Caudal C2 Body Fractures
Type I C2 body fracture variants include the isolated extension teardrop and hyperextension dislocation injuries described by Burke and Harris (see injury mechanisms C and D, Fig. 6.5 a). 10 With these fracture variants, the vertical coronal dorsal C2 body component is not observed. Therefore, the whole C2 body extends, instead of the aspect of the vertebral body that is ventral to the fault, as in the formal type II C2 body fracture. This extension results in C2–C3 disc interspace disruption (with the hypertension dislocation injury) and a teardrop or avulsion injury to the ventral caudal aspect of the C2 vertebral body (Fig. 6.8). These variants are more common than other C2 body fractures. 10 They are termed horizontal caudal C2 body fractures.

C1 Burst Fracture (Jefferson Fracture)
Axial loads applied to the vertex of the calvaria (see injury mechanism E, Fig. 6.5a) can cause several types of injury. The most common of these is the C1 burst fracture (Jefferson fracture; see Fig. 6.1). The bursting of the C1 ring occurs because of the radically oriented (lateral) resultant forces applied by the condyles of the occiput and facet joints of C2. Their oblique orientation causes the laterally directed resultant force. This causes a fracture about the ring of C1, usually in four locations. If the total lateral displacement of the C1 facet joints on C2 exceeds 7 mm (adding together the lateral displacements of the right and left sides), the fracture may be unstable. This is because of the rupture of the transverse ligament of the atlas (Fig. 6.9). This rule of thumb can be misleading. Computed tomography (CT) and MR imaging can be helpful regarding the definition of an unstable injury. A type I transverse ligament of the axis injury is purely ligamentous. A type II injury is an avulsion-type injury. The latter usually heals by fracture healing. The former does not and may require surgery. 27 It is emphasized that the mechanism of injury is controversial. 28

Horizontal C1 Fractures
A horizontal split of the ventral C1 arch is often associated with other ligamentous injuries, and therefore its presence should suggest a careful work-up to identify associated injuries. The injury results from violent distraction and extension. The longus colli muscle attaches to the anterior tubercle of C1 and likely applies the force that results in the avulsion or separation between the rostral and caudal portions of the ventral C1 ring (Fig. 6.10). 29

Occipital Condyle Fracture
There are three types of occipital condyle fractures. 7 , 30 – 33 Types I and II usually are incurred via an axially applied load (see injury mechanism E, Fig. 6.5a; see also Fig. 6.11). A type I fracture is a medial disruption of the condyle (impacted occipital condyle) caused by a resultant force that is applied medially on account of the oblique orientation of the occipital condyle–C1 facet (Fig. 6.11a). This is relatively uncommon, most likely because the C1 arch is usually a weaker link and so tends to fail first (Jefferson fracture). The type II occipital condyle fracture (an extension of a basilar skull fracture) most likely also results from an axially applied load (Fig. 6.11b). 30 , 33 The management of occipital condyle fractures is complex. Decision making, therefore, must be based on data and logic. 34

Vertical Sagittally Oriented C2 Burst-Pedicle Fracture (Type II C2 Body Fracture)
Axial loads applied to the vertex of the skull (see injury mechanism E, Fig. 6.5a, b) may occasionally cause a C2 body fracture. 35 If other spinal elements do not fail first (resulting in an occipital condyle fracture, a Jefferson fracture, or a subaxial cervical spine burst fracture), the load applied to the articular pillars of C2 may result in a comminuted sagittal fracture of the C2 body. This injury is best visualized via an anteroposterior view (Fig. 6.12). With this fracture, the C2 body fails along its lateral aspect, in the region of the pedicle–vertebral body junction. Because part of the dorsal wall of the C2 vertebral body is thrust into the spinal canal by virtue of the predominantly axial load applied, the fracture is, by the definition of Denis et al, 36 a burst fracture (see the following).

The C1 burst fracture (Jefferson fracture) and the type I and type II occipital fractures are caused by the same mechanism of injury. If the C1 ring and the occipital condyles are strong, the better part of an applied axial load is accepted by C2. If not, the mechanism of injury may result in a Jefferson fracture or, less commonly, an occipital condyle fracture. With purely axial loads, most of the load is borne by the facet joints; with the addition of hyperextension or flexion components, a large portion of the load is borne by the odontoid process. In the case of nonaxially applied loads, the dens functions as a lever or moment arm by accepting the load and applying a bending moment. Only when isolated axial loads are applied to the C2 vertebra does the dens not function as a lever arm. The addition of a flexion or extension component results in the stressing of a substantially weaker link (see Fig. 6.3).
The addition of a lateral component to the axial load may shift the location of the fracture laterally (see Fig. 6.5a, b), causing a more laterally situated sagittal C2 fracture. 17 , 35 This fracture may pass through the foramina transversaria and along the pars interarticularis of C2.
C1 Arch Fracture
Axial loads, with or without a hyperextension component (see injury mechanisms C, D, and E, Fig. 6.5a), may result in a fracture through the weakest point of the ring of C1. This weak point is near the course of the vertebral artery; its fracture is commonly associated with other upper cervical spine injuries (e.g., hangman’s fracture). Hyperflexion may result in the same injury, via the ligamentous attachments to C1 (see Fig. 6.1).
Vertical Coronally Oriented Dorsal C2 Body Teardrop Fracture with C2–C3 Flexion–Subluxation (Type I C2 Body Fracture with Flexion–Subluxation)
A dorsally applied force vector with an axial load component (see injury mechanism F, Fig. 6.5a) may result in the opening of the dorsal aspect of the C2–C3 disc interspace (capital neck flexion), thus causing an accompanying avulsion teardrop fracture of the dorsal aspect of the caudal C2 vertebral body. Because the C2–C3 disc interspace is slanted in a downward direction, its orientation is nearly in line with the applied-force vector. This, then, results in a subluxation between C2 and C3. This fracture resembles other type I C2 body fractures (see Fig. 6.3). Occasionally, the fracture may extend rostrally into the dens, or a vertical dens fracture can occur in isolation. 37 The latter may be caused by the ventral foramen magnum impinging on the dens as a result of an applied axial load.
Horizontal Rostral C2 Body Fracture (Type III C2 Body Fracture)
A dorsal blow to the head (see injury mechanism G, Fig. 6.5a) may result in true neck flexion. Previously reported data 14 demonstrate that if the C2 region fails, a horizontal fracture occurs through the rostral portion of the body of C2. This has been termed a type III odontoid process fracture. 38 However, this fracture is, by the definition of Anderson and D’Alonzo, through the region of the C2 body, not the odontoid process. 17 , 33 Therefore, it should be considered not an odontoid fracture, but rather a C2 body fracture (horizontal rostral C2 body fracture, type III C2 body fracture). For completeness, the Anderson and D’Alonzo scheme is depicted in Fig. 6.13. The horizontal rostral C2 body fracture (type III Anderson and D’Alonzo fracture) is depicted in Fig. 6.12c.

C2 fractures in children are uncommon. They tend to occur through ossification centers (synchondroses) and result from a dorsal blow to the head (see injury mechanism G, Fig. 6.5a). The synchondroses are depicted in Fig. 6.14a, and a CT scan and the relevant embrylogic anatomy of such a fracture are shown in Fig. 6.14b, e. Of note is that the embryonic dens encompasses much of what is considered the body of C2 in adults.

Rupture of the Transverse Ligament of the Atlas
If the odontoid process does not yield to a failure-producing force applied by injury mechanism G (see Fig. 6.5a), the transverse ligament of the atlas may rupture (Fig. 6.15). 14 , 15 The ligament is usually a stronger link than the rostral portion of the C2 vertebral body; hence, there is lesser incidence of this injury. MR imaging may be used to demonstrate disruption of the transverse ligament of the atlas. 39 Vertical mobile atlantoaxial dislocations have been described. 40 Ligamentous laxity is most certainly a predisposing factor because overt trauma does not appear to be requisite.

Vertical Coronally Oriented Dorsal C2 Body Fracture with Flexion–Distraction (Type I C2 Body Fracture with Flexion–Distraction)
If a capital flexion injury is combined with a distraction component, which is usually caused by deceleration over a fulcrum (e.g., the shoulder harness of an automobile), a flexion–distraction force complex is applied (see injury mechanism H, Fig. 6.5a). This results in a bending moment about the ventral caudal aspect of C2, an opening of the disc interspace dorsally, maintenance or exaggeration of the disc height, and preservation of the ventral soft tissue integrity (evidenced by the lack of demonstration of ventral soft tissue injuries on MR imaging). This is termed a vertical coronally oriented dorsal C2 body fracture with flexion–distraction (type I C2 body fracture with flexion–distraction). Its radiographic appearance is similar to those of other type I C2 body fractures (see Fig. 6.4). It has also been termed an atypical hangman’s fracture. 41
Comment: Vertical coronally oriented C2 body fractures have multiple mechanistic causes. These include hyperextension with an axial load, hyperflexion with an axial load, and flexion–distraction (Fig. 6.16). The complex etiology of C2 body fractures is the source of the confusion surrounding this aspect of spinal trauma.

Dens Fracture
The type II odontoid process fracture of Anderson and D’Alonzo 38 (see Fig. 6.13) may be more appropriately termed a dens fracture. 2 It most probably results from a lateral blow to the head (see injury mechanism I, Fig. 6.5b), 31 possibly coupled with extension. 32
Dens fractures have been further subcategorized by Korres et al, based on the level and characteristics of the fracture. 42 Most feel it is unnecessary to employ such a scheme clinically because clinical relevance has yet to be shown. Nonunion is common with type II fractures. A nonunion may evolve into an os odontoideum. 43 Advancing age portends an increasingly poor prognosis regarding fracture healing and the development of medical complications associated with dens fractures, particularly after surgical intervention 44 —hence the currently increased enthusiasm for nonoperative management in octogenarians 45 in selected cases. 46
Occipital Condyle Fracture (Type III)
A lateral blow to the head (see injury mechanism I, Fig. 6.5b) uncommonly results in a medial avulsion of the occipital condyle. This is termed a type III occipital condyle fracture. 30 It is an avulsion injury caused by shearing forces that put tension on the occipital condyle via the alar and capsular ligaments (see Fig. 6.11C). 30 , 33 It may be associated with open upper cervical injuries. 47 Tectorial membrane injury is a key feature, particularly in children. 2
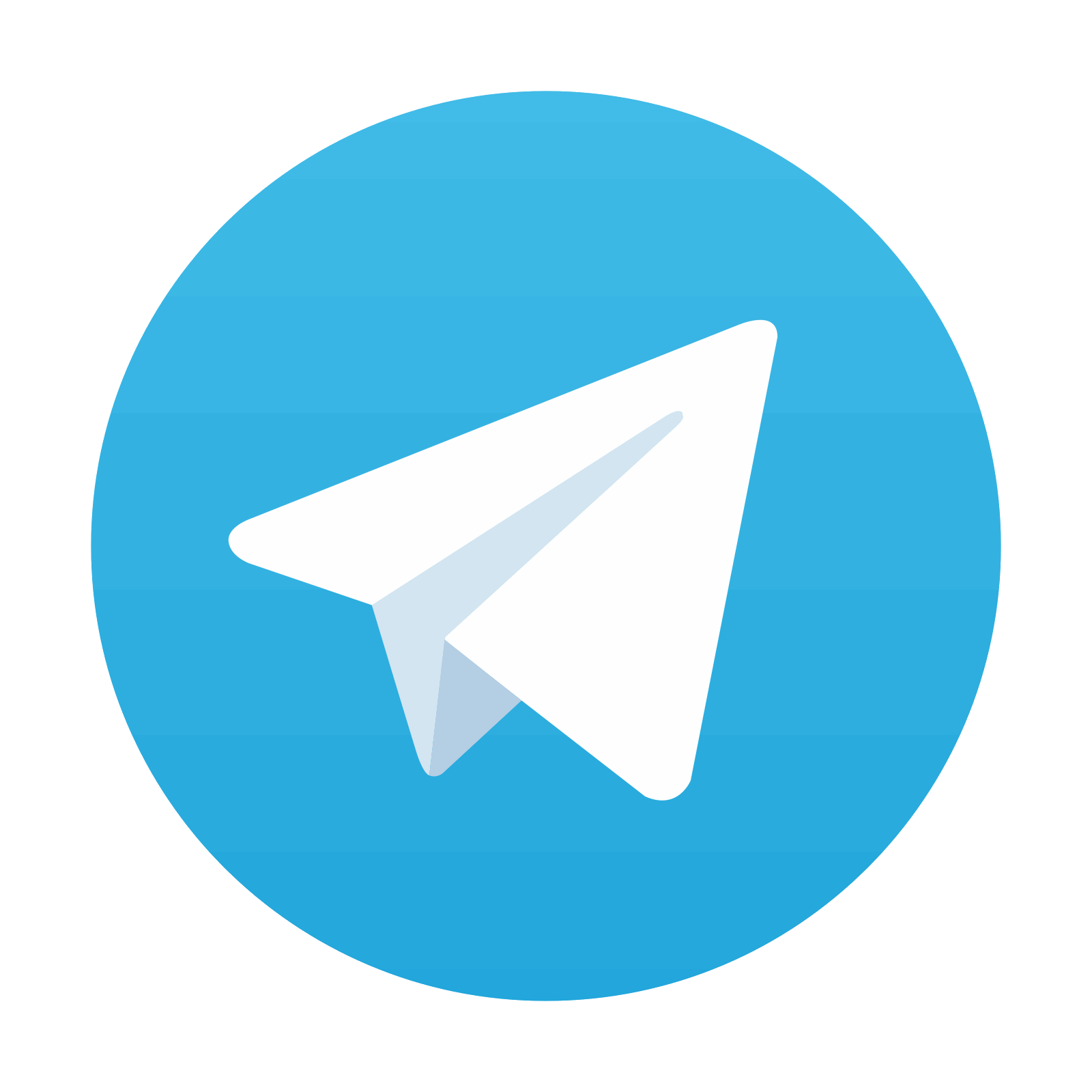
Stay updated, free articles. Join our Telegram channel

Full access? Get Clinical Tree
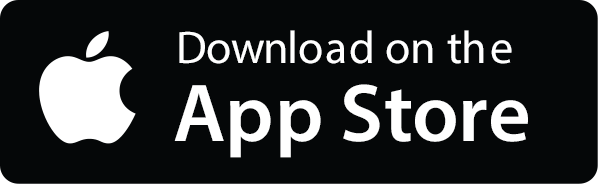
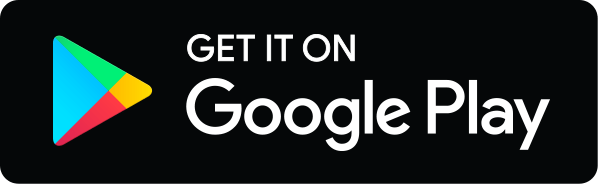
